ABSTRACT
mRNA is an attractive drug target for therapeutic interventions. In this review we highlight the current state, clinical trials, and developments in antisense therapy, including the classical approaches like RNaseH-dependent oligomers, splice-switching oligomers, aptamers, and therapeutic RNA interference. Furthermore, we provide an overview on emerging concepts for using RNA in therapeutic settings including protein replacement by in-vitro-transcribed mRNAs, mRNA as vaccines and anti-allergic drugs. Finally, we give a brief outlook on early-stage RNA repair approaches that apply endogenous or engineered proteins in combination with short RNAs or chemically stabilized oligomers for the re-programming of point mutations, RNA modifications, and frame shift mutations directly on the endogenous mRNA.
Abbreviations
ASO | = | Antisense oligonucleotide |
CD | = | Cluster of differentiation |
CFTR | = | Cystic fibrosis transmembrane conductance regulator |
CRISPR/Cas9 | = | Clustered regularly interspaced short palindromic repeats/CRISPR-associated 9 |
FDA | = | US Food and Drug Administration |
GalNAc | = | N-acetyl galactosamine |
IVT-mRNA | = | In-vitro transcribed mRNA |
MHC | = | Major histocompatibility complex |
miRNA | = | microRNA |
MOE | = | 2´-O-methoxyethyl |
mRNA | = | messenger RNA |
ψ | = | pseudouridine |
PS | = | Phosphothioate |
RNAi | = | RNA interference |
siRNA | = | Short interfering RNA |
SSO | = | Splice-switching oligonucleotide |
SMN2 | = | Survival of motor neuron 2 |
TALEN | = | Transcription activator-like effector nuclease |
TLR | = | Toll-like receptor |
TH1/2 cell | = | Type 1/2 T helper cell |
TR1 | = | Type 1 regulatory T cell |
VEGF | = | Vascular endothelial growth factor |
VEGFR-1 | = | Vascular endothelial growth factor receptor 1 |
ZFN | = | Zinc finger nuclease |
Introduction
During the last 15 y the diverse roles of RNA in regular but also pathological cellular processes became increasingly clear. RNA is not only a short-lived messenger and part of the translational machinery but RNA contributes significantly to the regulation and diversification of the genetic information. There is now increasing insight into the mechanistic role of defective RNA processing, including (alternative) splicing, modification, translation, and decay for the etiology of various diseases.Citation1-4 However, not only mis-regulation and defective processing cause disease, but even RNA species themselves can initiate disease processes independent of their protein-coding function. Nucleotide repeat diseases are typical examples.Citation5 To employ this new mechanistic knowledge and to translate it into therapy requires drugs that reliably target nucleic acids in a sequence-specific manner. However, there are only few small molecule drugs that target nucleic acids and those are limited in their capacity of sequence addressing. In contrast, oligonucleotide analogs provide a basis for the rational design of highly sequence-specific drugs to target virtually any cellular nucleic acid in a specific manner.Citation6 Classical drugs like small molecules target enzymes and receptors to block or alter their specific functions. In contrast, the interference at the nucleic acid level would allow to manipulate the transcriptome and the proteome itself. This is not limited to the simple up- or down-regulation of target gene expression. Most appealing is the possibility of actively creating new transcript and protein isoforms with altered properties and functions, for instance by re-programming a protein-coding stretch, or by altering splice sites, modification patterns, polyadenylation states, miRNA binding sites, etc.Citation7 Affecting the cell by targeting its nucleic acids clearly enlarges the scope of currently available therapeutic interventions including the causal treatment of some genetic diseases.
However, already short oligonucleotides have unfavorable pharmacological properties. They are hydrophilic, polyanionic macromolecules that can hardly overcome cellular membranes, are unstable against RNases, and suffer from rapid renal clearance.Citation8 This leads to short half-life and low bioavailability. Furthermore, adverse toxic effects may appear that include immune-reactions and off-target binding to non-targeted cellular nucleic acids. Together, oligonucleotide drugs are often characterized by low efficacy and high toxicity which strongly limits their clinical application.Citation6 During the last decades, medicinal chemists have put enormous effort into the development of new chemistries that improve lifetime, delivery, potency, and efficacy of the drugs while reducing their toxicity and immunogenicity. These new chemistries are now approaching clinical trials and will hopefully pave the way for the broad clinical application of oligonucleotide drugs. An overview on recent developments in oligonucleotide medicinal chemistry can be found elsewhere.Citation6,7
In principle, interference with the genetic information could be achieved permanently at the DNA- or transiently at the RNA-level. In this review we will focus on the RNA-level. Even though novel approaches for genome engineering are currently keenly explored,Citation9 we believe that it would be foolish to carelessly discard the RNA alternative. With respect to ethical issues and safety aspects, the transient and thus reversible nature of RNA manipulation could turn out as a blessing in disguise. Both, the therapeutic effects and the potential adverse effects, are likely to be tunable. Furthermore, manipulations are conceivable that are inaccessible or difficult to realize on the genome level per se. This includes amino acid changes or transcript level changes that would kill a cell if they are permanently enforced. Potentially lethal interventions on kinases, apoptosis factors, transcription or translation factors could be realized on the RNA-level suddenly, transiently or partially to obtain a therapeutic effect, for instance. Manipulation at the RNA-level might also be much more efficient compared to HDR-dependent genomic knock-in, which remained persistently inefficient in vivo, in particular in postmitotic tissues like the brain.Citation9 For many genetic diseases, which are caused by loss-of-function mutations, a patient would benefit more from a drug that can restore a small fraction (like 5%) of functional gene product in a large fraction of a the tissue than from a drug that can restore full gene function (100%) but only in a small fraction of the tissue. A typical example is cystic fibrosis.Citation10
In this review we will first update on recent developments in the classical approaches, like RNaseH-dependent decay, chemically stabilized oligonucleotides that target mRNAs to induce splice-switching, aptamers, and the knock-down via RNAi (). After painful years of repeated relapse one seems to have learned the lessons and have now substantially improved the effectiveness of such drugs. For instance, in 2015 therapeutic RNAi was demonstrated in a relevant monkey model by subcutaneous administration of a chemically stabilized siRNA that partially knocks down antithrombin in the monkey´s liver.Citation11 The problem of delivery and toxicity seems to be solved, at least for simple oligonucleotide drugs and for some organs, and allows therapeutic intervention with an affordable amount of the drug under compliant administration routes. Consequently, the number of promising clinical phase II and III studies has increased during the last few years (see ).
Figure 1. Chemically stabilized, short oligonucleotides can employ various mechanisms for their therapeutic effects ranging from blocking ligand – receptor binding, RNA degradation via RISC or RNaseH(1) recruitment, and alteration of splicing. The classical modes of action are shown on the left panel, a small section of typically used chemical backbone modifications are depicted on the right.
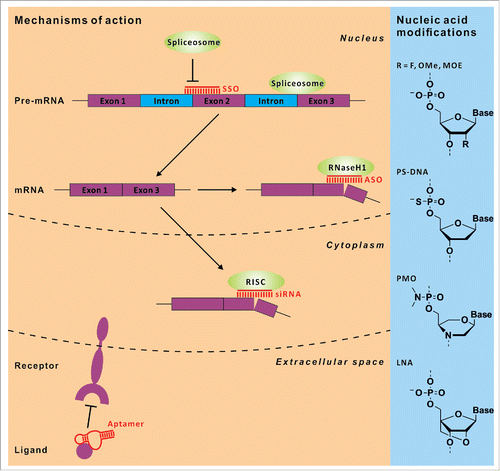
Table 1. Overview on the most recent and advanced clinical trials in the corresponding fields not claiming completeness. For RNAi, failed early trials are also listed that have been terminated in 2009 or before.
Every new discovery in RNA function and regulation offers a starting point to develop novel therapies. After its discovery in 1998 we now find numerous drug candidates in clinical studies that apply the RNAi mechanism ().Citation12 In the second part of this review we highlight emerging concepts that are still in the pre-clinical or very early clinical exploration stage but that have the potential to become medicines of the future. This includes therapeutic mRNAs, mRNAs as vaccine, and RNA repair approaches. The latter apply endogenous or engineered enzymes to repair, re-program, or modify a target RNA at a specific site in order to provoke a therapeutically relevant effect ().
Figure 2. Overview on selected enzymatic processes that could be harnessed to restore gene function by repairing or re-programming mRNA site-specifically. Site-directed A-to-I editing, 2´-O-methylation, pseudouridylation, and frameshift correction via expression or administration of short guideRNAs has already been demonstrated. Many other processes are conceivable and currently under exploration.
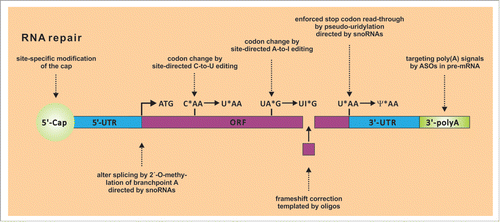
Update on established approaches
RNaseH-dependent antisense oligonucleotides
Oligonucleotides working through an RNaseH-dependent cleavage mechanism are the oldest class of antisense oligonucleotides (ASO). They are extensively explored and represent the largest class of nucleic acid analog drugs in clinical trials. RNaseH-dependent ASOs are short DNA oligomers targeting mRNA. Once the DNA-oligo/mRNA heteroduplex is formed, human RNaseH1 binds to it and catalyzes RNA cleavage under release of the intact DNA oligomer.Citation13
Medicinal chemists have undertaken great efforts to improve ASO design regarding nuclease resistance, circulation half-life, target affinity (potency), and tissue specificity. The first ASOs tested in clinical trials, also referred to as 1st generation ASOs, have been modified by oxygen-to-sulfur substitutions in the phosphate backbone. ASOs with such a phosphothioate (PS) backbone show enhanced nuclease resistance and prolonged plasma half-life due to non-specific binding to plasma proteins preventing them from rapid renal filtration. However, numerous toxicities were also associated with that type of modification.Citation6 In 1998, fomivirsen was the first FDA-approved ASO and was applied for the treatment of human cytomegalovirus-induced retinitis in HIV patients.Citation14-16 Marketed as Vitravene, the 21 nt PS-oligonucleotide was administered by intravitreal injection to target the immediate early region 2 of the viral mRNA. Since the approval of fomivirsen, several ASOs belonging to the 1st generation are under clinical review. For instance, targeting the mRNA of intercellular adhesion molecule 1 and the insulin receptor substrate 1 are advanced in the treatment of pouchitisCitation17,18 and vascular disorders in the eye,Citation19-22 respectively. The RNaseH-mediated degradation of Akt-1 mRNA to impede tumor proliferationCitation23 is currently tested for clinical application.Citation24-26
Due to the early success with 1st generation ASO, further medicinal chemistry was explored to improve half-life and potency of the drugs in order to reduce the administered dose, the application frequency, the costs, and to minimize adverse effects.Citation27 This resulted in the 2nd generation ASOs, also referred to as gapmers. A typical gapmer is a 20 nt oligonucleotide comprising a PS backbone and 5 flanking 2′O-methoxyethyl (MOE) groups at both termini. Due to the unmodified internal DNA gap, such ASOs remain good substrates for RNaseH, whereas the terminal MOE modifications increase nuclease resistance and enhances the binding of the ASO to the target mRNA.Citation28 2nd generation ASOs entered clinical trials for various therapeutic applications. The most prominent representative of the 2nd generation is the MOE gapmer mipomersen as the second FDA-approved RNaseH-dependent ASO. The compound targets apolipoprotein B-100 mRNA and is subcutaneously administered to treat familiar hypercholesterolemia. The genetic disorder is caused by the loss of low-density lipoprotein (LDL) receptor function leading to high LDL cholesterol plasma concentration and early cardiovascular disease. Phase III trials had demonstrated an efficient decrease of LDL cholesterol by lowering ApoB-100 amount in patients obtaining mipomersen.Citation29-31 The treatment obviously profited from the general pharmacokinetics of systemically administered ASOs which preferably accumulate in the liver where ApoB-100 synthesis takes place.Citation8 Recently, an RNase-dependent ASOCitation32 has reached clinical phase III to reduce transthyretin expression in patients suffering from familial amyloid polyneuropathy.Citation33-35 Chemotherapy combined with RNaseH-mediated degradation of clusterin mRNA is a potential therapeutic option in the treatment of prostateCitation36-38 and lung cancer.Citation39,40
Generation 2.5 ASO are derived from the traditional gapmer design. For this, the MOE modifications are replaced by 2′,4′-constrained ethyl (cEt) bridges in the flanking nucleotides. It was found that cEt-modified oligonucleotides provide the same superior target affinity, but increased nuclease resistance as compared to locked nucleic acid (LNA)-containing oligonucleotides.Citation41 One of the generation 2.5 ASOs targets the mRNA of signal transducer and activator of transcription 3Citation42 and is currently tested for the treatment of various cancer types.Citation43-46
Most recently, a new chemistry has been developed that strongly increases the liver-specific uptake of oligonucleotide drugs, including ASO and siRNA therapeutics. For this, ASOsCitation47 and siRNAsCitation48 are conjugated with triantennary N-acetyl galactosamine (GalNAc3). GalNAc3 mediates liver-specific uptake through the asialoglycoprotein receptor (ASGPR) that is exclusively expressed on hepatocytes. Marketed as ligand-conjugated antisense (LICA) technology (Ionis Pharmaceuticals), it could be shown that the conjugation increases the potency of MOE gapmers up to 10-fold for inhibiting the expression of hepatic genes in mice.Citation49 When using a GalNAc3-conjugated cEt gapmer, the RNaseH-mediated mRNA degradation was enhanced around 60-fold as compared to the corresponding 2nd generation MOE ASO. Additionally, Ionis Pharmaceuticals announced that its LICA drug targeting apolipoprotein(a) was 30-fold more potent in a phase I study than the unconjugated MOE gapmer.Citation50,51
Splice-switching oligonucleotides
Pre-mRNA is matured during a complex nuclear process called splicing that removes the introns (non-coding sequences) and joins the exons (coding sequences). By applying alternative splice sites and by occasional inclusion or exclusion of exons and introns, multiple protein variants are derived from one gene (alternative splicing). Several diseases are related to aberrant RNA-splicing leading to non-functional proteins, and great efforts have been undertaken to develop antisense oligonucleotides, referred to as splice-switching oligonucleotides (SSOs) that manipulate splicing. Therapeutic SSOs promoting exon skipping and exon retention for the treatment for Duchenne muscular dystrophy (DMD) and spinal muscular atrophy (SMA) are currently evaluated in clinical trials.Citation7
Dystrophin, the protein encoded by the DMD gene, is crucial for the integrity of muscle tissue.Citation52 In rare cases, newborn males harbor a defect dystrophin gene on their X chromosome. The patients suffer from successive muscle wasting resulting in a premature death due to respiratory or cardiac failure. In most cases, the loss-of-protein-function results from exonic out-of-frame deletions. In many cases the reading frame can be restored by skipping the aberrant exon by addressing a SSO to an internal exonic splicing enhancer.Citation53 The resulting truncated dystrophin protein retains partial function and gives the less severe Becker muscular dystrophy phenotype.Citation54 Several SSOs have been developed that are clinically evaluated for the skipping of exons 44, 45, 51, and 53, including drisapersen and eteplirsen (). Recently, both companies submitted new drug applications for their lead compounds drisapersenCitation55-57 and eteplirsen,Citation58,59, both amenable to exon 51 skipping. In case of drisapersen, the FDA rejected the application due to major concerns about the efficacy and safety of the drug.Citation60 The high dosage required led to severe adverse effects including renal and vascular injury. To improve efficacy and safety other SSO chemistries might be more successful. Whereas drisapersen is a 20 nt 2′O-methoxy phosphorothioate RNA analog, eteplirsen is a 30 nt phosphorodiamidate oligomer, a so-called morpholino. The final decision on the efficacy and safety evaluation by the FDA is still pending for eteplirsen. Additionally, a new, morpholino-based SSO for exon 53 skipping is currently under clinical evaluation (NS-065/NCNP-01).Citation61,62 For the future, we can hope in new chemistries. A SSO that relies on 2′O,4′C-ethylene-bridged nucleosides (ENA oligonucleotides)Citation63 which mediate nuclease resistance and improved binding affinity to RNA has now entered a clinical phase I/II trial for the treatment of DMD (DS-4151b).Citation64,65
Spinal muscular atrophy (SMA) is a rare genetic disorder caused by survival of motor neuron 1 (SMN1) gene mutations.Citation66 Infant patients affected by this disease suffer from the loss of motor neurons and associated muscle wasting. However, there is a therapeutic approach by activating the SMN2 gene, which is almost identical to SMN1, but a single mutation in a splicing enhancer strongly prevents the inclusion of exon 7 resulting in an unstable protein unable to replace the lost SMN1 function.Citation67 In a mouse model, a highly potent 2′O-methoxyethyl PS SSO for exon 7 retention in SMN2 was identified (IONIS-SMNRx).Citation68 The drug is injected in the spinal cord ensuring the direct delivery to the affected motor neurons without the need to cross the blood-brain barrier. After promising clinical phase II results regarding efficacy and safety of the drug candidate,Citation69 two phase III trials were recently initiated for evaluating IONIS-SMNRx.Citation70,71
Although the SSO design remains challenging, several new therapeutic applications were successfully validated in preclinical studies.Citation72 Possible drug approvals of eteplirsen or IONIS-SMNRx in the near future could eventually proof the feasibility of the splice-modulating antisense oligonucleotide approach.
Aptamers
Aptamers are 20 – 100 nt long oligomers that adopt complex three dimensional structures that allow them to interact potently and specifically with various proteins typically achieving nM- to pM binding affinities.Citation73 They are readily obtained in an iterative laboratory evolution procedure called SELEX (systematic evolution of ligands by exponential enrichment).Citation74 Currently, aptamers are mainly targeting extracellular structures such as plasma proteins and cell surface receptors thus avoiding the problem of intracellular delivery. Hence, aptamers are comparable in many aspects to antibodies, however, aptamers are much smaller, can penetrate tissues deeper, are chemically synthesized to highest purity and homogeneity and differ in their toxicity and immunogenicity profile. To improve their plasma life-time and to adjust their toxicity, aptamers are typically chemically stabilized (2′-OMe, 2′-F, 3′ inverted dT) and PEGylated.
In 2004, the first (and until today the only) aptamer, Macugen, was approved by the FDA for clinical therapy of AMD (age-related macular degeneration). The 27-nt chemically stabilized RNA oligomer is directed against the vascular endothelial growth factor (isoform 165) and blocks VEGF-receptor-induced neovascularization.Citation75,76 After achieving its highest sales in 2010, it has now almost entirely been displaced by antibodies (Ranibizumab and Bevacizumab, for instance) which can bind additional VEGF isoforms besides VEGF-165 and thus benefit for their poorer specificity compared to the aptamer. After this early breakthrough with Macugen, numerous aptamers have been explored in clinical settings. However, some programs suffered very unfortunate setbacks at late clinical trial states, like the aptamer-containing anticoagulation system REG1 which was terminated in 2014 in a phase III study due to unexpected toxicity / immunogenicity issues ().Citation77,78
Currently, several aptamers for the local treatment of eye diseases are in late clinic trials (II and III), for instance the aptamers FovistaCitation79-81 and Zimura,Citation82,83 which target PDFG (it is a growth factor) and C5, respectively. In combination with VEGF inhibitors they might find application in the treatment of AMD in the near future. To overcome the prevalent problems with toxicity and immunogenicity, NOXXON Pharma develops so-called Spiegelmer therapeutics.Citation84 These drugs apply stereochemically inverted nucleotides based on l-ribose instead of the natural d-ribose, can be evolved via SELEX, and are suggested to be resistant against nucleasesCitation85 and invisible for the immune system.Citation86 Currently, 3 Spiegelmer aptamersCitation86-92 are in clinical phase II studies ().
Therapeutic RNAi
RNA interference (RNAi) is a mechanism of posttranscriptional gene regulation that was discovered in 1998.Citation12 RNAi can interfere with gene expression in various ways including the degradation of a specific mRNA target via endonucleolytic cleavage, or via recruitment of deadenylation / decapping enzymes, but it can also positively affect the stability and translation of a specific mRNA. The mechanistic details that lead to the respective responses are still under exploration. In principle, a dsRNA that is introduced into the cytoplasm is processed by the RNase dicer into ∼22 bp RNA duplexes and loaded onto the endonuclease Argonaut-2 (Ago-2). Ago-2 slices the passenger strand of the RNA-duplex and applies the remaining guide strand for sequence-specific mRNA-targeting.Citation93 While short interfering RNAs (siRNAs) are fully complementary to their target mRNA and promote cleavage (knock-down), micro RNAs (miRNAs) contain bulges and loops that prohibit slicing by Ago-2, but alter the stability and translational activity of the target.Citation94
Allowing the selective knock-down of genes in cell culture and animal-models, RNAi quickly became a valuable tool in basic biology.Citation95-97 In parallel a race started to exploit the RNAi mechanism for therapeutic purposes and several big pharma companies, like Merck, Roche, and Pfizer made large investments that resulted in the first clinical trials in 2004, already 6 y after the discovery of RNAi.Citation98,99 However, in the aftermath those early trials mostly failed due to strong innate immune reactions and/or lack of patients´ benefit, and in the consequence big pharma left RNAi again.Citation100-104 In the 18 y since its discovery the field of therapeutic RNAi went from enthusiastic interest over despondence and back again, resulting in a re-assessment of the technological obstacles and more realistic expectations for clinical trials. This has been accompanied by commentary elsewhere.Citation105,106
However, after recent successes in clinical trials, showing the efficacy of RNAi therapeutics to reduce transthyretinCitation107 and PCSK9Citation108 in patients, the interest in RNAi is currently growing and even big pharma including Sanofi and Roche started to invest again.Citation98 The initial drawbacks in clinical trials were mostly related to the low efficacy of the drugs, off-target issues and immune-related toxicity.Citation109 Off-target effects include immune-reactions induced by the siRNA/miRNA precursors, and up- and downregulation of non-target mRNAs due to saturation of the RNAi machinery and off-target binding of the siRNA.Citation110 There is now increasing success in tackling all those issues. Current innovations include chemical modification / sequence optimization of siRNAs and its precursors, and new solutions to the delivery problem. The latter include various forms of (lipid) nanoparticles and bioconjugates. The details of this progress are comprehensively reviewed elsewhere.Citation110-113 Briefly, clinical trials seem more successful when they are confined to readily accessible organs like the liver, cancer, and immune-privileged areas like the eye.Citation114-122 Whereas the eye is a good target for naked siRNAs, treatment of the liver benefited from lipid-based nanoparticles and the above-mentioned GalNAc3 conjugates.Citation116 In particular the GalNAc3 approach has significantly improved the efficacy of siRNA-conjugates, allowing now the weekly administration of liver-targeting siRNA via subcutaneous injection in non-human primates to knock-down antithrombin to clinically relevant levels.Citation11 Notable in this approach is that it allows to knockdown an essential protein (like antithrombin) in a tunable and reversible manner, whereas the permanent knock-out of antithrombin (for instance at the DNA-level) is lethal.Citation11 Overall, more than 20 siRNA drugs in various formulations are in clinical trials now (up to phase III, ).Citation123 RNAi-therapy clearly has the potential to tackle currently undruggable diseases and to appear in the clinics soon.
The therapeutic use of the miRNA-related mechanism (not applying the slicing activity of Ago2) is still in its infancy. Attractive is the possibility of manipulating larger networks of genes simultaneously in both, a negative and positive manner.Citation124 This might become interesting for the treatment of complex diseases like cancer. On the other hand, endogenous miRNAs are involved in many cellular processes and their manipulation could also be disease-relevant. The knockdown of miRNA 122 with antisense oligonucleotides was shown to interfere with hepatitis C virus progression and is currently in phase II clinical studies.Citation125 As the hepatitis virus seems to require the endogenous miRNA for its functioning the knockdown of this host-specific factor is particularly promising as the virus cannot adapt easily by evolution.Citation126 Other miRNAs that are linked to cancer like miRNA 16 and 34a are also targeted with ASOs and are currently in clinical trials phase I.Citation127,128
Emerging concepts for therapy
Therapeutic mRNA
For a long time it has been believed that only short, chemically stabilized oligonucleotides are suitable as drugs. However, long (protein-encoding) mRNAs haven recently proven their enormous therapeutic potential. Protein replacement experiments were first performed in the early 1990ties with naked mRNA in mice and rats.Citation129,130 Even though replacement experiments were successful to some degree, there have been massive problems related to the well-known RNA-dependent immune-stimulation through interferon-I (IFN-I) and a generally low translation efficiency.Citation131,132
However, during the last 15 years, our mechanistic understanding of the immune-stimulatory effect of RNA has substantially improved. This was due to the discovery of RNA sensors including the Toll-like receptors (TLR) 3, 7, 8, Melanoma differentiation-associated protein 5 (MDA-5), Retinoic acid inducible gene I (RIG-I), as well as various RNA helicases.Citation133 Besides the activation of the innate immune response under release of the respective signaling molecules we have also learned how these RNA-sensing events are directly linked to the general repression of mRNA translation in the affected cells. Among others, general translation repression is mediated by phosphorylation of translation initiation factor 2α via protein kinase R activation.Citation134,135 In the worst case, IFN-I activates 2′–5′-adenylate synthase and RNaseL and leads to apoptosis.Citation136
RNA replacement strategies aim to achieve high translation levels under minimal immune stimulation. Both can be achieved by designing mRNAs that evade RNA-sensing. The following strategies turned out as particularly successful.
Chemically modified pyrimide nucleotides like pseudouridine (ψ), 2-thiouridine (s2U), and 5-methylcytidine (m5C) are incorporated into mRNAs during in-vitro-transcription to minimize recognition by RNA sensors.Citation137 Substitution of uridine by pseudouridine was shown to diminish recognition by TLR-3, -7, -8, and RIG-I.Citation137,138 To fine-tune effects on translation efficiency, nucleotide analogs are often mixed with their natural counterparts. The extent to which these modifications may induce mistranslation is yet unknown.Citation139
Rigorous purification of the mRNA product from unincorporated nucleoside triphosphates, small abortive transcripts, remaining DNA templates, and in particular dsRNA via HPLC (High performance liquid chromatography) was shown to dramatically reduce immunogenicity of the transcripts and can increase the translation 10- to 1000-fold.Citation140,141
Synthetic cap analog structures like ARCA (anti-reverse-cap-analog) can further decrease immune response and improve translation. In contrast to older cap analogs, ARCA is always incorporated in correct orientation.Citation142,143 A new ARCA variant contains a phosphothioate that resists enzymatic decapping and can increase the half-life of the mRNA.Citation144
Computational sequence design allows to reduce the number of particularly immune-stimulatory nucleotides and combinations (like UW, with W = A or U). Citation145-147 Furthermore, transcript stability can be optimized by the introduction of 3′-UTRs (or some elements) taken from other mammalian or viral genes as well as addition of Poly(A)-tails.Citation148-153
The RNA replacement strategy is particularly advantageous when a transient, burst-like expression of a protein is desired. Typical examples for the latter are the epigenetic re-programming (induced pluripotency), wound healing, and genome editing. In this sense, in-vitro transcribed mRNA (IVT-mRNAs) has been used to deliver a) human bone morphogenetic protein 2 (hBMP-2) to support bone regeneration in rats; to deliver b) the transcription factor mix that induces pluripotency; and to deliver c) vascular endothelial growth factor-A (VEGF-A) into a mouse model for myocardial infarction resulting in an improved heart function and enhanced survival.Citation154-160 Furthermore, IVT-mRNAs have been successful in the delivery of surfactant protein B in deficient mice, and in the delivery of murine erythropoietin to increase the hematocrit.Citation138,161
IVT-mRNA could turn out as a valuable tool for genome editing. Genome editing holds great promise for the treatment of various diseases by a permanent repair of a gene via a site-directed knock-in or knockout.Citation162 However, the respective nucleases that induce the required double-strand DNA breaks including ZFNs, Talens, and CRISPR/Cas, should not be persistently expressed as this would dramatically increase the chance of off-target genome editing.Citation9 Consequently, its delivery as an mRNA is beneficial compared to a DNA vector and also circumvents the typical safety risks of viral and non-viral DNA-based methods like genomic insertion and antivector immunogenicity. Encoding of genome editing tools via IVT-mRNAs has already been widely used to generate transgenic animals.Citation163-169 In a proof-of-concept study, gene function was restored via homology-directed promotor exchange in a surfactant-B-deficient mouse model by in-vivo-delivery of the ZFN in form of an IVT-mRNA. However, this required the additional delivery of the repair template (with the promotor) in form of an AAV6 (Adeno-associated-virus serotype 6).Citation170 Successful promoter exchange was demonstrated and resulted in a prolonged life of the treated mice. IVT-mRNA encoded Talen have been used successfully to disrupt the CCR5 (CC chemokine receptor type 5) gene via non-homologous-end-joining in the T-cell line PM1. As the loss of CCR5 function confers resistance toward R5-tropic HIV-1 infection, side-directed nucleases are promising to target this infectious disease.Citation171 An initial clinical phase I study is currently starting.Citation172 As IVT-mRNA is a young field, this study represents the first clinical study that uses IVT-mRNAs, but more are likely to follow soon.
mRNA can have many advantages over DNA vectors to deliver therapeutic proteins. Besides its transient nature, we want note that mRNA is very well and quickly translated in postmitotic cells that are difficult to transfect with DNA vectors. mRNA also works independent of a promotor, but this can potentially limit its application if tissue-specificity is required. However, we know from various studies that there is a large number of regulatory elements, typically in the 3´-UTR, including miRNA binding sites, stabilizing and destabilizing elements that could allow to manipulate the expression of an IVT-mRNA in a tissue-specific manner in the future.Citation94,173
Oligonucleotides for vaccination and desensitization
As indicated above, very successful strategies haven't been developed to evade the RNA-sensing event and to trick the innate immune system. However, inducing a specific immune response can be highly desired. Thus the recent knowledge on the immune stimulation by RNA can be used for the latter. Currently, the classical vaccination is based on the delivery of inactivated or living viruses, virus-like particles, or antigenic peptides. While the antigenic peptides require additional vaccination adjuvants like alum salts, the other entities contain sufficient pathogen-associated-molecular-patterns (PAMPs) in form of proteins, nucleic-acids, and lipopolysaccharides. These PAMPs are detected by pattern-recognition-receptors (including the above-mentioned RNA sensors) and induce the release of type-I interferons, pro-inflammatory cytokines, and chemokines. This is reviewed in-depth elsewhere.Citation174,175 Short peptide fragments are then presented to the immune system via MHC-complexes on dendritic cells and other antigen presenting cells.Citation176 This process finally induces a humoral as well as cellular immune response of the adaptive immune system.
The presented antigens are mainly protein-derived peptides. This opens the intriguing possibility to deliver antigens for MHC-presentation encoded as IVT-mRNAs under simultaneous induction of the necessary innate and adaptive immune stimulation as the IVT-mRNA itself can function as PAMP. By doing so, it is well conceivable to create specific immune responses not only against viruses and bacteria, but also against cancer cells or for allergy treatment.Citation177-181 The design of such mRNA-based vaccines would be highly rational, fast, cheap, and could be done in a personalized manner, for instance against the specific transcriptome of a patient-specific cancer.Citation182 IVT-mRNA vaccines would be faster available as the generation of virus-particles (and similar entities) would be circumvented. Lyophilized mRNA vaccines can be stored at 37 °C for several weeks.Citation183 This allows the transport of vaccines into regions that cannot provide an uninterrupted cold chain. The safety-profile could also be better compared to DNA-based methods (insertion mutagenesis, low efficiency) or virus-like entities (therapy-induced virus-specific humoral immune response).Citation184-186 Again, also for vaccination, the transient nature of RNA expression is beneficial, as a low-level, long-term expression of an antigen might induce tolerance.Citation187
Two major IVT-mRNA-based vaccination strategies are currently explored: the ex-vivo and the in-vivo approach. The first, which was earlier developed, is based on the ex-vivo pulsing of allogenic (= patient-derived) dendritic cells with antigen-encoding mRNA, which allows the redirection of the adaptive immune system to target cancer or virus-infected cells. The feasibility and safety of this method was proven in pre- and clinical trials focused on HIV and various cancer types. However, personalized ex-vivo therapies require time-consuming and expensive individualized manufacturing processes which currently limit their broad clinical application.Citation188-195 Nevertheless, further clinical trials up to phase III are currently running.Citation196-202
Even though cumbersome, the ex-vivo strategy allows to optimize and control mRNA transfection and immune stimulation more carefully. The in-vivo approach, however, is potentially more simple and elegant, but encounters additional problems. Whereas all IVT-mRNA strategies require stable and highly translatable transcripts, the in-vivo strategy requires additionally the immune-stimulatory effect that counteracts translation. It was found that complexation of IVT-mRNA with protamine enhances immunogenicity via TLR-7 activation and simultaneously improves stability, however, with the downside of low antigen expression.Citation203 Anyway, a combination of protamine-complexed IVT-mRNA together with naked IVT-mRNA of the same sequence turned out to satisfy both needs at the same time: high translation efficiency and immune stimulation. Those self-adjuvanting mRNAs are currently in phase I and II clinical trials against prostate cancer, late stage lung cancer, and rabies; pre-clinical trials against influenza have been performed.Citation183,186,204-209 We wish to mention that also other approaches that apply naked or formulated IVT-mRNAs are in clinical trials, for instance for targeting other cancer entities.Citation172,210 Furthermore, non-coding RNA can also be used as a vaccination adjuvant replacing the classical alum salts as adjuvant of protein- or peptide-based vaccines.Citation211
Currently, IVT-mRNA are expensive therapies. On one hand, the GMP (Good manufacturing practice) production of IVT-mRNA in large scale is not yet fully established, but CureVac has announced significant progress here.Citation212 On the other hand the potency of IVT-mRNA could be further improved by assisted delivery via lipid-nanoparticels, polymeric nanoparticles, gold nanoparticles, among others, as reviewed elsewhere.Citation213 Furthermore, there are promising attempts to develop self-replicating RNA-vaccines that apply viral RNA-dependent RNA-polymerases (from α-virus) to produce the RNA vaccine from a dilute IVT-mRNA template.Citation214-216 However, there are safety concerns related to the control of the replication process and the tolerance against the viral RNA-polymerase, but the strategy is still in the pre-clinical exploration phase.Citation217
Finally, mRNA vaccines could also be used in allergy treatment to desensitize the immune system against a specific antigen. Desensitization against type-I allergies is typically accomplished through repeated intra-dermal, intra-nodal, or sub-lingual application of allergens. Whereas a strong Immunglobuline E and CD8+ T-cell responses is intended during vaccination, desensitization aims to change the TH1/TR1 to TH2 cell ratio toward TH1/TR1 to fine-tune the immune response and to induce tolerance.Citation178 Application of low-dose IVT-mRNA could be used for that purpose, and there is pre-clinical data that prove efficacy and suggest a long-term protective effect.Citation218 One can expect first clinical trials to start within the next few years. Applying mRNA as an anti-allergic vaccine has several advantages compared to the classical allergen extract (like standardized cat extract) or DNA-based vaccines.Citation219,220 IVT-mRNA is obtained in a defined and highly pure state thus avoiding unintended antigens that can be included in allergen extracts.Citation221,222 DNA-based allergy treatment on the other hand suffers from the above mentioned safety concerns and thus harbors disproportional risk in the context of a preventative therapy.
RNA repair
Besides the manipulation of splicing, most interventions on the RNA-level aim to destroy or block their endogenous targets. Strategies to restore the function of an RNA that is corrupted by missense, nonsense or frameshift mutation, or by defective processing are rare. In case of loss-of-function mutations, the administration of a therapeutic mRNA to replace the non-functional variant might solve the problem, as discussed above. However, this is only feasible with a small number of therapeutic mRNAs that can be translated under low control of translation level and tissues specificity. Indeed, many transcripts are tightly regulated with respect to their dose and tissue specificity and come as a mixture of various isoforms due to alternative promotor usage, alternative splicing, alternative polyadenylation and alternative posttranscriptional modification. Such transcript variants may differ in their function, localization, stability, etc. To address this variety in an mRNA replacement strategy seems impractical. A better alternative would be the repair of the endogenously expressed but defective RNA transcript, a strategy, we call RNA repair.
Very recently, we and others have engineered artificial RNA-guided editing machineries that allow to re-program genetic information at the RNA level.Citation223-225 For this, adenosine-to-inosine (A-to-I) RNA editing enzymesCitation226,227 are directed toward specific sites on selected transcripts and allow for the precise posttranscriptional manipulation of the genetic information. The manipulation results from the fact that inosine is biochemically interpreted as guanosine. Thus, formal A-to-G conversions become accessible, in a highly site-specific manner. The specificity comes from the guideRNA that addresses the editing enzymes and can be readily programmed in rational way, simply by applying Watson-Crick pairing rules.Citation228 Even though only A-to-G mutations are accessible the scope of manipulations is large. Twelve out of the 20 canonical amino acids can be manipulated, comprising almost all of the polar ones which are essential for protein function.Citation223 Furthermore, START and STOP codon, splice elements, polyadenylation signals, and viral RNA are potential targets.Citation226,227 We and others have shown that such strategies work inside mammalian cell cultureCitation229 and even in a simple organismCitation230 and allow the repair of disease-relevant genes, like the CFTR mRNA.Citation225
Other people have recently shown the possibility of re-directing snoRNA-guided RNA modification machineries, like the 2´-O-methylationCitation231 and the pseudouridylation machinery.Citation139 The first modification allows interference with splicing, the second allows the read-through of premature STOP codons. Mammalian cells harbor a plethora of RNA modifying and processing enzymes. There is no need to restrict ourselves to the usage of nucleases, like RISC, RNaseH, and RNaseP.Citation232 Just to give a few examples, there are RNA editing and modifying enzymes inside the cell that can change nucleotides (A-to-I, C-to-U233, U-to-ψ, A-to-m6A,Citation234 and many more for the tRNAsCitation235), that add the capCitation236 and the poly(A)-tail,Citation237 RNAs can be precisely processed, for instance by the CCA-adding enzymes,Citation238,239 TUTases,Citation240 etc.Citation241 Thus, even complex repair processes are conceivable, including the repair of insertion and deletion mutations at the RNA-level. In this respect, we want to recall a largely overseen work from 2004, done by Paul Zamecnik, the pioneer of antisense therapy, in his early nineties shortly before he passed away. He demonstrated the possibility of repairing the terrible Δ508 deletion mutation in the CFTR gene, the main cause of cystic fibrosis, simply by administration of 2 chemically stabilized RNA oligomers.Citation242 In cell culture, the efficiency of mRNA repair was sufficient to restore the chloride channel function. Unfortunately, he was unable to elucidate the mechanism, but he could clearly demonstrate the repair to take place at the mRNA. Such a complex repair requires a concerted nuclease, ligase (and polymerase) activity at a specific site on an mRNA molecule. In summary, it seems that numerous endogenous enzymes stand ready inside the cell for RNA repair processes. We just have to learn how to make use of them.Citation243,244 If successful, one can establish novel platforms for therapeutic intervention.
Conclusions
While splice-switching oligomers and aptamers are still struggling on their ways to the clinic, major progress has been made for RNaseH-dependent ASOs and for therapeutic RNAi with chemically stabilized siRNAs. This is due to the development of new chemistries that improve efficacy and delivery of the drugs to some specific organs. An impressive example is the development of the GalNAc3 conjugation that clearly improves liver targeting and might allow for the administration of siRNA and ASO by subcutaneous administration in the future. However, overcoming problems with delivery and efficacy remains elusive for many organs and will require massive basic research in the future.
Among the emerging approaches, the usage of in-vitro-transcribed mRNA for protein replacement and vaccination has made impressive progress. This was mainly due to the tailored suppression or harnessing of the RNA-induced immune response by chemical modification and formulation. The approach has the potential to find wide application in the clinics whenever a transient, burst-like expression is advantageous. The RNA repair approach is still in its infancy, but we believe that the harnessing of artificial and in particular endogenous RNA repair proteins might enable new therapies, complementing the above-mentioned classical RNA-based and the approaching genome editing methods, and being superior to the latter with respect to safety and ethical issues.
Overall, the progress during last years is impressive. The increasing number of clinical trials for various approaches makes us feel optimistic that numerous nucleic-acid-based drugs will soon find their ways to the patients to enable novel therapies.
Disclosure of potential conflicts of interest
No potential conflicts of interest were disclosed.
Funding
We gratefully acknowledge support from the University of Tübingen and the Deutsche Forschungsgemeinschaft (STA 1053/3–2, STA 1053/4–1). This work has received funding from the European Research Council (ERC) under the European Union's Horizon 2020 research and innovation program (grant agreement No 647328, RNArepair).
References
- Klungland A, Dahl JA. Dynamic RNA modifications in disease. Curr Opin Genet Dev 2014; 26:47-52; PMID:25005745; http://dx.doi.org/10.1016/j.gde.2014.05.006
- Schoenberg DR, Maquat LE. Regulation of cytoplasmic mRNA decay. Nat Rev Genet 2012; 13:246-59; PMID:22392217; http://dx.doi.org/10.1038/nrg3254
- Chabot B, Shkreta L. Defective control of pre-messenger RNA splicing in human disease. J Cell Biol 2016; 212:13-27; PMID:26728853; http://dx.doi.org/10.1083/jcb.201510032
- Scheper GC, van der Knaap MS, Proud CG. Translation matters: protein synthesis defects in inherited disease. Nat Rev Genet 2007; 8:711-23; PMID:17680008; http://dx.doi.org/10.1038/nrg2142
- Nalavade R, Griesche N, Ryan DP, Hildebrand S, Krauss S. Mechanisms of RNA-induced toxicity in CAG repeat disorders. Cell Death Dis 2013; 4:e752; PMID:23907466; http://dx.doi.org/10.1038/cddis.2013.276
- Bennett CF, Swayze EE. RNA targeting therapeutics: molecular mechanisms of antisense oligonucleotides as a therapeutic platform. Annu Rev Pharmacol Toxicol 2010; 50:259-93; PMID:20055705; http://dx.doi.org/10.1146/annurev.pharmtox.010909.105654
- Kole R, Krainer AR, Altman S. RNA therapeutics: beyond RNA interference and antisense oligonucleotides. Nat Rev Drug Discov 2012; 11:125-40; PMID:22262036; http:dx.doi.org/10.1038/nrd3625
- Geary RS. Antisense oligonucleotide pharmacokinetics and metabolism. Expert Opin Drug Metab Toxicol 2009; 5:381-91; PMID:19379126; http://dx.doi.org/10.1517/17425250902877680
- Cox DB, Platt RJ, Zhang F. Therapeutic genome editing: prospects and challenges. Nat Med 2015; 21:121-31; PMID:25654603; http://dx.doi.org/10.1038/nm.3793
- Myra Stern and Eric WFW Alton. Use of Liposomes in the Treatment of Cystic Fibrosis. Gene Therapy in Lung Disease CRC Press, 2002; 383–396; http://dx.doi.org/10.3109/9780203908822-18
- Sehgal A, Barros S, Ivanciu L, Cooley B, Qin J, Racie T, Hettinger J, Carioto M, Jiang Y, Brodsky J, et al. An RNAi therapeutic targeting antithrombin to rebalance the coagulation system and promote hemostasis in hemophilia. Nat Med 2015; 21:492-7; PMID:25849132; http://dx.doi.org/10.1038/nm.3847
- Fire A, Xu S, Montgomery MK, Kostas SA, Driver SE, Mello CC. Potent and specific genetic interference by double-stranded RNA in Caenorhabditis elegans. Nature 1998; 391:806-11; PMID:9486653; http://dx.doi.org/10.1038/35888
- Wu H, Lima WF, Zhang H, Fan A, Sun H, Crooke ST. Determination of the role of the human RNase H1 in the pharmacology of DNA-like antisense drugs. J Biol Chem 2004; 279:17181-9; PMID:14960586; http://dx.doi.org/10.1074/jbc.M311683200
- Vitravene Study Group. A randomized controlled clinical trial of intravitreous fomivirsen for treatment of newly diagnosed peripheral cytomegalovirus retinitis in patients with AIDS. Am J Ophthalmol 2002; 133:467-74; PMID:11931780; http://dx.doi.org/10.1016/S0002-9394(02)01327-2
- Vitravene Study Group. Randomized dose-comparison studies of intravitreous fomivirsen for treatment of cytomegalovirus retinitis that has reactivated or is persistently active despite other therapies in patients with AIDS. Am J Ophthalmol 2002; 133:475-83; PMID:11931781; http://dx.doi.org/10.1016/S0002-9394(02)01326-0
- Vitravene Study Group. Safety of intravitreous fomivirsen for treatment of cytomegalovirus retinitis in patients with AIDS. Am J Ophthalmol 2002; 133:484-98; PMID:11931782; http://dx.doi.org/10.1016/S0002-9394(02)01332-6
- Greuter T, Biedermann L, Rogler G, Sauter B, Seibold F. Alicaforsen, an antisense inhibitor of ICAM-1, as treatment for chronic refractory pouchitis after proctocolectomy: A case series. United European Gastroenterol J 2016; 4:97-104; PMID:26966529; http://dx.doi.org/10.1177/2050640615593681
- Randomized Study of Topical Alicaforsen Enema in Antibiotic Refractory Pouchitis. (2015). Clinical Phase Trial III
- Cloutier F, Lawrence M, Goody R, Lamoureux S, Al-Mahmood S, Colin S, Ferry A, Conduzorgues JP, Hadri A, Cursiefen C, et al. Antiangiogenic activity of aganirsen in nonhuman primate and rodent models of retinal neovascular disease after topical administration. Invest Ophthalmol Vis Sci 2012; 53:1195-203; PMID:22323484; http://dx.doi.org/10.1167/iovs.11-9064
- Cursiefen C, Viaud E, Bock F, Geudelin B, Ferry A, Kadlecová P, Lévy M, Al Mahmood S, Colin S, Thorin E, et al. Aganirsen antisense oligonucleotide eye drops inhibit keratitis-induced corneal neovascularization and reduce need for transplantation: the I-CAN study. Ophthalmology 2014; 121:1683-92; PMID:24811963; http://dx.doi.org/10.1016/j.ophtha.2014.03.038
- A multicentre double-blind randomized study to investigate the efficacy and tolerability of GS-101 eye drops, an antisense oligonucleotide, versus placebo on inhibition of corneal neovascularization, a major risk factor of corneal graft rejection: The I-GRAFT study. (2009). Clinical Trial Phase III
- The STRONG Study. (http://strong-nvg.com/the-study/). Clinical Trial Phase II/III
- Yoon H, Kim DJ, Ahn EH, Gellert GC, Shay JW, Ahn CH, Lee YB. Antitumor activity of a novel antisense oligonucleotide against Akt1. J Cell Biochem 2009; 108:832-8; PMID:19693774; http://dx.doi.org/10.1002/jcb.22311
- A Safety and Efficacy Study of RX-0201 Plus Gemcitabine in Metastatic Pancreatic Cancer. (2009). Clinical Trial Phase II
- Dose-Finding, Safety and Efficacy Study of RX-0201 Plus Everolimus in Metastatic Renal Cell Cancer. (2014). Clinical Trial Phase IB/II
- Tagawa ST, Chatta GS, Mazhari R, Benaim E. Archexin, a novel AKT-1-specific inhibitor for the treatment of metastatic renal cancer: Preliminary phase I data. ASCO Annual Meeting Proceedings 2016; (suppl 2S; abstr 550);
- MacLeod AR. Antisense therapies for cancer: bridging the pharmacogenomic divide. Drug Discovery Today: Therapeutic Strategies 2013; 10:e157-e63
- Teplova M, Minasov G, Tereshko V, Inamati GB, Cook PD, Manoharan M, Egli M. Crystal structure and improved antisense properties of 2′-O-(2-methoxyethyl)-RNA. Nat Struct Mol Biol 1999; 6:535-9; PMID:10360355; http://dx.doi.org/10.1038/9304.
- Raal FJ, Santos RD, Blom DJ, Marais AD, Charng M-J, Cromwell WC, Lachmann RH, Gaudet D, Tan JL, Chasan-Taber S, et al. Mipomersen, an apolipoprotein B synthesis inhibitor, for lowering of LDL cholesterol concentrations in patients with homozygous familial hypercholesterolaemia: a randomised, double-blind, placebo-controlled trial. The Lancet 2010; 375:998-1006; http://dx.doi.org/10.1016/S0140-6736(10)60284-X
- Stein EA, Dufour R, Gagne C, Gaudet D, East C, Donovan JM, Chin W, Tribble DL, McGowan M. Apolipoprotein B synthesis inhibition with mipomersen in heterozygous familial hypercholesterolemia: results of a randomized, double-blind, placebo controlled trial to assess efficacy and safety as add-on therapy in patients with coronary artery disease. Circulation 2012; 126:2283-92; PMID:23060426; http://dx.doi.org/10.1161/CIRCULATIONAHA.112.104125
- Santos RD, Duell PB, East C, Guyton JR, Moriarty PM, Chin W, Mittleman RS. Long-term efficacy and safety of mipomersen in patients with familial hypercholesterolaemia: 2-year interim results of an open-label extension. Eur Heart J 2015; 36:566-75; PMID:24366918; http://dx.doi.org/10.1093/eurheartj/eht549
- Ackermann EJ, Guo S, Booten S, Alvarado L, Benson M, Hughes S, Monia BP. Clinical development of an antisense therapy for the treatment of transthyretin-associated polyneuropathy. Amyloid 2012; 19:43-4; PMID:22494066; http://dx.doi.org/10.3109/13506129.2012.673140
- Efficacy and Safety of IONIS-TTR Rx in Familial Amyloid Polyneuropathy. (2012). Clinical Phase trial II/III
- Open-Label Extension Assessing Long Term Safety and Efficacy of IONIS-TTR Rx in Familial Amyloid Polyneuropathy (FAP). (2012). Clinical Phase Trial III
- Ionis Pharmaceuticals Press Release. (http://ir.isispharm.com/phoenix.zhtml?c=222170&p=irol-newsArticle&ID2105651). 2015
- Comparison of Docetaxel/Prednisone to Docetaxel/Prednisone in Combination With OGX-011 in Men With Prostate Cancer (SYNERGY). (2010). Clinical Trial Phase III
- Comparison of Cabazitaxel/Prednisone Alone or in Combination With Custirsen for 2nd Line Chemotherapy in Prostate Cancer (AFFINITY). (2012). Clinical Trial Phase III
- OncoGenex Press Release. (http://ir.oncogenex.com/releasedetail.cfm?ReleaseID=933276). 2015
- Laskin JJ, Nicholas G, Lee C, Gitlitz B, Vincent M, Cormier Y, Stephenson J, Ung Y, Sanborn R, Pressnail B, et al. Phase I/II trial of custirsen (OGX-011), an inhibitor of clusterin, in combination with a gemcitabine and platinum regimen in patients with previously untreated advanced non-small cell lung cancer. J Thorac Oncol 2012; 7:579-86; PMID:22198426; http://dx.doi.org/10.1097/JTO.0b013e31823f459c
- A Multinational, Randomized, Open-Label Study of Custirsen In Patients With Advanced or Metastatic (Stage IV) Non-Small Cell Lung Cancer. (2012). Clinical Trial Phase III
- Seth PP, Vasquez G, Allerson CA, Berdeja A, Gaus H, Kinberger GA, Prakash TP, Migawa MT, Bhat B, Swayze EE. Synthesis and biophysical evaluation of 2′, 4′-constrained 2′ O-methoxyethyl and 2′, 4′-constrained 2′ O-ethyl nucleic acid analogues. J Org Chem 2010; 75:1569-81; PMID:20136157; http://dx.doi.org/10.1021/jo902560f
- Hong D, Kurzrock R, Kim Y, Woessner R, Younes A, Nemunaitis J, Fowler N, Zhou T, Schmidt J, Jo M, et al. AZD9150, a next-generation antisense oligonucleotide inhibitor of STAT3 with early evidence of clinical activity in lymphoma and lung cancer. Sci Translat Med 2015; 7:314ra185-314ra185; http://dx.doi.org/10.1126/scitranslmed.aac5272
- AZD9150, a STAT3 Antisense Oligonucleotide, in People With Malignant Ascites. (2015). Clinical Trial Phase II
- Study to Assess MEDI4736 With Either AZD9150 or AZD5069 in Relapsed Metastatic Squamous Cell Carcinoma of Head & Neck. (2015). Clinical Trial Phase IB/II
- Phase 1/2, Open-label, Dose-escalation Study of IONIS-STAT3Rx, Administered to Patients With Advanced Cancers. (2012). Clinical Trial Phase I/II
- MEDI4736 Alone and in Combination With Tremelimumab or AZD9150 in Adult Subjects With Diffuse Large B-cell Lymphoma (D4190C00023). (2015). Clinical Trial Phase IB
- Prakash PT, Seth PP, Swayze EE, Graham MJ. Compositions and methods for modulating apolipoprotein (a) expression (US. Patent No. Nine,181,550). 2015
- Nair JK, Willoughby JL, Chan A, Charisse K, Alam MR, Wang Q, Hoekstra M, Kandasamy P, Kel'in AV, Milstein S, et al. Multivalent N-acetylgalactosamine-conjugated siRNA localizes in hepatocytes and elicits robust RNAi-mediated gene silencing. J Am Chem Soc 2014; 136:16958-61; PMID:25434769; http://dx.doi.org/10.1021/ja505986a
- Prakash TP, Graham MJ, Yu J, Carty R, Low A, Chappell A, Schmidt K, Zhao C, Aghajan M, Murray HF, et al. Targeted delivery of antisense oligonucleotides to hepatocytes using triantennary N-acetyl galactosamine improves potency 10-fold in mice. Nucleic Acids Res 2014; 42:8796-807; PMID:24992960; http://dx.doi.org/10.1093/nar/gku531
- Ionis Pharmaceuticals Press Release. (http://ir.isispharm.com/phoenix.zhtml?c=222170&p=irol-newsArticle&ID=2110180) 2015
- Safety, Tolerability, Pharmacokinetics, and Pharmacodynamics of IONIS APO(a)-LRx in Healthy Volunteers With Elevated Lipoprotein(a). (2015). Clinical Trial Phase I
- Hoffman EP, Brown RH, Kunkel LM. Dystrophin: the protein product of the Duchenne muscular dystrophy locus. Cell 1987; 51:919-28; PMID:3319190; http://dx.doi.org/10.1016/0092-8674(87)90579-4
- Aartsma‐Rus A, Fokkema I, Verschuuren J, Ginjaar I, van Deutekom J, van Ommen GJ, den Dunnen JT. Theoretic applicability of antisense‐mediated exon skipping for Duchenne muscular dystrophy mutations. Hum Mutat 2009; 30:293-9; PMID:19156838; http://dx.doi.org/10.1002/humu.20918
- Koenig M, Beggs A, Moyer M, Scherpf S, Heindrich K, Bettecken T, Meng G, Müller CR, Lindlöf M, Kaariainen H, et al. The molecular basis for Duchenne versus Becker muscular dystrophy: correlation of severity with type of deletion. Am J Hum Genet 1989; 45:498; PMID:2491009
- Voit T, Topaloglu H, Straub V, Muntoni F, Deconinck N, Campion G, De Kimpe SJ, Eagle M, Guglieri M, Hood S, et al. Safety and efficacy of drisapersen for the treatment of Duchenne muscular dystrophy (DEMAND II): an exploratory, randomised, placebo-controlled phase 2 study. Lancet Neurol 2014; 13:987-96; PMID:25209738; http://dx.doi.org/10.1016/S1474-4422(14)70195-4
- A Study of the Safety, Tolerability & Efficacy of Long-term Administration of Drisapersen in US & Canadian Subjects. (2013). Clinical Trial Phase III
- Extension Study of Drisapersen in DMD Subjects. (2015). Clinical Trial Phase IIIB
- Mendell JR, Rodino‐Klapac LR, Sahenk Z, Roush K, Bird L, Lowes LP, Alfano L, Gomez AM, Lewis S, Kota J, et al. Eteplirsen for the treatment of Duchenne muscular dystrophy. Ann Neurol 2013; 74:637-47; PMID:23907995; http://dx.doi.org/10.1002/ana.23982
- Confirmatory Study of Eteplirsen in DMD Patients (PROMOVI). (2014). Clinical Trial Phase III
- Drisapersen FDA Report. (http://www.fda.gov/downloads/AdvisoryCommittees/CommitteesMeetingMaterials/Drugs/PeripheralandCentralNervousSystemDrugsAdvisoryCommittee/UCM473737.pdf). 2015
- Komaki H, Nagata T, Saito T, Masuda S, Takeshita E, Tachimori H, et al. GP 251-Exon 53 skipping of the dystrophin gene in patients with Duchenne muscular dystrophy by systemic administration of NS-065/NCNP-01: A phase 1, dose escalation, first-in-human study. Neuromuscular Disorders 2015; 25:S261-S2; http://dx.doi.org/10.1016/j.nmd.2015.06.276
- Nippon Shinyaku Press Release. (https://www.nippon-shinyaku.co.jp/english/news/?id=2920). 2016
- Koizumi M. 2′-O, 4′-C-Ethylene-bridged nucleic acids (ENA) as next-generation antisense and antigene agents. Biol Pharm Bull 2004; 27:453-6; PMID:15056846; http://dx.doi.org/10.1248/bpb.27.453
- Daiichi Sankyo Press Release. (http://www.daiichisankyo.com/media_investors/media_relations/press_releases/detail/006412/160225_635_E.pdf). 2016
- Study of DS-5141b in Patients With Duchenne Muscular Dystrophy. (2016). Clinical Trial Phase I/II
- Lefebvre S, Bürglen L, Reboullet S, Clermont O, Burlet P, Viollet L, Benichou B, Cruaud C, Millasseau P, Zeviani M, et al. Identification and characterization of a spinal muscular atrophy-determining gene. Cell 1995; 80:155-65; PMID:7813012; http://dx.doi.org/10.1016/0092-8674(95)90460-3
- Lorson CL, Hahnen E, Androphy EJ, Wirth B. A single nucleotide in the SMN gene regulates splicing and is responsible for spinal muscular atrophy. Proc Natl Acad Sci U S A 1999; 96:6307-11; PMID:103395583; http://dx.doi.org/10.1073/pnas.96.11.6307
- Hua Y, Vickers TA, Okunola HL, Bennett CF, Krainer AR. Antisense masking of an hnRNP A1/A2 intronic splicing silencer corrects SMN2 splicing in transgenic mice. Am J Hum Genet 2008; 82:834-48; PMID:18371932; http://dx.doi.org/10.1016/j.ajhg.2008.01.014
- Ionis Pharmaceuticals. (http://ir.ionispharma.com/phoenix.zhtml?c=222170&p=irol-newsArticle&ID=2097778). 2015
- A Study to Assess the Efficacy and Safety of IONIS-SMN Rx in Infants With Spinal Muscular Atrophy. (2014). Clinical Trial Phase III
- A Study to Assess the Efficacy and Safety of IONIS-SMN Rx in Patients With Later-onset Spinal Muscular Atrophy. (2014). Clinical Phase Trial III
- Disterer P, Kryczka A, Liu Y, Badi YE, Wong JJ, Owen JS, Khoo B. Development of therapeutic splice-switching oligonucleotides. Hum Gene Ther 2014; 25:587-98; PMID:24826963; http://dx.doi.org/10.1089/hum.2013.234
- Keefe AD, Pai S, Ellington A. Aptamers as therapeutics. Nat Rev Drug Discov 2010; 9:537-50; PMID:20592747; http://dx.doi.org/10.1038/nrd3141
- Bouchard PR, Hutabarat RM, Thompson KM. Discovery and Development of Therapeutic Aptamers. Annu Rev Pharmacol Toxicol 2010; 50:237-57; PMID:20055704; http://dx.doi.org/10.1146/annurev.pharmtox.010909.105547
- Ruckman J, Green LS, Beeson J, Waugh S, Gillette WL, Henninger DD, Claesson-Welsh L, Janjić N. 2′-Fluoropyrimidine RNA-based aptamers to the 165-amino acid form of vascular endothelial growth factor (VEGF165). Inhibition of receptor binding and VEGF-induced vascular permeability through interactions requiring the exon 7-encoded domain. J Biol Chem 1998; 273:20556-67; PMID:9685413; http://dx.doi.org/10.1074/jbc.273.32.20556
- Ng EWM, Shima DT, Calias P, Cunningham ET, Guyer DR, Adamis AP. Pegaptanib, a targeted anti-VEGF aptamer for ocular vascular disease. Nat Rev Drug Discov 2006; 5:123-32; PMID:16518379; http://dx.doi.org/10.1038/nrd1955
- Lincoff AM, Mehran R, Povsic TJ, Zelenkofske SL, Huang Z, Armstrong PW, Steg PG, Bode C, Cohen MG, Buller C, et al. Effect of the REG1 anticoagulation system versus bivalirudin on outcomes after percutaneous coronary intervention (REGULATE-PCI): a randomised clinical trial. Lancet 2016; 387:349-56; PMID:26547100; http://dx.doi.org/10.1016/S0140-6736(15)00515-2
- A Study To Determine the Efficacy and Safety of REG1 Compared to Bivalirudin in Patients Undergoing PCI (Regulate). (2013). Clinical Trial Phase III (Terminated)
- Dugel PU. Anti-PDGF combination therapy in neovascular age-related macular degeneration: results of a phase 2b study. Retina Today 2013; 8:65-71
- A Phase 3 Safety and Efficacy Study of Fovista® (E10030) Intravitreous Administration in Combination With Lucentis® Compared to Lucentis® Monotherapy. (2013). Clinical Trial Phase III
- A Phase 3 Safety and Efficacy Study of Fovista® (E10030) Intravitreous Administration in Combination With Either Avastin® or Eylea® Compared to Avastin® or Eylea® Monotherapy. (2013). Clinical Trial Phase III
- Monés J. Complement factor 5 inhibition in age-related macular degeneration. Retina Today 2010; 5:52-5
- A Phase 2/3 Trial to Assess the Safety and Efficacy of Intravitreous Administration of Zimura® (Anti-C5 Aptamer) in Subjects With Geographic Atrophy Secondary to Dry Age-Related Macular Degeneration. (2016). Clinical Trial Phase II/III
- Vater A, Klussmann S. Turning mirror-image oligonucleotides into drugs: the evolution of Spiegelmer® therapeutics. Drug Discovery Today 2015; 20:147-55; PMID:25236655; http://dx.doi.org/10.1016/j.drudis.2014.09.004
- Ashley GW. Modeling, synthesis, and hybridization properties of (L)-ribonucleic acid. J Am Chem Soc 1992; 114:9731-6; http://dx.doi.org/10.1021/ja00051a001
- van Eijk LT, John AS, Schwoebel F, Summo L, Vauléon S, Zöllner S, Laarakkers CM, Kox M, van der Hoeven JG, Swinkels DW, et al. Effect of the antihepcidin Spiegelmer lexaptepid on inflammation-induced decrease in serum iron in humans. Blood 2014; 124:2643-6; PMID:25163699; http://dx.doi.org/10.1182/blood-2014-03-559484
- Roccaro AM, Sacco A, Purschke WG, Moschetta M, Buchner K, Maasch C, Zboralski D, Zöllner S, Vonhoff S, Mishima Y, et al. SDF-1 inhibition targets the bone marrow niche for cancer therapy. Cell Rep 2014; 9:118-28; PMID:25263552; http://dx.doi.org/10.1016/j.celrep.2014.08.042
- NOX-A12 in Combination With Bortezomib and Dexamethasone in Relapsed Multiple Myeloma. (2012). Clinical Trial Phase IIA
- NOX-A12 in Combination With Bendamustine and Rituximab in Relapsed Chronic Lymphocytic Leukemia (CLL). (2011). Clinical Trial Phase IIA
- Oberthür D, Achenbach J, Gabdulkhakov A, Buchner K, Maasch C, Falke S, Rehders D, Klussmann S, Betzel C. Crystal structure of a mirror-image L-RNA aptamer (Spiegelmer) in complex with the natural L-protein target CCL2. Nat Commun 2015; 6:6923; PMID:25901662; http://dx.doi.org/10.1038/ncomms7923
- NOX-E36 in Patients With Type 2 Diabetes Mellitus and Albuminuria. (2012). Clinical Trial Phase IIA
- Efficacy of NOX-H94 on Anemia of Chronic Disease in Patients With Cancer. (2012). Clinical Trial Phase IIA
- MacRae IJ, Ma E, Zhou M, Robinson CV, Doudna JA. In vitro reconstitution of the human RISC-loading complex. Proc Natl Acad Sci U S A 2008; 105:512-7; PMID:18178619; http://dx.doi.org/10.1073/pnas.0710869105
- Valinezhad Orang A, Safaralizadeh R, Kazemzadeh-Bavili M. Mechanisms of miRNA-Mediated Gene Regulation from Common Downregulation to mRNA-Specific Upregulation. Int J Genomics 2014; 2014:970607; PMID:25180174
- Elbashir SM, Harborth J, Lendeckel W, Yalcin A, Weber K, Tuschl T. Duplexes of 21-nucleotide RNAs mediate RNA interference in cultured mammalian cells. Nature 2001; 411:494-8; PMID:11373684; http://dx.doi.org/10.1038/35078107
- Mellitzer G, Hallonet M, Chen L, Ang SL. Spatial and temporal ‘knock down’ of gene expression by electroporation of double-stranded RNA and morpholinos into early postimplantation mouse embryos. Mech Dev 2002; 118:57-63; PMID:12351170; http://dx.doi.org/10.1016/S0925-4773(02)00191-0
- Calegari F, Haubensak W, Yang D, Huttner WB, Buchholz F. Tissue-specific RNA interference in postimplantation mouse embryos with endoribonuclease-prepared short interfering RNA. Proc Natl Acad Sci U S A 2002; 99:14236-40; PMID:12391321; http://dx.doi.org/10.1073/pnas.192559699
- Zeliadt N. Big pharma shows signs of renewed interest in RNAi drugs. Nat Med 2014; 20:109; PMID:24504395; http://dx.doi.org/10.1038/nm0214-109
- Open Label Study for the Evaluation of Tolerability of Five Dose Levels of Cand5. (2004). Clinical Trial Phase I
- A Dose Escalation and Safety Study of I5NP to Prevent Acute Kidney Injury (AKI) in Patients at High Risk of AKI Undergoing Major Cardiovascular Surgery (QRK.004). (2008). Clinical Trial Phase I (Terminated)
- Safety & Efficacy Study Evaluating the Combination of Bevasiranib & Lucentis Therapy in Wet AMD (COBALT). (2007). Clinical Trial Phase III (Terminated)
- Study Evaluating Efficacy and Safety of PF-04523655 Versus Laser in Subjects With Diabetic Macular Edema (DEGAS). (2008). Clinical Trial Phase II (Terminated)
- Study to Evaluate the Safety, Tolerability, Pharmacokinetics (PK), and Pharmacodynamics (PD) of Liposomal siRNA in Subjects With High Cholesterol. (2009). Clinical Trial Phase I (Terminated)
- A Study Using Intravitreal Injections of a Small Interfering RNA in Patients With Age-Related Macular Degeneration. (2006). Clinical Trial Phase II (Terminated)
- Conde J, Artzi N. Are RNAi and miRNA therapeutics truly dead? Trends Biotechnol 2015; 33:141-4; PMID:25595555; http://dx.doi.org/10.1016/j.tibtech.2014.12.005
- Krieg AM. Is RNAi dead? Mol Ther 2011; 19:1001-2; PMID:21629254; http://dx.doi.org/10.1038/mt.2011.94
- Coelho T, Adams D, Silva A, Lozeron P, Hawkins PN, Mant T, Perez J, Chiesa J, Warrington S, Tranter E, et al. Safety and efficacy of RNAi therapy for transthyretin amyloidosis. N Engl J Med 2013; 369:819-29; PMID:23984729; http://dx.doi.org/10.1056/NEJMoa1208760
- Fitzgerald K, Frank-Kamenetsky M, Shulga-Morskaya S, Liebow A, Bettencourt BR, Sutherland JE, Hutabarat RM, Clausen VA, Karsten V, Cehelsky J, et al. Effect of an RNA interference drug on the synthesis of proprotein convertase subtilisin/kexin type 9 (PCSK9) and the concentration of serum LDL cholesterol in healthy volunteers: a randomised, single-blind, placebo-controlled, phase 1 trial. Lancet 2014; 383:60-8; PMID:24094767; http://dx.doi.org/10.1016/S0140-6736(13)61914-5
- van de Water FM, Boerman OC, Wouterse AC, Peters JG, Russel FG, Masereeuw R. Intravenously administered short interfering RNA accumulates in the kidney and selectively suppresses gene function in renal proximal tubules. Drug Metab Dispos 2006; 34:1393-7; PMID:16714375; http://dx.doi.org/10.1124/dmd.106.009555
- Alagia A, Eritja R. siRNA and RNAi optimization. Wiley interdisciplinary reviews RNA, 2016 7; 316-329; PMID:26840434; http://dx.doi.org/10.1002/wrna.1337
- Lorenzer C, Dirin M, Winkler AM, Baumann V, Winkler J. Going beyond the liver: progress and challenges of targeted delivery of siRNA therapeutics. J Control Release 2015; 203:1-15; PMID:25660205; http://dx.doi.org/10.1016/j.jconrel.2015.02.003
- Jeong EH, Kim H, Jang B, Cho H, Ryu J, Kim B, Park Y, Kim J, Lee JB, Lee H. Technological development of structural DNA/RNA-based RNAi systems and their applications. Adv Drug Deliv Rev 2016; 107:29-43; PMID:26494399; http://dx.doi.org/10.1016/j.addr.2015.10.008
- Bramsen JB, Kjems J. Engineering small interfering RNAs by strategic chemical modification. Methods Mol Biol 2013; 942:87-109; PMID:23027047; http://dx.doi.org/10.1007/978-1-62703-119-6_5
- Phase 1b/2, Open Label, Repeat Dose, Dose Escalation Study of ND-L02-s0201 Injection in Subjects With Moderate to Extensive Fibrosis (METAVIR F3-4). (2014). Clinical Trial Phase I
- Study of ARB-001467 in Subjects With Chronic HBV Infection Receiving Nucleos(t)Ide Analogue Therapy. (2015). Clinical Trial Phase II
- A Phase 1 Study of ALN-AS1 in Patients With Acute Intermittent Porphyria (AIP). (2015). Clinical Trial Phase I
- ENDEAVOUR: Phase 3 Multicenter Study of Revusiran (ALN-TTRSC) in Patients With Transthyretin (TTR) Mediated Familial Amyloidotic Cardiomyopathy (FAC). (2014). Clinical Trial Phase III
- A Phase 1 Study of an Investigational Drug, ALN-AT3SC, in Healthy Volunteers and Hemophilia A or B Patients. (2014). Clinical Trial Phase I
- Phase I Intratumoral Pbi-shRNA STMN1 LP in Advanced and/or Metastatic Cancer (STMN1-LP). (2012). Clinical Trial Phase I
- APN401 in Treating Patients With Melanoma, Kidney Cancer, Pancreatic Cancer, or Other Solid Tumors That Are Metastatic or Cannot Be Removed By Surgery. (2014). Clinical Trial Phase I
- SYL040012, Treatment for Open Angle Glaucoma (SYLTAG). (2014). Clinical Trial Phase II
- Phase 2/3, Randomized, Double-Masked, Sham-Controlled Trial of QPI-1007 in Subjects With Acute Nonarteritic Anterior Ischemic Optic Neuropathy (NAION). (2015). Clinical Trial Phase II/III
- Bobbin ML, Rossi JJ. RNA Interference (RNAi)-Based Therapeutics: Delivering on the Promise? Annu Rev Pharmacol Toxicol 2016; 56:103-22; PMID:26738473; http://dx.doi.org/10.1146/annurev-pharmtox-010715-103633
- Lam JK, Chow MY, Zhang Y, Leung SW. siRNA Versus miRNA as Therapeutics for Gene Silencing. Mol Ther Nucleic Acids 2015; 4:e252; PMID:26372022; http://dx.doi.org/10.1038/mtna.2015.23
- Long-term Extension to Miravirsen Study in Null Responder to Pegylated Interferon Alpha Plus Ribavirin Subjects With Chronic Hepatitis C. (2014). Clinical Trial Phase II
- Lee CH, Kim JH, Lee SW. The role of microRNAs in hepatitis C virus replication and related liver diseases. J Microbiol 2014; 52:445-51; PMID:24871972; http://dx.doi.org/10.1007/s12275-014-4267-x
- MesomiR 1: A Phase I Study of TargomiRs as 2nd or 3rd Line Treatment for Patients With Recurrent MPM and NSCLC. (2015). Clinical Trial Phase I
- A Multicenter Phase I Study of MRX34, MicroRNA miR-RX34 Liposomal Injection. (2013). Clinical Trial Phase I
- Wolff JA, Malone RW, Williams P, Chong W, Acsadi G, Jani A, Felgner PL. Direct gene transfer into mouse muscle in vivo. Science 1990; 247:1465-8; PMID:1690918; http://dx.doi.org/10.1126/science.1690918
- Jirikowski GF, Sanna PP, Maciejewski-Lenoir D, Bloom FE. Reversal of diabetes insipidus in Brattleboro rats: intrahypothalamic injection of vasopressin mRNA. Science 1992; 255:996-8; PMID:1546298; http://dx.doi.org/10.1126/science.1546298
- Isaacs A, Cox RA, Rotem Z. Foreign nucleic acids as the stimulus to make interferon. Lancet 1963; 2:113-6; PMID:13956740; http://dx.doi.org/10.1016/S0140-6736(63)92585-6
- Weissman D. mRNA transcript therapy. Expert Rev Vaccines 2015; 14:265-81; PMID:25359562; http://dx.doi.org/10.1586/14760584.2015.973859
- Alexopoulou L, Holt AC, Medzhitov R, Flavell RA. Recognition of double-stranded RNA and activation of NF-kappaB by Toll-like receptor 3. Nature 2001; 413:732-8; PMID:11607032; http://dx.doi.org/10.1038/35099560
- Anderson BR, Muramatsu H, Nallagatla SR, Bevilacqua PC, Sansing LH, Weissman D, Karikó K. Incorporation of pseudouridine into mRNA enhances translation by diminishing PKR activation. Nucleic Acids Res 2010; 38:5884-92; PMID:20457754; http://dx.doi.org/10.1093/nar/gkq347
- Kariko K, Muramatsu H, Welsh FA, Ludwig J, Kato H, Akira S, Weissman D. Incorporation of pseudouridine into mRNA yields superior nonimmunogenic vector with increased translational capacity and biological stability. Mol Ther 2008; 16:1833-40; PMID:18797453; http://dx.doi.org/10.1038/mt.2008.200
- Goubau D, Deddouche S, Reis e Sousa C. Cytosolic sensing of viruses. Immunity 2013; 38:855-69; PMID:23706667; http://dx.doi.org/10.1016/j.immuni.2013.05.007
- Kariko K, Buckstein M, Ni H, Weissman D. Suppression of RNA recognition by Toll-like receptors: the impact of nucleoside modification and the evolutionary origin of RNA. Immunity 2005; 23:165-75; PMID:16111635; http://dx.doi.org/10.1016/j.immuni.2005.06.008
- Kormann MS, Hasenpusch G, Aneja MK, Nica G, Flemmer AW, Herber-Jonat S, Huppmann M, Mays LE, Illenyi M, Schams A, et al. Expression of therapeutic proteins after delivery of chemically modified mRNA in mice. Nat Biotechnol 2011; 29:154-7; PMID:21217696; http://dx.doi.org/10.1038/nbt.1733
- Karijolich J, Yu YT. Converting nonsense codons into sense codons by targeted pseudouridylation. Nature 2011; 474:395-8; PMID:21677757; http://dx.doi.org/10.1038/nature10165
- Summer H, Gramer R, Droge P. Denaturing urea polyacrylamide gel electrophoresis (Urea PAGE). J Vis Exp 2009; PMID:19865070; http://dx.doi.org/10.3791/1485(2009)
- Kariko K, Muramatsu H, Ludwig J, Weissman D. Generating the optimal mRNA for therapy: HPLC purification eliminates immune activation and improves translation of nucleoside-modified, protein-encoding mRNA. Nucleic Acids Res 2011; 39:e142; PMID:21890902; http://dx.doi.org/10.1093/nar/gkr695
- Pasquinelli AE, Dahlberg JE, Lund E. Reverse 5' caps in RNAs made in vitro by phage RNA polymerases. RNA 1995; 1:957-67; PMID:8548660
- Stepinski J, Waddell C, Stolarski R, Darzynkiewicz E, Rhoads RE. Synthesis and properties of mRNAs containing the novel “anti-reverse” cap analogs 7-methyl(3'-O-methyl)GpppG and 7-methyl (3'-deoxy)GpppG. RNA 2001; 7:1486-95; PMID:11680853; http://dx.doi.org/10.1017.S1355838201014078
- Grudzien-Nogalska E, Jemielity J, Kowalska J, Darzynkiewicz E, Rhoads RE. Phosphorothioate cap analogs stabilize mRNA and increase translational efficiency in mammalian cells. RNA 2007; 13:1745-55; PMID:17720878; http://dx.doi.org/10.1261/rna.701307
- Fath S, Bauer AP, Liss M, Spriestersbach A, Maertens B, Hahn P, Ludwig C, Schäfer F, Graf M, Wagner R. Multiparameter RNA and codon optimization: a standardized tool to assess and enhance autologous mammalian gene expression. PLoS one 2011; 6:e17596; PMID:21408612; http://dx.doi.org/10.1371/journal.pone.0017596
- Al-Saif M, Khabar KS. UU/UA dinucleotide frequency reduction in coding regions results in increased mRNA stability and protein expression. Mol Ther 2012; 20:954-9; PMID:22434136; http://dx.doi.org/10.1038/mt.2012.29
- Weissman D, Kariko K. mRNA: Fulfilling the Promise of Gene Therapy. Mol Ther 2015; 23:1416-7; PMID:26321183; http://dx.doi.org/10.1038/mt.2015.138
- Zubiaga AM, Belasco JG, Greenberg ME. The nonamer UUAUUUAUU is the key AU-rich sequence motif that mediates mRNA degradation. Mol Cell Biol 1995; 15:2219-30; PMID:7891716; http://dx.doi.org/10.1128/MCB.15.4.2219
- Peixeiro I, Silva AL, Romao L. Control of human β-globin mRNA stability and its impact on β-thalassemia phenotype. Haematologica 2011; 96:905-13; PMID:21357703; http://dx.doi.org/10.3324/haematol.2010.039206
- Waggoner SA, Liebhaber SA. Regulation of α-globin mRNA stability. Exp Biol Med (Maywood) 2003; 228:387-95; PMID:12671183
- Withers JB, Beemon KL. The structure and function of the rous sarcoma virus RNA stability element. J Cell Biochem 2011; 112:3085-92; PMID:21769913; http://dx.doi.org/10.1002/jcb.23272
- Gallie DR. The cap and poly(A) tail function synergistically to regulate mRNA translational efficiency. Genes Dev 1991; 5:2108-16; PMID:1682219; http://dx.doi.org/10.1101/gad.5.11.2108
- Peng J, Murray EL, Schoenberg DR. In vivo and in vitro analysis of poly(A) length effects on mRNA translation. Methods Mol Biol 2008; 419:215-30; PMID:18369986; http://dx.doi.org/10.1007/978-1-59745-033-1_15
- Balmayor ER, Geiger JP, Aneja MK, Berezhanskyy T, Utzinger M, Mykhaylyk O, Rudolph C, Plank C. Chemically modified RNA induces osteogenesis of stem cells and human tissue explants as well as accelerates bone healing in rats. Biomaterials 2016; 87:131-46; PMID:26923361; http://dx.doi.org/10.1016/j.biomaterials.2016.02.018
- Elangovan S, Khorsand B, Do AV, Hong L, Dewerth A, Kormann M, Ross RD, Sumner DR, Allamargot C, Salem AK. Chemically modified RNA activated matrices enhance bone regeneration. J Control Release 2015; 218:22-8; PMID:26415855; http://dx.doi.org/10.1016/j.jconrel.2015.09.050
- Plews JR, Li J, Jones M, Moore HD, Mason C, Andrews PW, Na J. Activation of pluripotency genes in human fibroblast cells by a novel mRNA based approach. PloS one 2010; 5:e14397; PMID:21209933; http://dx.doi.org/10.1371/journal.pone.0014397
- Warren L, Manos PD, Ahfeldt T, Loh YH, Li H, Lau F, Ebina W, Mandal PK, Smith ZD, Meissner A, et al. Highly efficient reprogramming to pluripotency and directed differentiation of human cells with synthetic modified mRNA. Cell Stem Cell 2010; 7:618-30; PMID:20888316; http://dx.doi.org/10.1016/j.stem.2010.08.012
- Mandal PK, Rossi DJ. Reprogramming human fibroblasts to pluripotency using modified mRNA. Nat Protoc 2013; 8:568-82; PMID:23429718; http://dx.doi.org/10.1038/nprot.2013.019
- Preskey D, Allison TF, Jones M, Mamchaoui K, Unger C. Synthetically modified mRNA for efficient and fast human iPS cell generation and direct transdifferentiation to myoblasts. Biochem Biophys Res Commun 2015; PMID:26449459; http://dx.doi.org/10.1016/j.bbrc.2015.09.102
- Simeonov KP, Uppal H. Direct reprogramming of human fibroblasts to hepatocyte-like cells by synthetic modified mRNAs. PloS One 2014; 9:e100134; PMID:24963715; http://dx.doi.org/10.1371/journal.pone.0100134
- Kariko K, Muramatsu H, Keller JM, Weissman D. Increased erythropoiesis in mice injected with submicrogram quantities of pseudouridine-containing mRNA encoding erythropoietin. Mol Ther 2012; 20:948-53; PMID:22334017; http://dx.doi.org/10.1038/mt.2012.7
- Yin H, Xue W, Chen S, Bogorad RL, Benedetti E, Grompe M, Koteliansky V, Sharp PA, Jacks T, Anderson DG. Genome editing with Cas9 in adult mice corrects a disease mutation and phenotype. Nat Biotechnol 2014; 32:551-3; PMID:24681508; http://dx.doi.org/10.1038/nbt.2884
- Wang X, Yu H, Lei A, Zhou J, Zeng W, Zhu H, Niu Y, Shi B, Cai B, Liu J, et al. Generation of gene-modified goats targeting MSTN and FGF5 via zygote injection of CRISPR/Cas9 system. Sci Rep 2015; 5:13878; PMID:26354037; http://dx.doi.org/10.1038/srep13878
- Kaneko T, Mashimo T. Simple Genome Editing of Rodent Intact Embryos by Electroporation. PloS one 2015; 10:e0142755; PMID:26556280; http://dx.doi.org/10.1371/journal.pone.0142755
- Wang T, Hong Y. Direct gene disruption by TALENs in medaka embryos. Gene 2014; 543:28-33; PMID:24713411; http://dx.doi.org/10.1016/j.gene.2014.04.013
- Yang D, Zhang J, Xu J, Zhu T, Fan Y, Fan J, Chen YE. Production of apolipoprotein C-III knockout rabbits using zinc finger nucleases. J Vis Exp 2013; 81; e50957; PMID:24301055; http://dx.doi.org/10.3791/50957
- Flisikowska T, Thorey IS, Offner S, Ros F, Lifke V, Zeitler B, Rottmann O, Vincent A, Zhang L, Jenkins S, et al. Efficient immunoglobulin gene disruption and targeted replacement in rabbit using zinc finger nucleases. PloS One 2011; 6:e21045; PMID:21695153; http://dx.doi.org/10.1371/journal.pone.0021045
- Williams DA, Thrasher AJ. Concise review: lessons learned from clinical trials of gene therapy in monogenic immunodeficiency diseases. Stem Cells Transl Med 2014; 3:636-42; PMID:24682287; http://dx.doi.org/10.5966/sctm.2013-0206
- Schirmbeck R, Reimann J, Kochanek S, Kreppel F. The immunogenicity of adenovirus vectors limits the multispecificity of CD8 T-cell responses to vector-encoded transgenic antigens. Mol Ther 2008; 16:1609-16; PMID:18612271; http://dx.doi.org/10.1038/mt.2008.141
- Mahiny AJ, Dewerth A, Mays LE, Alkhaled M, Mothes B, Malaeksefat E, Loretz B, Rottenberger J, Brosch DM, Reautschnig P, et al. In vivo genome editing using nuclease-encoding mRNA corrects SP-B deficiency. Nat Biotechnol 2015; 33:584-6; PMID:25985262; http://dx.doi.or/10.1038/nbt.3241
- Allers K, Hutter G, Hofmann J, Loddenkemper C, Rieger K, Thiel E, Schneider T. Evidence for the cure of HIV infection by CCR5Delta32/Delta32 stem cell transplantation. Blood 2011; 117:2791-9; PMID:21148083; http://dx.doi.org/10.1182/blood-2010-09-309591
- Evaluation the Safety and Tolerability of i.v. Administration of a Cancer Vaccine in Patients With Advanced Melanoma (Lipo-MERIT). (2015). Clinical Trial Phase I
- Wissink EM, Fogarty EA, Grimson A. High-throughput discovery of post-transcriptional cis-regulatory elements. BMC Genomics 2016; 17:177; PMID:26941072; http://dx.doi.org/10.1186/s12864-016-2479-7
- Desmet CJ, Ishii KJ. Nucleic acid sensing at the interface between innate and adaptive immunity in vaccination. Nat Rev Immunol 2012; 12:479-91; PMID:22728526; http://dx.doi.org/10.1038/nri3247
- Brubaker SW, Bonham KS, Zanoni I, Kagan JC. Innate immune pattern recognition: a cell biological perspective. Annu Rev Immunol 2015; 33:257-90; PMID:25581309; http://dx.doi.org/10.1146/annurev-immunol-032414-112240
- Clark GJ, Angel N, Kato M, Lopez JA, MacDonald K, Vuckovic S, Hart DN. The role of dendritic cells in the innate immune system. Microbes Infect 2000; 2:257-72; PMID:10758402; http://dx.doi.org/10.1016/S1286-4579(00)00302-6
- Jacobson JM, Routy JP, Welles S, DeBenedette M, Tcherepanova I, Angel JB, Asmuth DM, Stein DK, Baril JG, McKellar M, et al. Dendritic Cell Immunotherapy for HIV-1 Infection Using Autologous HIV-1 RNA: A Randomized, Double-Blind, Placebo-Controlled Clinical Trial. J Acquir Immune Defic Syndr 2016; 72:31-8; PMID:26751016; http://dx.doi.org/10.1097/QAI.0000000000000926
- Weiss R, Scheiblhofer S, Thalhamer J. Allergens are not pathogens: why immunization against allergy differs from vaccination against infectious diseases. Hum Vaccin Immunother 2014; 10:703-7; PMID:24280693; http://dx.doi.org/10.4161/hv.27183
- Weiss R, Scheiblhofer S, Roesler E, Ferreira F, Thalhamer J. Prophylactic mRNA vaccination against allergy. Curr Opin Allergy Clin Immunol 2010; 10:567-74; PMID:20856111; http://dx.doi.org/10.1097/ACI.0b013e32833fd5b6
- Fotin-Mleczek M, Duchardt KM, Lorenz C, Pfeiffer R, Ojkic-Zrna S, Probst J, Kallen KJ. Messenger RNA-based vaccines with dual activity induce balanced TLR-7 dependent adaptive immune responses and provide antitumor activity. J Immunother 2011; 34:1-15; PMID:21150709; http://dx.doi.org/10.1097/CJI.0b013e3181f7dbe8
- Fotin-Mleczek M, Zanzinger K, Heidenreich R, Lorenz C, Kowalczyk A, Kallen KJ, et al. mRNA-based vaccines synergize with radiation therapy to eradicate established tumors. Radiat Oncol 2014; 9:180; PMID:25127546; http://dx.doi.org/10.1186/1748-717X-9-180
- Schmidt MA, Goodwin TJ. Personalized medicine in human space flight: using Omics based analyses to develop individualized countermeasures that enhance astronaut safety and performance. Metabolomics 2013; 9:1134-56; PMID:24273472; http://dx.doi.org/10.1007/s11306-013-0556-3
- Petsch B, Schnee M, Vogel AB, Lange E, Hoffmann B, Voss D, Schlake T, Thess A, Kallen KJ, Stitz L, et al. Protective efficacy of in vitro synthesized, specific mRNA vaccines against influenza A virus infection. Nat Biotechnol 2012; 30:1210-6; PMID:23159882; http://dx.doi.org/10.1038/nbt.2436
- Pascolo S. Vaccination with messenger RNA. Methods Mol Med 2006; 127:23-40; PMID:16988444; htpp://dx.doi.org/10.1385/1-59745-168-1:23
- Mortimer I, Tam P, MacLachlan I, Graham RW, Saravolac EG, Joshi PB. Cationic lipid-mediated transfection of cells in culture requires mitotic activity. Gene Ther 1999; 6:403-11; PMID:10435090; http://dx.doi.org/10.1038/sj.gt.3300837
- Wong SS, Webby RJ. An mRNA vaccine for influenza. Nat Biotechnol 2012; 30:1202-4; PMID:23222788; http://dx.doi.org/10.1038/nbt.2439
- Ichino M, Mor G, Conover J, Weiss WR, Takeno M, Ishii KJ, Klinman DM. Factors associated with the development of neonatal tolerance after the administration of a plasmid DNA vaccine. J Immunol 1999; 162:3814-8; PMID:10201898
- Ponsaerts P, Van Tendeloo VF, Berneman ZN. Cancer immunotherapy using RNA-loaded dendritic cells. Clin Exp Immunol 2003; 134:378-84; PMID:14632740; http://dx.doi.org/10.1046/j.1365-2249.2003.02286.x
- Vik-Mo EO, Nyakas M, Mikkelsen BV, Moe MC, Due-Tonnesen P, Suso EM, Sæbøe-Larssen S, Sandberg C, Brinchmann JE, Helseth E, et al. Therapeutic vaccination against autologous cancer stem cells with mRNA-transfected dendritic cells in patients with glioblastoma. Cancer Immunol Immunother 2013; 62:1499-509; PMID:23817721; http://dx.doi.org/10.1007/s00262-013-1453-3
- Van Gulck E, Vlieghe E, Vekemans M, Van Tendeloo VF, Van De Velde A, Smits E, Anguille S, Cools N, Goossens H, Mertens L, et al. mRNA-based dendritic cell vaccination induces potent antiviral T-cell responses in HIV-1-infected patients. AIDS 2012; 26:F1-12; PMID:22156965; http://dx.doi.org/10.1097/QAD.0b013e32834f33e8
- Aarntzen EH, Schreibelt G, Bol K, Lesterhuis WJ, Croockewit AJ, de Wilt JH, van Rossum MM, Blokx WA, Jacobs JF, Duiveman-de Boer T, et al. Vaccination with mRNA-electroporated dendritic cells induces robust tumor antigen-specific CD4+ and CD8+ T cells responses in stage III and IV melanoma patients. Clin Cancer Res 2012; 18:5460-70; PMID:22896657; http://dx.doi.org/10.1158/1078-0432.CCR-11-3368
- Suso EM, Dueland S, Rasmussen AM, Vetrhus T, Aamdal S, Kvalheim G, Gaudernack G. hTERT mRNA dendritic cell vaccination: complete response in a pancreatic cancer patient associated with response against several hTERT epitopes. Cancer Immunol Immunother 2011; 60:809-18; PMID:21365467; http://dx.doi.org/10.1007/s00262-011-0991-9
- Van Tendeloo VF, Van de Velde A, Van Driessche A, Cools N, Anguille S, Ladell K, Gostick E, Vermeulen K, Pieters K, Nijs G, et al. Induction of complete and molecular remissions in acute myeloid leukemia by Wilms' tumor 1 antigen-targeted dendritic cell vaccination. Proc Natl Acad Sci U S A 2010; 107:13824-9; PMID:20631300; http://dx.doi.org/10.1073/pnas.1008051107
- Routy JP, Boulassel MR, Yassine-Diab B, Nicolette C, Healey D, Jain R, Landry C, Yegorov O, Tcherepanova I, Monesmith T, et al. Immunologic activity and safety of autologous HIV RNA-electroporated dendritic cells in HIV-1 infected patients receiving antiretroviral therapy. Clin Immunol 2010; 134:140-7; PMID:19889582; http://dx.doi.org/10.1016/j.clim.2009.09.009
- Coosemans A, Wolfl M, Berneman ZN, Van Tendeloo V, Vergote I, Amant F, Van Gool SW. Immunological response after therapeutic vaccination with WT1 mRNA-loaded dendritic cells in end-stage endometrial carcinoma. Anticancer Res 2010; 30:3709-14; PMID:20944158
- Phase 3 Trial of Autologous Dendritic Cell Immunotherapy (AGS-003) Plus Standard Treatment of Advanced Renal Cell Carcinoma (RCC) (ADAPT). (2012). Clinical Trial Phase III
- Wilgenhof S, Corthals J, Heirman C, van Baren N, Lucas S, Kvistborg P, Thielemans K, Neyns B. Phase II Study of Autologous Monocyte-Derived mRNA Electroporated Dendritic Cells (TriMixDC-MEL) Plus Ipilimumab in Patients With Pretreated Advanced Melanoma. J Clin Oncol 2016; 34:1330-8; PMID:26926680; http://dx.doi.org/10.1200/JCO.2015.63.4121
- Bol KF, Figdor CG, Aarntzen EH, Welzen ME, van Rossum MM, Blokx WA, van de Rakt MW, Scharenborg NM, de Boer AJ, Pots JM, et al. Intranodal vaccination with mRNA-optimized dendritic cells in metastatic melanoma patients. Oncoimmunology 2015; 4:e1019197; PMID:26405571; http://dx.doi.org/10.1080/2162402X.2015.1019197
- MiHA-loaded PD-L-silenced DC Vaccination After Allogeneic SCT (PSCT19). (2016). Clinical Trial Phase II
- Adjuvant Dendritic Cell-immunotherapy Plus Temozolomide in Glioblastoma Patients (ADDIT-GLIO). (2016). Clinical Trial Phase II
- Autologous Dendritic Cell Vaccination in Mesothelioma (MESODEC). (2016). Clinical Trial Phase II
- Vaccine Therapy for the Treatment of Newly Diagnosed Glioblastoma Multiforme (ATTAC-II). (2016). Clinical Trial Phase II
- Weide B, Pascolo S, Scheel B, Derhovanessian E, Pflugfelder A, Eigentler TK, Pawelec G, Hoerr I, Rammensee HG, Garbe C. Direct injection of protamine-protected mRNA: results of a phase 1/2 vaccination trial in metastatic melanoma patients. J Immunother 2009; 32:498-507; PMID:19609242; http://dx.doi.org/10.1097/CJI.0b013e3181a00068
- RNActive® Rabies Vaccine (CV7201) in Healthy Adults. (2014). Clinical Trial Phase I
- Hekele A, Bertholet S, Archer J, Gibson DG, Palladino G, Brito LA, Otten GR, Brazzoli M, Buccato S, Bonci A, et al. Rapidly produced SAM((R)) vaccine against H7N9 influenza is immunogenic in mice. Emerg Microbes Infect 2013; 2:e52; PMID:26038486; http://dx.doi.org/10.1038/emi.2013.54
- Trial of RNActive®-Derived Cancer Vaccine and Local Radiation in in Stage IV Non Small Cell Lung Cancer (NSCLC). (2013). Clinical Trial Phase I
- Safety and Efficacy Trial of a RNActive®-Derived Prostate Cancer Vaccine in Hormone Refractory Disease. (2009). Clinical Trial Phase II
- Trial of RNActive®-Derived Prostate Cancer Vaccine in Metastatic Castrate-refractory Prostate Cancer. (2013). Clinical Trial Phase II
- An Open Label Randomised Trial of RNActive® Cancer Vaccine in High Risk and Intermediate Risk Patients With Prostate Cancer. (2014). Clinical Trial Phase II
- IVAC MUTANOME Phase I Clinical Trial. (2014). Clinical Trial Phase I
- Heidenreich R, Jasny E, Kowalczyk A, Lutz J, Probst J, Baumhof P, Scheel B, Voss S, Kallen KJ, Fotin-Mleczek M. A novel RNA-based adjuvant combines strong immunostimulatory capacities with a favorable safety profile. Int J Cancer 2015; 137:372-84; PMID:25530186; http://dx.doi.org/10.1002/ijc.29402
- CureVac Press Release. (http://www.curevac.com/fileadmin/curevac.de/media/Content/Newsroom/20151221_CureVac_Press_release_JP_Morgan.pdf). 2015
- Islam MA, Reesor EK, Xu Y, Zope HR, Zetter BR, Shi J. Biomaterials for mRNA delivery. Biomater Sci 2015; 3:1519-33; PMID:26280625; http://dx.doi.org/10.1039/C5BM00198F
- Geall AJ, Verma A, Otten GR, Shaw CA, Hekele A, Banerjee K, Cu Y, Beard CW, Brito LA, Krucker T, et al. Nonviral delivery of self-amplifying RNA vaccines. Proc Natl Acad Sci U S A 2012; 109:14604-9; PMID:22908294; http://dx.doi.org/10.1073/pnas.1209367109
- Rodriguez-Gascon A, del Pozo-Rodriguez A, Solinis MA. Development of nucleic acid vaccines: use of self-amplifying RNA in lipid nanoparticles. Int J Nanomedicine 2014; 9:1833-43; PMID:24748793; http://dx.doi.org/10.2147/IJN.S39810
- Xu J, Luft JC, Yi X, Tian S, Owens G, Wang J, Johnson A, Berglund P, Smith J, Napier ME, et al. RNA replicon delivery via lipid-complexed PRINT protein particles. Mol Pharm 2013; 10:3366-74; PMID:23924216; http://dx.doi.org/10.1021/mp400190z
- Pascolo S. The messenger's great message for vaccination. Expert Rev Vaccines 2015; 14:153-6; PMID:25586101; http://dx.doi.org/10.1586/14760584.2015.1000871
- Hattinger E, Scheiblhofer S, Roesler E, Thalhamer T, Thalhamer J, Weiss R. Prophylactic mRNA Vaccination against Allergy Confers Long-Term Memory Responses and Persistent Protection in Mice. J Immunol Res 2015; 2015:797421; PMID:26557723; http://dx.doi.org/10.1155/2015/797421
- Nelson HS, Oppenheimer J, Vatsia GA, Buchmeier A. A double-blind, placebo-controlled evaluation of sublingual immunotherapy with standardized cat extract. J Allergy Clin Immunol 1993; 92:229-36; PMID:8349933; http://dx.doi.org/10.1016/0091-6749(93)90166-D
- Roesler E, Weiss R, Weinberger EE, Fruehwirth A, Stoecklinger A, Mostbock S, Ferreira F, Thalhamer J, Scheiblhofer S. Immunize and disappear-safety-optimized mRNA vaccination with a panel of 29 allergens. J Allergy Clin Immunol 2009; 124:1070-7 e1-11; PMID:19665781; http://dx.doi.org/10.1016/j.jaci.2009.06.036
- Ball T, Sperr WR, Valent P, Lidholm J, Spitzauer S, Ebner C, Kraft D, Valenta R. Induction of antibody responses to new B cell epitopes indicates vaccination character of allergen immunotherapy. Eur J Immunol 1999; 29:2026-36; PMID:10382766; http://dx.doi.org/10.1002/(SICI)1521-4141(199906)29:06%3c2026::AID-IMMU2026%3e3.0.CO;2-2
- Van Ree R, Van Leeuwen WA, Dieges PH, Van Wijk RG, De Jong N, Brewczyski PZ, Kroon AM, Schilte PP, Tan KY, Simon-Licht IF, et al. Measurement of IgE antibodies against purified grass pollen allergens (Lol p 1, 2, 3 and 5) during immunotherapy. Clin Exp Allergy 1997; 27:68-74; PMID:9117883; http://dx.doi.org/10.1046/j.1365-2222.1997.d01-416.x
- Stafforst T, Schneider MF. An RNA-deaminase conjugate selectively repairs point mutations. Angewandte Chemie 2012; 51:11166-9; PMID:23038402; http://dx.doi.org/10.1002/anie.201206489
- Schneider MF, Wettengel J, Hoffmann PC, Stafforst T. Optimal guideRNAs for re-directing deaminase activity of hADAR1 and hADAR2 in trans. Nucleic Acids Res 2014; 42:e87; PMID:24744243; http://dx.doi.org/10.1093/nar/gku272
- Montiel-Gonzalez MF, Vallecillo-Viejo I, Yudowski GA, Rosenthal JJ. Correction of mutations within the cystic fibrosis transmembrane conductance regulator by site-directed RNA editing. Proc Natl Acad Sci U S A 2013; 110:18285-90; PMID:24108353; http://dx.doi.org/10.1073/pnas.1306243110
- Bass BL. RNA editing by adenosine deaminases that act on RNA. Annu Rev Biochem 2002; 71:817-46; PMID:12045112; http://dx.doi.org/10.1146/annurev.biochem.71.110601.135501
- Nishikura K. Functions and regulation of RNA editing by ADAR deaminases. Annu Rev Biochem 2010; 79:321-49; PMID:20192758; http://dx.doi.org/10.1146/annurev-biochem-060208-105251
- Vogel P, Stafforst T. Site-directed RNA editing with antagomir deaminases–a tool to study protein and RNA function. ChemMedChem 2014; 9:2021-5; PMID:24954543; http://dx.doi.org/10.1002/cmdc.201402139
- Vogel P, Schneider MF, Wettengel J, Stafforst T. Improving site-directed RNA editing in vitro and in cell culture by chemical modification of the guideRNA. Angewandte Chemie 2014; 53:6267-71; PMID:24890431; http://dx.doi.org/10.1002/anie.201402634
- Hanswillemenke A, Kuzdere T, Vogel P, Jékely G, Stafforst T. Site-Directed RNA Editing in Vivo Can Be Triggered by the Light-Driven Assembly of an Artificial Riboprotein. J Am Chem Soc 2015; 137:15875-81; PMID:26594902; http://dx.doi.org/10.1021/jacs.5b10216
- Zhao X, Yu Y-T. Targeted pre-mRNA modification for gene silencing and regulation. Nat Methods 2008; 5:95-100; PMID:18066073; http://dx.doi.org/10.1038/nmeth1142
- Kole R, Krainer AR, Altman S. RNA therapeutics: beyond RNA interference and antisense oligonucleotides. Nat Rev Drug Discov 2012; 11:125-40; PMID:22262036; http://dx.doi.org/10.1038/nrd3625
- Blanc V, Davidson NO. C-to-U RNA editing: mechanisms leading to genetic diversity. J Biol Chem 2003; 278:1395-8; PMID:12446660; http://dx.doi.org/10.1074/jbc.R200024200
- Liu N, Pan T. N6-methyladenosine-encoded epitranscriptomics. Nat Struct Mol Biol 2016; 23:98-102; PMID:26840897; http://dx.doi.org/10.1038/nsmb.3162
- Machnicka MA, Milanowska K, Oglou OO, Purta E, Kurkowska M, Olchowik A, Januszewski W, Kalinowski S, Dunin-Horkawicz S, Rother KM, et al. MODOMICS: a database of RNA modification pathways—2013 update. Nucleic Acids Res 2013; 41:D262-D7; PMID:23118484; http://dx.doi.org/10.1093/nar/gks1007
- Muttach F, Rentmeister A. A Biocatalytic Cascade for Versatile One‐Pot Modification of mRNA Starting from Methionine Analogues. Angewandte Chemie International Edition 2016; 55:1917-20; http://dx.doi.org/10.1002/anie.201507577
- Vickers TA, Wyatt JR, Burckin T, Bennett CF, Freier SM. Fully modified 2′ MOE oligonucleotides redirect polyadenylation. Nucleic Acids Res 2001; 29:1293-9; PMID:11238995; http://dx.doi.org/10.1093/nar/29.6.1293
- Xiong Y, Steitz TA. Mechanism of transfer RNA maturation by CCA-adding enzyme without using an oligonucleotide template. Nature 2004; 430:640-5; PMID:15295590; http://dx.doi.org/10.1038/nature02711
- Cho HD, Verlinde CL, Weiner AM. Reengineering CCA-adding enzymes to function as (U, G)-or dCdCdA-adding enzymes or poly (C, A) and poly (U, G) polymerases. Proc Natl Acad Sci U S A 2007; 104:54-9; PMID:17179213; http://dx.doi.org/10.1073/pnas.0606961104
- Trippe R, Guschina E, Hossbach M, Urlaub H, Lührmann R, Benecke B-J. Identification, cloning, and functional analysis of the human U6 snRNA-specific terminal uridylyl transferase. RNA 2006; 12:1494-504; PMID:16790842; http://dx.doi.org/10.1261/rna.87706
- Martin G, Keller W. RNA-specific ribonucleotidyl transferases. RNA 2007; 13:1834-49; PMID:17872511; http://dx.doi.org/10.1261/rna.652807
- Zamecnik PC, Raychowdhury MK, Tabatadze DR, Cantiello HF. Reversal of cystic fibrosis phenotype in a cultured Δ508 cystic fibrosis transmembrane conductance regulator cell line by oligonucleotide insertion. Proc Natl Acad Sci U S A 2004; 101:8150-5; PMID:15148387; http://dx.doi.org/10.1073/pnas.0401933101
- Exploratory Study to Evaluate QR-010 in Subjects With Cystic Fibrosis ΔF508 CFTR Mutation. (2015). Clinical Trial Phase I
- Dose Escalation Study of QR-010 in Homozygous ΔF508 Cystic Fibrosis Patients. (2015). Clinical Trial Phase IB
- Open Label, Extension Study of PRO044 in Duchenne Muscular Dystrophy (DMD). (2014). Clinical Trial Phase II
- Phase IIb Study of PRO045 in Subjects With Duchenne Muscular Dystrophy. (2013). Clinical Trial Phase 2B
- A Phase I/II Study of PRO053 in Subjects With Duchenne Muscular Dystrophy (DMD). (2013). Clinical Phase Trial I/II
- Study of SRP-4045 and SRP-4053 in DMD Patients (ESSENCE). (2015). Clinical Trial Phase III
- A Study to Assess the Safety and Tolerability of Single Doses of AZD4076 in Healthy Male Subjects. (2015). Clinical Trial Phase I
- RNA-Immunotherapy of IVAC_W_bre1_uID and IVAC_M_uID (TNBC-MERIT). (2014). Clinical Trial Phase I
- A Phase I Study of T-Cells Genetically Modified at the CCR5 Gene by Zinc Finger Nucleases SB-728mR in HIV-Infected Patients. (2015). Clinical Trial Phase I