ABSTRACT
Toxin-antitoxin systems (TA) are widespread in bacteria and archea. They are commonly found in chromosomes and mobile genetic elements. These systems move from different genomic locations and bacterial hosts through horizontal gene transfer, using mobile elements as vehicles. Their potential roles in bacterial physiology are still a matter of debate in the field. The mechanisms of action of different toxin families have been deciphered at the molecular level. Intriguingly, the vast majority of these toxins target protein synthesis. They use a variety of molecular mechanisms and inhibit nearly every step of the translation process. Recently, we have identified a novel toxin, AtaT, presenting acetyltransferase activity.Citation1 Our work uncovered the molecular activity of AtaT: it specifically acetylates the methionine moiety on the initiator Met-tRNAfMet. This modification drastically impairs recognition by initiation factor 2 (IF2), thereby inhibiting the initiation step of translation.
Background
Type II TA systems are commonly small operons encoding a stable toxic protein and an unstable antitoxin protein (for reviews,Citation2-4). These systems are widespread in bacterial and archeal genomes, i.e. chromosomes and mobile genetic elements such as plasmids, phages and conjugative transposons. They have literally invaded bacterial genomes via horizontal gene transfer,Citation5-7 some genomes encoding several dozen of them. The underlying reason for this striking evolutionary success remains enigmatic although several hypotheses have been proposed (for reviews,Citation8-11). Mobile genetic elements borne TA systems have the capacity to promote their stable maintenance in growing bacterial populations by eliminating bacterial progeny that did not receive a copy of the element during cell division. This mechanism relies on the differential stability of the antitoxin as compared to the toxin. In these cells, in absence of de novo synthesis of toxin and antitoxin, proteolysis of the antitoxin by cellular ATP-dependent proteases would liberate the toxin activity and lead to corruption of essential processes in the cell, thereby halting cell growth and eventually leading to cell death. These cells are therefore addicted to the presence of the TA system, they die if the system is lost.Citation12 This addictive property led to the idea that TA systems may be selfish elements, devoid of any particular function in the cell. However, body of data has shown that these systems might have other functions, notably they could serve as defense mechanisms against phage infection and plasmid establishment. The mainstream hypothesis regarding the function of chromosomally-encoded systems is their involvement in persistence to antibiotics. Activation of these systems would trigger a dormant, metabolically inactive physiological state that would allow bacteria to sustain antibiotic challenges or other types of stress. However, conflicting data have been recently publishedCitation13-15 and whether or not TA systems are main players in persistence is still under investigation.
Regarding the essential processes targeted, it is striking to observe that protein synthesis is the favorite target of type II toxins. Toxins use various molecular mechanisms to achieve their toxic functions and target nearly every step of translation (). Up to recently, 7 super-families of toxins have been characterized. Some toxins families have diverged significantly during evolution, remaining homologous only at the structural level allowing functional divergence (in activity and/or target specificity). In the CcdB/MazF family, CcdB-like toxins target DNA-gyraseCitation16 while MazF- like toxins cleave either free mRNAs,Citation17 23S rRNACitation18 or specific elongator tRNAs.Citation19 Such functional divergence is exemplified in most of the super-families. Toxins from the Fic family cleave high-energy nucleotides transferring to the target elements from either side of the scissile bond, which defines the two subgroups the family is divided. Thus, Doc-like toxins are kinases that phosphorylate the translation elongation factor EF-TuCitation20 whereas Fic-like toxins catalyze adenylylation of Gyrase and topoisomerase IV.Citation21 VapC toxins provide another interesting example. They are all RNA-endonucleases targeting a myriad of RNAs from initiator and elongator tRNAs to the 23S ribosomal RNA.Citation22-25
Figure 1. Type II TA toxins inhibit nearly every step of translation. Toxins blocking different steps of translation process are indicated in red, translation machinery components and assembly units are labeled in black. AtaT toxin acetylates Met-tRNAfMet and generates translation-incompatible acetylated Met-tRNAfMet that is not recognized by initiation factor IF2.Citation1 TacT acetylates elongation tRNAs on their amino acid blocking the free amine group necessary for peptide bond formation.Citation31 Different VapC toxins cleave either the anticodon loop of initiator tRNACitation22,25, the sarcin-ricin loop of 23S rRNACitation24 or different elongator tRNAs.Citation23 Different MazF toxin cleave either mRNAsCitation17, elongator tRNAsCitation19 or 23S rRNA.Citation18 RelE toxins cleave mRNAs that are being translated.Citation40 GraT inhibits ribosome biogenesis.Citation41 Doc phosphorylates elongation factor EF-Tu.Citation20 HipA phosphorylates Glutamyl-tRNA-synthetase.Citation42,43
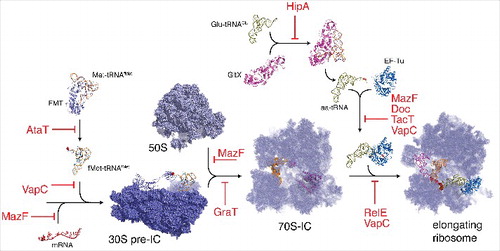
AtaT specifically acetylates the α-amino group of the methionine moiety charged on initiator tRNAfmet
Recently, we characterized a novel type II toxin presenting a GNAT-fold (GCN5-related N-acetyltransferases).Citation1 AtaT toxin is part of the ataRT TA system located in the E. coli O157:H7 enterohemorragic (EHEC) strain. We observed that expression of ataT in E. coli lab strain devoid of the system (MG1655) led to cell growth inhibition by blocking translation. Using an in vitro coupled transcription/translation system with purified E. coli translation components, we were able to show that acetyl-Coenzyme A (Ac-CoA) is necessary for the activity of AtaT. This was further confirmed by generating mutants in conserved residues located in the Ac-CoA binding pocket. Using radiolabelled Ac-CoA, we were then able to show that AtaT transfers specifically the acetyl group to the free amine group of the initiator tRNA charged with methionine. This modification impairs the interaction with initiation factor 2 (IF2) and the formation of the translation pre-initiation complex (30S pre-IC). In vivo, this is manifested by accumulation of 30S and 50S ribosomal subunits. We proposed that AtaT competes with L-methionyl-tRNAfMet-N-formyltransferase (FMT), thereby reducing strongly formylation. Moreover, since AtaT activity appears to be irreversible (see below), it is likely that acetylated Met-tRNAfMet constitutes a dead-end product that will accumulate with time and reduce the pool of initiator tRNA available for formylation.
Interestingly, AtaT is able to discriminate between initiator and elongator tRNAs, both carrying methionine amino acid. Initiator tRNA possess unique features distinct from those of elongator tRNAs. In bacteria and eukaryotic organelles that has been derived from bacteria (mitochondria and chloroplasts), the initiator tRNA is formylated by FMT after aminoacylation (for a review on translation initiation in bacteriaCitation26). Initiator tRNA binds to the P-site of the ribosome in the contrary to elongator tRNAs, which bind to the A-site and subsequently translocate to the P-site. Initiator tRNA is unable to bind to the A-site and cannot act as an elongator tRNA. This property is due to specific sequence/structural characteristics unique and conserved in all initiator tRNAs (). First, the absence of Watson-Crick base pairing between nucleotides C1 and A72 at the end of acceptor stem CCA prevents the use of initiator tRNAs as elongators and is also needed for formylation.Citation27 The presence of a purine 11:pyrimidine 24 base pair in the dihyrouridine stem instead of a pyrimidine 11:purine 24 in elongator tRNAS increases the efficiency of formylation.Citation28 Another important difference is the presence of 3 consecutive G:C pairs in the stem of the anticodon loop that are required for targeting initiator tRNAs to the P-siteCitation29 ().
Figure 2. Initiator fMet-tRNAfMet and elongator Met-tRNAMet. The secondary structure of tRNAs is represented in cloverleaf fashion highlighting the major sequence differences important for initiator tRNA identity during translation.
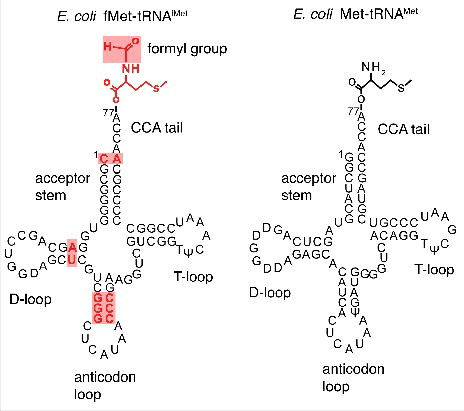
How AtaT selects specifically the initiator tRNA remains to be uncovered. Evidently the specific features described above could be crucial for AtaT recognition. As for FMT specific recognition, the absence of nucleotides 1:72 pairing might be an essential determinant, however AtaT is able to acetylate in vitro initiator tRNA even if the first nucleotide is substituted as well as base-paired (Our unpublished data). We also know that post-transcriptional modifications are not necessary for the activity of AtaT, since it is able to acetylate methionine on both in vitro-synthesized (unmodified) as well as in vivo-purified initiator tRNAs.Citation1 Besides the differences separating the initiator and elongator tRNAs described above, other differences notably at the primary sequence and/or structural levels could be the determinants for AtaT recognition.
AtaT and TacT define a novel GNAT family of type II toxins
In addition to AtaT, several TA systems encoding GNAT toxins have been recently identified.Citation1,30-33 These TA systems are located in bacterial chromosomes,Citation1,31,33 plasmidsCitation32 and gene cassettes from superintegrons.Citation30 The ataRT system is part of the accessory genome of E. coli EHEC strains and constitutes a small genomic islet in the O157:H7 strain.Citation34 Homologues are detected in other enterobacteria and on large plasmids from enteropathogenic bacteria.Citation34
These newly identified toxins define a novel super-family of GNAT toxins presenting unique acetyltransferase catalytic action, exemplified by the detailed molecular analysis of AtaTCitation1 and TacTCitation31 activities. The TacT toxin belongs to the TacAT system encoded in the chromosome of Samonella enterica serovar typhimurium and is involved in the intramacrophage persistence of this pathogen.Citation35 While TacT is a distant homologue of AtaT (24% identity at the amino acids sequence level), it presents the same catalytic activity as AtaT: it acetylates the free amine group of aminoacylated tRNAs. Major differences are however observed in terms of the aminoacyl tRNA specificity. AtaT is highly specific for initiator tRNA in contrast to TacT that presents a more relaxed specificity and is able to recognize and modify several elongator tRNAs.Citation31 The precise mechanism leading to inhibition of translation elongation by TacT remains to be uncovered although it is likely that acetylation prevents interaction of elongator tRNAs with the elongator factor EF-Tu, thereby preventing delivery to the ribosome A-site.Citation36 It will be interesting in the future to decipher the determinants of TacT specificity as well as the precise molecular mechanism leading to the inhibition of translation elongation. It is to be noted that the activity of AtaT and TacT are unique for GNAT enzymes. GNATs are conserved and widespread enzymes in all domains of life acetylating a large variety of substrates, from small molecules, antibiotics and proteins (for reviews,Citation37,38). Several GNAT families are involved in translation control by acetylating ribosomal proteins or tRNAs on nucleotides. Conversely GNAT-toxins recognize the CCA end of charged tRNAs suggesting that both the tRNAs part as well as the amino acyl moiety are recognized. Furthermore, their acetyltransferase activity precludes the use of the charged tRNAs in protein synthesis resulting in a novel mechanism of translation inhibition. Additional biochemistry and structural data will be required to understand precisely how these toxins recognize their substrates.
Rescuing cells from the action of type II GNAT toxins
In addition to differences in specificity, the action of TacT in Salmonella can be counteracted by several mechanisms, but this is not the case for AtaT (at least not by the same mechanisms). In a screen for multi-copy suppressors for TacT toxicity, Helaine and coworkers identified the pth gene encoding an essential peptidyl-tRNA hydrolase.Citation31 Pth hydrolyzes the ester bond between the carboxy-terminus of the nascent peptide chain and the 3′ hydroxyl of tRNA in case of abortive translation.Citation39 In vitro experiments showed that Pth releases acetylated amino acids from tRNA. Pth thus replenishes the pool of uncharged tRNAs and makes them readily available for acylation by the corresponding aminoacyl-tRNA synthetases. Another mechanism of reversibility involving the CobB non-specific deacetylase was identified in the same study, although the molecular bases appear less clear. The authors showed that the deletion of the cobB gene abolishes the dependency of TacT toxicity on bacterial growth phase and increased the activity of TacT. However, cobB overexpression did not counteract the toxicity of TacT.
In the case of AtaT, we were unable to detect any effect of Pth. Overexpression of Pth did not abolish toxicity of AtaT (). This is not surprising since the initiator Met-tRNAfMet is not a Pth substrate.Citation26 Base pairing at the stem of the acceptor domain appears to be crucial for Pth activity. Thus, the absence of base pairing between nucleotides C1 and A72 impairs Pth hydrolysis ().Citation26 As for TacT, AtaT toxicity is not reversed by CobB overexpression in vivo (Data not shown). Moreover, acetylation of Met-tRNAfMet by AtaT is not reversed in vitro by the AtaR antitoxin.Citation1
Figure 3. AtaT toxicity is not reversed by Pth overexpression. The ataT gene was cloned in the pBAD33 plasmid under the pBAD promoter control. The ataR and pth genes were cloned in the pKK223-3 plasmid under the pTac promoter control as previously described1. Overnight cultures of E. coli strain (DJ624Δara, a MG1655 derivative devoid of the ataT-ataR system) carrying combination of pBAD33 derivatives and pKK223-3 derivatives as indicated on the left panel were serially diluted as indicated above the panel and spotted on LB agar plates supplemented with 0.2% arabinose and 1 mM IPTG (left panel). After incubating the plates overnight at 37°C, colonies from the 10-3 dilution were collected and streaked on LB agar with 1% glucose to test viability (right panel).
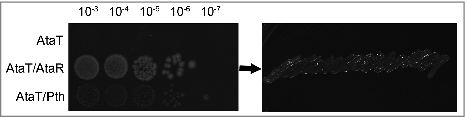
These differences in the way cells cope with the action of AtaT and TacT may indicate different functions. As mentioned above, TacT is activated upon the entry of Samonella into macrophages and leads to the differentiation as persister cells.Citation35 Detoxification of elongator tRNAs by Pth would allow TacT-induced persisters to resume growth. The cellular function of the AtaRT system has not been investigated. However, the fact that none of the detoxifying mechanisms identified for TacT appears to reverse the modification mediated by AtaT suggests that this system might not be involved in persistence.
Concluding remarks
Inhibiting translation from the start by interfering with the initiator tRNA is undoubtedly a very efficient way to halt cell growth. Some VapC toxins target initiator tRNA and cleave the anticodon stem loop.Citation22,25 Similarly by acetylating the methionine moiety of the initiator tRNA, AtaT generates dead-end products that will curtail the pool of initiator tRNA available for formylation by FMT.Citation1 These 2 toxins, by using different modes of action, alter initiator tRNA in an irreversible manner and thereby lead to efficient inhibition of the initiation step of the protein synthesis process.
Disclosure of potential conflicts of interest
No potential conflicts of interest were disclosed.
Acknowledgments
D.J. is a PhD fellow at FNRS (aspirant FNRS). Work in CM2 is supported by the Fonds National de la Recherche Scientifique (FNRS, grant number: T.0147.15F PDR and J.0061.16F CDR and F.4505.16 MIS), the Fonds d'Encouragement à la Recherche ULB (FER-ULB), the Interuniversity Attraction Poles Program initiated by the Belgian Science Policy Office (MICRODEV), Fonds Jean Brachet and Fondation Van Buuren.
References
- Jurenas D, Chatterjee S, Konijnenberg A, Sobott F, Droogmans L, Garcia-Pino A, Van Melderen L. AtaT blocks translation initiation by N--acetylation of the initiator tRNAfMet. Nat Chem Biol. 2017;13:640–6. doi:10.1038/nchembio.2346.
- Hayes F, Van Melderen L. Toxins--antitoxins: diversity, evolution and function. Crit Rev Biochem Mol Biol. 2011;46:386–408. doi:10.3109/10409238.2011.600437.
- Page R, Peti W. Toxin--antitoxin systems in bacterial growth arrest and persistence. Nat Chem Biol. 2016;12:208–14. doi:10.1038/nchembio.2044.
- Goeders N, Van Melderen L. Toxin--antitoxin systems as multilevel interaction systems. Toxins (Basel). 2014;6:304–24. doi:10.3390/toxins6010304.
- Leplae R, Geeraerts D, Hallez R, Guglielmini J, Dreze P, Van Melderen L. Diversity of bacterial type II toxin--antitoxin systems: a comprehensive search and functional analysis of novel families. Nucleic Acids Res. 2011;39:5513–25. doi:10.1093/nar/gkr131.
- Ramisetty BC, Santhosh RS. Horizontal gene transfer of chromosomal Type II toxin--antitoxin systems of Escherichia coli. FEMS Microbiol Lett. 2016;363.
- Pandey DP, Gerdes K. Toxin--antitoxin loci are highly abundant in free--living but lost from host--associated prokaryotes. Nucleic Acids Res. 2005;33:966–76. doi:10.1093/nar/gki201.
- Van Melderen L. Toxin--antitoxin systems: why so many, what for? Curr Opin Microbiol. 2010;13:781–5. doi:10.1016/j.mib.2010.10.006.
- Van Melderen L, Saavedra De Bast M. Bacterial toxin--antitoxin systems: more than selfish entities? PLoS Genet. 2009;5:e1000437. doi:10.1371/journal.pgen.1000437.
- Harms A, Maisonneuve E, Gerdes K. Mechanisms of bacterial persistence during stress and antibiotic exposure. Science. 2016;354. doi:10.1126/science.aaf4268.
- Magnuson RD. Hypothetical functions of toxin--antitoxin systems. J Bacteriol. 2007;189:6089–92. doi:10.1128/JB.00958-07.
- Yarmolinski MB. Programmed cell death in bacterial populations. Science. 1995;267:836–7. doi:10.1126/science.7846528.
- Shan Y, Brown Gandt A, Rowe SE, Deisinger JP, Conlon BP, Lewis K. ATP-- Dependent Persister Formation in Escherichia coli. MBio. 2017;8. pii:e02267–16. doi:10.1128/mBio.02267-16.
- Ramisetty BC, Ghosh D, Roy Chowdhury M, Santhosh RS. What Is the Link between Stringent Response, Endoribonuclease Encoding Type II Toxin--Antitoxin Systems and Persistence? Front Microbiol. 2016;7:1882. doi:10.3389/fmicb.2016.01882.
- Van Melderen L, Wood TK. Commentary: What Is the Link between Stringent Response, Endoribonuclease Encoding Type II Toxin--Antitoxin Systems and Persistence? Front Microbiol. 2017;8:191. doi:10.3389/fmicb.2017.00191.
- Bernard P, Couturier M. Cell killing by the F plasmid CcdB protein involves poisoning of DNA--topoisomerase II complexes. J Mol Biol. 1992;226:735–45. doi:10.1016/0022-2836(92)90629-X.
- Zhang Y, Zhang J, Hoeflich KP, Ikura M, Qing G, Inouye M. MazF cleaves cellular mRNAs specifically at ACA to block protein synthesis in Escherichia coli. Mol Cell. 2003;12:913–23. doi:10.1016/S1097-2765(03)00402-7.
- Schifano JM, Edifor R, Sharp JD, Ouyang M, Konkimalla A, Husson RN, Woychik NA. Mycobacterial toxin MazF--mt6 inhibits translation through cleavage of 23S rRNA at the ribosomal A site. Proc Natl Acad Sci U S A. 2013;110:8501–6. doi:10.1073/pnas.1222031110.
- Schifano JM, Cruz JW, Vvedenskaya IO, Edifor R, Ouyang M, Husson RN, Nickels BE, Woychik NA. tRNA is a new target for cleavage by a MazF toxin. Nucleic Acids Res. 2016;44:1256–70. doi:10.1093/nar/gkv1370.
- Castro-Roa D, Garcia-Pino A, De Gieter S, van Nuland NAJ, Loris R, Zenkin N. The Fic protein Doc uses an inverted substrate to phosphorylate and inactivate EF--Tu. Nat Chem Biol. 2013;9:811–7. doi:10.1038/nchembio.1364.
- Harms A, Stanger FV, Scheu PD, de Jong IG, Goepfert A, Glatter T, Gerdes K, Schirmer T, Dehio C. Adenylylation of Gyrase and Topo IV by FicT Toxins Disrupts Bacterial DNA Topology. Cell Rep. 2015;12:1497–507. doi:10.1016/j.celrep.2015.07.056.
- Lopes AP, Lopes LM, Fraga TR, Chura-Chambi RM, Sanson AL, Cheng E, Nakajima E, Morganti L, Martins EA. VapC from the leptospiral VapBC toxin--antitoxin module displays ribonuclease activity on the initiator tRNA. PLoS One. 2014;9:e101678. doi:10.1371/journal.pone.0101678.
- Winther K, Tree JJ, Tollervey D, Gerdes K. VapCs of Mycobacterium tuberculosis cleave RNAs essential for translation. Nucleic Acids Res. 2016;44:9860–71. doi:10.1093/nar/gkw781.
- Winther KS, Brodersen DE, Brown AK, Gerdes K. VapC20 of Mycobacterium tuberculosis cleaves the sarcin--ricin loop of 23S rRNA. Nat Commun. 2013;4:2796. doi:10.1038/ncomms3796.
- Winther KS, Gerdes K. Enteric virulence associated protein VapC inhibits translation by cleavage of initiator tRNA. Proc Natl Acad Sci U S A. 2011;108:7403–7. doi:10.1073/pnas.1019587108.
- Laursen BS, Sorensen HP, Mortensen KK, Sperling-Petersen HU. Initiation of protein synthesis in bacteria. Microbiol Mol Biol Rev. 2005;69:101–23. doi:10.1128/MMBR.69.1.101-123.2005.
- Seong BL, RajBhandary UL. Mutants of Escherichia coli formylmethionine tRNA: a single base change enables initiator tRNA to act as an elongator in vitro. Proc Natl Acad Sci U S A. 1987;84:8859–63. doi:10.1073/pnas.84.24.8859.
- Varshney U, Lee CP, RajBhandary UL. From elongator tRNA to initiator tRNA. Proc Natl Acad Sci U S A. 1993;90:2305–9. doi:10.1073/pnas.90.6.2305.
- Seong BL, RajBhandary UL. Escherichia coli formylmethionine tRNA: mutations in GGGCCC sequence conserved in anticodon stem of initiator tRNAs affect initiation of protein synthesis and conformation of anticodon loop. Proc Natl Acad Sci U S A. 1987; 84:334–8. doi:10.1073/pnas.84.2.334.
- Iqbal N, Guerout AM, Krin E, Le Roux F, Mazel D. Comprehensive Functional Analysis of the 18 Vibrio cholerae N16961 Toxin--Antitoxin Systems Substantiates Their Role in Stabilizing the Superintegron. J Bacteriol. 2015;197:2150–9. doi:10.1128/JB.00108-15.
- Cheverton AM, Gollan B, Przydacz M, Wong CT, Mylona A, Hare SA, Helaine S. A Salmonella Toxin Promotes Persister Formation through Acetylation of tRNA. Mol Cell. 2016;63:86–96. doi:10.1016/j.molcel.2016.05.002.
- McVicker G, Tang CM. Deletion of toxin--antitoxin systems in the evolution of Shigella sonnei as a host--adapted pathogen. Nat Microbiol. 2016;2:16204. doi:10.1038/nmicrobiol.2016.204.
- Van Acker H, Sass A, Dhondt I, Nelis HJ, Coenye T. Involvement of toxin--antitoxin modules in Burkholderia cenocepacia biofilm persistence. Pathog Dis. 2014;71:326–35. doi:10.1111/2049-632X.12177.
- Jurenas D, Garcia-Pino A, Van Melderen L. Novel toxins from type II toxin-- antitoxin systems with acetyltransferase activity. Plasmid. 2017;93:30–5. doi:10.1016/j.plasmid.2017.08.005.
- Helaine S, Cheverton AM, Watson KG, Faure LM, Matthews SA, Holden DW. Internalization of Salmonella by macrophages induces formation of nonreplicating persisters. Science. 2014;343:204–8. doi:10.1126/science.1244705.
- Janiak F, Dell VA, Abrahamson JK, Watson BS, Miller DL, Johnson AE. Fluorescence characterization of the interaction of various transfer RNA species with elongation factor Tu.GTP: evidence for a new functional role for elongation factor Tu in protein biosynthesis. Biochemistry. 1990;29:4268–77. doi:10.1021/bi00470a002.
- Favrot L, Blanchard JS, Vergnolle O. Bacterial GCN5--Related N--Acetyltransferases: From Resistance to Regulation. Biochemistry. 2016;55:989–1002. doi:10.1021/acs.biochem.5b01269.
- Salah Ud-Din AI, Tikhomirova A, Roujeinikova A. Structure and Functional Diversity of GCN5--Related N--Acetyltransferases (GNAT). Int J Mol Sci. 2016;17. doi:10.3390/ijms17071018.
- Sharma S, Kaushik S, Sinha M, Kushwaha GS, Singh A, Sikarwar J, Chaudhary A, Gupta A, Kaur P, Singh TP. Structural and functional insights into peptidyl--tRNA hydrolase. Biochim Biophys Acta. 2014;1844:1279–88. doi:10.1016/j.bbapap.2014.04.012.
- Pedersen K, Zavialov AV, Pavlov MY, Elf J, Gerdes K, Ehrenberg M. The bacterial toxin RelE displays codon--specific cleavage of mRNAs in the ribosomal A site. Cell. 2003;112:131–40. doi:10.1016/S0092-8674(02)01248-5.
- Ainelo A, Tamman H, Leppik M, Remme J, Horak R. The toxin GraT inhibits ribosome biogenesis. Mol Microbiol. 2016;100:719–34. doi:10.1111/mmi.13344.
- Germain E, Castro-Roa D, Zenkin N, Gerdes K. Molecular mechanism of bacterial persistence by HipA. Mol Cell. 2013;52:248–54. doi:10.1016/j.molcel.2013.08.045.
- Kaspy I, Rotem E, Weiss N, Ronin I, Balaban NQ, Glaser G. HipA-mediated antibiotic persistence via phosphorylation of the glutamyl--tRNA--synthetase. Nat Commun. 2013;4:3001. doi:10.1038/ncomms4001.