Abstract
Season of birth correlates with approximately 10-fold increased risk of early mortality from infectious disease in parts of The Gambia, West Africa. The increased early death occurred in individuals aged 25–50, who were born during the nonharvest season when stored groundnuts constituted a significant portion of the diet. Insect pests destroy stored groundnuts if not prevented, thus, the people mix insecticides into the storage bags before sealing of the bags. This procedure has been used since the 1970s or earlier, with the specific insecticide agents varying by availability. Organochlorine insecticides including DDT, lindane, and chlordane were added to bags until as recently as the 1980s. Subsequently organophosphates and pyrethroids became available and largely replaced the organochlorine agents. These agents both cross the placenta and are secreted into milk. Further, all are recognized immunotoxicants. These collective observations suggest the possibility that perinatal immunotoxic pesticide exposure, in individuals born during nonharvest months, may impair development of the immune system and increase risk of early death from infectious disease. Additional studies are planned to investigate our hypothesis that pesticide-related developmental immuntoxicity may contribute, in part, to Gambian seasonal mortality.
INTRODUCTION
We previously published survival data from rural Gambia, which showed that mortality from infectious diseases among young adults was strongly correlated with the season of their birth (Moore et al., Citation1999). Specifically, individuals born during the annual rainy (‘hungry’) season (July to December) had a 10-fold greater chance of dying in early adulthood than their counterparts born during the dry harvest season ( and ). We hypothesized that an early life insult, correlating with season of birth, caused permanent damage to the developing immune system, resulting in the premature deaths. Possible candidate insults included maternal undernutrition and fetal growth restriction, exposure to highly seasonal infections affecting the infant or pregnant mother, or exposure to aflatoxin on stored foods (Turner et al., Citation2003).
FIG. 1Kaplan-Meier survival plots by season of birth. Data are for 1103 deaths from 3,162 births (Jan–Jun); lower (solid) line = hungry season births (Jul–Dec). (Reproduced with permission from the International Journal of Epidemiology)
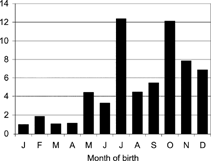
FIG. 2Seasonal distribution of births among premature adult deaths. Data are expressed as % of monthly births to adjust for seasonal differences in birth rate. (Reproduced with permission from the International Journal of Epidemiology)
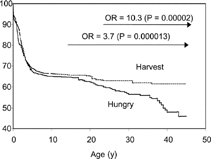
Agricultural activity and the resultant food supply are of a bimodal pattern in many areas along the southern edge of the Sahara, with the year being divided into the rainy season when planting and cultivation occur (July–October in The Gambia), and the dry season when crops are harvested. The harvest season starts at the end of the year, and food, which is in relative plenty at this time, is stored for consumption during the next planting season. The subtropical conditions make for an abundance of insect pests that attack stored crops. The availability of food for consumption in the next planting season, and hence for survival, has always been critically dependent on effective storage and protection of the previous year's produce from insect pests. The introduction of modern pesticides was an effective solution to the insect problem, and assured food supply in the hungry months, but this may have come at a cost to a people not educated enough to understand the full implications of their use.
We recently found that, like early mortality, thymus size in Gambian infants is affected by birth season, and is reduced in hungry season infants (Collinson et al., Citation2003). The diminished thymus size did not correlate with infection or nutritional status. In the current paper, we review pesticide use and the immunotoxicity of agents that have been preferentially utilized for food preservation in The Gambia. These collective reports suggest the possibility that perinatal exposure to immunotoxic pesticides might disrupt infant immune development, resulting in lifelong impaired immune function. We therefore propose that early pesticide exposure in The Gambia may offer an alternative etiological hypothesis to explain the profound season-of-birth effect on adult mortality.
Pesticide Use in Rural Gambia
We obtained retrospective information on pesticide usage within Keneba, Kantong Kunda and Manduar; the same three villages in which we had observed that season-of-birth predicts premature adult mortality. These are rural, subsistence-farming communities whose pattern of life remained relatively unchanged over the latter half of the last century. Information on the farming system and food storage methods practiced locally was obtained by interviews with the most active farmers and elderly residents from the community. Rice (Oryza sativa) and groundnuts (peanuts; Arachis hypogaea) constitute the staple diet of the people, and are cultivated by most families for food. In more recent times, some imported rice has been purchased by smaller farmers to supplement their farm produce, but all groundnuts consumed are locally produced. Other crops grown are maize (Zea mays), millet (Pennisetum typhoideum), sorghum (Sorghum margaritiferum), findo (Digitaria exilis), cassava (Manihot esculenta), and sweet potatoes (Ipomoea batatas).
Farmers use insecticides both on the crops in the fields, and on the harvested farm produce. For rice, millet and sorghum, insecticides typically are applied when the crops start flowering. This is the period when insects are attracted to the forming seeds. After the seeds have been harvested, pesticides are used on some of the produce. The grains: rice, maize, millet and sorghums, are usually processed by milling or removing the husks after which they are stored and gradually consumed or sold. The remainder are treated with pesticides and preserved for use as seeds in the next planting season.
Groundnuts present a special problem to the farmers, as they may be infested with insect pests from the time they are harvested in the months of October/November. The groundnuts that will be consumed in the short term are generally processed and stored without pesticide application. When longer storage is required, an insecticide is applied on the nuts to preserve them for use as seeds and also for consumption in the next planting season. The pyrethroid insecticide cypermethrin has been widely used in recent years, in a powder formulation. Generous quantities are poured into the groundnut bags, after which the bags are shaken to ensure an even distribution, then sealed and stored. Organophosphates (OP; e.g., chlorpyrifos, malathion) have also been used as these agents were available. From the 1940s to 1980s, organochlorine (OC) insecticides including 1,1,1-trichloro-2.2-bis(p-chlorophenyl)ethane (DDT), hexachlorocyclohexane (lindane), and the cyclodiene insecticide chlordane were used, with DDT predominating. It is universally confirmed by the locals that without the pesticides, groundnuts invariably get destroyed by the time the rains approach. The population is an illiterate one, and there is no regulation or restriction of use of the available pesticides. Bigger farmers with excess produce offer them for sale to people who buy them for consumption, after running out of supplies in the hungry season.
The OC insecticides used in the past to preserve groundnuts were stable in the storage environment. The presently often-used cypermethrin is a light-stable type-II synthetic pyrethroid insecticide (Hayes and Laws, Citation1990). In the dark and enclosed conditions of groundnut storage, its half-life can extend to months (USEPA, Citation1989a). The cypermethrin whitish powder can be seen coating the seed groundnuts when stored bags are opened in the months of June/July. Shelling is then usually done by hand by the women, such that their hands get covered with and transfer insecticide to groundnuts being picked out. The high oil content of groundnuts would be expected to promote accumulation of lipophilic insecticides during this process, including DDT and cypermethrin. The groundnut shells are next separated by winnowing in the wind, after which the seeds are sorted with the better seeds reserved for planting and the remainder pounded into a paste for preparation of groundnut sauce (tia durango or domoda), which is eaten with rice as the staple. The winnowing process also suspends the powder as dust that may be inhaled by the women and their children who are never far away. Hands are usually not washed after the process, so transdermal absorption, mouthing (especially in children) and contamination of other food preparations are possible and may constitute additional routes of exposure. In this way, there has been an ongoing seasonal (hungry season) exposure to pesticides, mostly through the oily groundnuts that were stored in pesticide-laced bags.
This same system of crop and seed protection has been in use since these chemicals became available as conventional pesticides here. Prior to their introduction, naturally occurring substances including the powder from the crushed bark of certain trees and various roots were used.
Effects of Pesticides Previously Used in The Gambia on the Immune System
The major classes of pesticides known to have been used in The Gambia, OC, OP, and increasingly pyrethroid, have toxic effects on the immune system both in laboratory animals and humans. DDT reduced the proliferative response of beluga whale splenocytes cultured either with or without the mitogen, phytohemagglutinin A (PHA) (De Guise et al., Citation1998), suppressed humoral and cell mediated immune (CMI) responses in rats (Banerjee et al., Citation1996), and reduced antibody production in BALB/c mice (Wiltrout et al., Citation1978). Nunez et al. (Citation2002) found that DDT inhibited activation of mouse macrophages and decreased resistance of exposed animals to infection by Mycobacterium microti. Decreased B-cell responses to lipopolysaccharide (LPS) and increased incidence of trematode infection in the marine toad (Bufo marinus) were also associated with high levels of DDT (Linzey et al., Citation2003). High levels of persistent pesticide mixtures that included DDT and its metabolite dichlorodiphenyl- dichloro-ethylene (DDE), in dolphins in the Mediterranean, the North Sea and the North Atlantic, were suspected in mass mortality from common viruses to which the animals were normally resistant (Aguilar and Raga, Citation1993).
Almost no published data are available to assess the developmental immunotoxicity potential of DDT. In one report, Milston et al. (Citation2003) demonstrated long-lasting immune suppression in Chinook salmon following DDE exposure during early life-history stages. Eggs were immersed in 10 ppm DDE for 1 hour at fertilization and fry were immersed in the same concentration for 2 hours at hatching. At 1 year of age, the fish displayed significant reduction in ability of splenic leukocytes to proliferate upon stimulation with LPS and reduced splenic leukocyte surface immunoglobulin M (SIgM) expression. The authors noted that mortality rate, time to hatch, fish length, and weight were unaffected by the DDE treatment. These results suggest exposure to DDT in early life may cause immunologic damage that persists to maturity, in the absence of overt toxicity.
Human immunotoxicity has also been demonstrated in populations inadvertently or occupationally exposed to pesticide mixtures that included DDT. Levels of serum IgG decreased with increasing DDE levels in a relatively highly exposed population of farmers in the United States (Cooper et al., Citation2004). Decreased TH1 cytokines, such as IL-2 and interferon-gamma (IFN-γ), were associated with increasing levels of DDT metabolites in farmers and farmhands in the former German Democratic Republic (Daniel et al., Citation2002). Indian farmers heavily exposed to DDT and other pesticides had elevated relative rates of immune deficiency-associated cancers (Repetto and Baliga, Citation1996). In Central Luzon in the Philippines, researchers found a clear association between exposure to pesticide mixtures that included DDT, and mortality rates: mortality rates rose among adult male farmers exposed to pesticides in small-holder rice farming and simultaneously fell for adult females in the same villages and for adult males living in nearby towns (Loevinsohn, Citation1987). Seasonal peaks in mortality among these farmers coincided with periods of intensive pesticide application. Thus, available data clearly indicate that DDT is an immunotoxic pesticide.
Selective and highly persistent immune suppression have also been observed in mice after gestational exposure to the organochlorine cyclodiene insecticide, chlordane (Spyker-Cranmer et al., Citation1982). Mice exposed to chlordane during fetal life displayed significant depression of cell-mediated immunity that is still present 101 days after birth. In addition, a reduced number of granulocyte-macrophage colony-forming units and colony-forming units in the spleen is observed at 200 days of age, and also a long-term depression of both delayed-type hypersensitivity (DTH) and mixed lymphocyte reactivity (MLR) (Barnett et al., Citation1987). Chlordane exposure during development also reduced the number of pro-B-cells in late gestation, and B-cells detectable in the CFU-B assay in postnatal animals (Barnett et al., Citation2005). To be noted is that these apparently permanent developmental immune defects are either reduced or absent in adult mice exposed to chlordane at dose levels equal to those given to the pregnant mice. It remains uncertain if similar developmental immunotoxicity occurs in humans after in utero chlordane exposure. Inuit infants have been exposed to chlordane during gestation and nursing, and displayed postnatal immunosuppression (Dewailly et al., Citation1993a, Citation1993b; Mulvad et al., Citation1996, 2002). However, chlordane was not the only OC immunotoxicant to which these infants were exposed; thus, the contribution of chlordane to the reported pathology remains uncertain.
Pyrethroid insecticides are among the more recent and widely used agents that have replaced persistent OC insecticides such as DDT. The immunotoxicity database in laboratory animals or humans exposed to these agents is quite limited; however, available reports clearly suggest the potential for immunotoxicity. Suppression of cytotoxic activity of T-lymphocytes in BALB/c mice exposed to permethrin (a type-I pyrethroid) was demonstrated by Blaylock et al. (Citation1995). Enan et al. (Citation1996) found that deltamethrin (a type-II pyrethroid) produced atrophy of the thymus of BALB/c mice in a dose- and time-dependent fashion. Punareewattana et al. (2000, 2001) exposed mice to topical permethrin, and observed decreased thymic weight, increased splenic weight, inhibited macrophage function, and reduced antibody production. Prater et al. (Citation2005) reported persistent, dose-related inhibition of the contact hypersensitivity response in mice after topical exposure to permethrin. Desi et al. (Citation1985) described suppressive effects of orally-administered cypermethrin on both humoral and cellular immunological responses in rats and rabbits. Humans exposed to pyrethroids through correctly performed pest control applications also displayed limited but significant immunologic changes, including reduced serum immunoglobulins IgM, IgA and IgG and complement components C3c and C4, and decreased numbers of CD3+, CD4+ and CD20+ serum leukocytes (Hadnagy et al., Citation2003).
Finally, a limited but increasing body of literature associates the OP insecticides with immunotoxicity. Japanese medaka (Oryzias latipes) exposed to malathion in the water displayed dose-dependent inhibition of antibody production and increased death after bacterial challenge (Beaman et al., Citation1999). Diazinon exposure in C57Bl/6 mice caused histopathologic changes in thymus and spleen, reduced thymus to body weight ratio, depressed antibody production, and diminished delayed-type hypersensitivity responses (Neishabouri et al., Citation2004). Rats dosed with chlorpyrifos showed impaired T-cell proliferation and reduced T-cell-dependent antibody production (Blakley et al., Citation1999). In humans, chlorpyrifos exposure has been associated with increased atopy and antibiotic sensitivity, elevated CD26+ cells, and a higher rate of autoimmune disease (Thrasher et al., Citation1993, Citation2002).
Galloway and Handy (Citation2003) recently provided a review of mechanisms underlying OP immunotoxicity, including inhibition of serum hydrolases or esterases in immune cells, oxidative damage to immune organs, and modulation of signal transduction pathways that control immune function. These authors described associated immune lesions that have been reported, including pathology of immune organs, decreased humoral and cell-mediated immune responses, altered non-specific immunity, decreased host resistance, and increased hypersensitivity and autoimmunity disorders. In one report that considered developmental exposure, rats treated with chlorpyrifos on postnatal days 1–4 or 11–14 displayed impaired T-cell responses into adulthood (Navarro et al., Citation2001). This single developmental report suggests long-term immunologic deficits may be caused by early chlropyrifos exposure.
Thus, there is good evidence that the pesticides preferentially used in Gambia for preservation of food are all immunotoxicants. There is also ample evidence from experimental studies that indicates adverse effects on the developing immune system after in utero or postnatal exposure to immunotoxic chemicals or drugs (for reviews, see Holladay and Luster Citation1994; Holladay and Smialowicz, Citation2000). The resultant inhibited postnatal immune responses in such animals are often more dramatic and persistent than those occurring after exposure during adult life (Faith and Moore, Citation1977; Spyker-Cranmer et al., Citation1982; Holladay et al., Citation1991; Gehrs and Smialowicz, Citation1999; Barnett et al., Citation2005) and usually occur at lower doses (Faith and Moore, Citation1977; Weber and Birnbaum, Citation1985; Fine et al., Citation1989; Choi et al., Citation2004; Hrubec et al., Citation2005). These collective reports suggest consideration of the possibility that perinatal exposure to immunotoxic pesticides in The Gambia might explain, at least in part, the 10-fold increased later-life mortality from infectious disease.
Possible Causal Relationship Between Early Pesticide Exposure and Premature Mortality
The time frame of early-life pesticide exposure in rural Gambians must be considered relative to increased early mortality. The population affected was born from the late 1940s to the 1970s and 80s, with increased early mortality being a continuing problem (Moore et al., Citation1999). Obtaining accurate pesticide usage information is not possible due to poor record-keeping; however, old farmers confirm by interview the use of pesticides as far back as they can remember and generally in the same way as present day use. The noteworthy difference over time during the lives of the study population has been the specific substances used. As recently as a decade ago, cypermethrine, chlorpyriphos and carbosulphan became the commonly-used pesticides. Farmers purchase these agents as needed in the open market. Many farmers claim that decades ago, the pesticides were much more powerful and effective at controlling insect pests. This was at a time when the state agriculture department routinely supplied farmers with OC pesticides, including DDT, which is banned in most developed countries.
Studies were recently performed in Keneba and nearby villages to test our initial hypothesis that perinatal malnutrition exposure may relate to increased early mortality. We investigated the immune function of 6–8-year-old children according to their birth weight, season of birth, and whether or not their mothers had benefited from dietary supplementation during pregnancy (Moore et al., Citation2001). The study found no consistent associations between any of the three experimental variables and a broad panel of immunological outcomes. Although such was not the intent of the experiments, these results may suggest that perinatal exposure to more recent pesticides, including cypermethrin, do not cause developmental immunotoxicity. Alternatively, it should be considered that perinatal exposure to some immunotoxicants may imprint changes in immune cells that do not express until after puberty or after later-life immunologic challenges. As an example, Karpuzoglu-Sahin et al. (Citation2001) found no detectable immune dysfunction in adult mice that had received a single 10 g/kg dose of diethylstilbestrol (DES) during gestation. However, if the in utero DES-exposed mice received a second low-level exposure to DES as late as 12–18 months of age, it became apparent that T-cell cytokine production was dysregulated in the mice. In that the excess early mortality in The Gambia was observed in individuals between ages 25 and 50, a similar later-life manifestation of an imprinted changed immune responsiveness, from early damage to the immune system, may be possible. The age of the increased mortality population is also largely but not completely consistent with the time of OC pesticide use, thus these agents may have greatest potential to cause developmental immunotoxicity associated with increased later infectious disease-related mortality.
Potential Trans-Placental and Lactational Exposure of Gambian Infants to Pesticides
Available laboratory rodent data show that transplacental exposure to a growing list of immunotoxicants can cause permanent damage to the developing immune system, with lifelong postnatal immune suppression as a consequence (reviewed by Holladay and Smialowicz, Citation2000). Essentially no human data are presently available regarding the possibility for similar postnatal immune consequences as a result of gestational exposure to individual or mixtures of immunotoxic pesticides. However, recent increased use of therapeutic immunosuppressants during human pregnancy has been accompanied by reports of transient to long-lasting (i.e., postpubertal) changes in immune responses in offspring, supporting the rodent data (reviewed by Gogal and Holladay, Citation2005).
The immunotoxic OC pesiticides DDT and lindane, that have seen heavy use by Gambian farmers, cross both the rodent (You et al., Citation1999) and human placenta (Saxena et al., Citation1980, Citation1981; Siddiqui et al., Citation1981). Chlordane crosses the rat placenta, however placental transfer data are lacking for humans (Barnett et al., Citation2005). Human placental transfer data are again very limited for OP pesticides and pyrethroid pesticides. The OP pesticide chlorpyrifos has been found to cross the rat placenta (Abdel-Rahmann et al., Citation2002), while parathion crossed the human placenta in in vitro perfusion studies (Benjaminov et al., Citation1992). Pyrethroids, including permethrin, deltamethrin, and cyhalothrin cause limited developmental teratogenic effects, however it is not known if these are due to direct (transplacental) or indirect effects (Spencer and Berhane, Citation1982; Gomes et al., Citation1991; Abdel-Khalik et al., Citation1993). More recent data indicate detectable transfer of permethrin across the human placenta (CitationATSDR, 2003).
Persistent lipophilic OC pesticides and their residues are also secreted into human milk, including p,p′-DDT, o,p′-DDE, and p,p′-DDE (Galetin-Smith et al., Citation1990), chlordane and lindane (Kunisue et al., Citation2004). Organophosphates have been detected in human milk (Kacew, Citation1994) while pyrethroids have been detected in the milk of treated goats (Ivie and Hunt, Citation1980), dairy cattle (Bissacot and Vassilie, Citation1997; Sassine et al., Citation2004) and humans (Bonyata, Citation2002). Thus, the OC, OP and pyrethroid pesticides that have seen greatest use for food preservation in The Gambia, at least to some degree, both cross the placenta and are secreted into milk. Data that quantify OP and pyrethroid trans-placental and lactational transfer are not available, however it seems likely that the more lipophilic OC pesticides would see the greatest transfer of maternal dose to the fetus/infant by either route.
Potential immune system damage as a result of breast milk transfer of OC pollutants to infants has been suggested by findings among Inuit Indians whose diet of animal blubber contained organochlorine compounds. Inuit children in the Hudson Bay, Canada, area were found to be 30 times more likely to suffer from meningitis than U.S. children (Proulx, Citation1988). Infections of the inner ear were epidemic among similar Inuit children in Northern Quebec, resulting in high rates of hearing impairments. Children who were breast-fed were more likely to suffer from acute ear infections than bottle-fed babies. Dewailly et al. (Citation1993a) hypothesized that the organochlorines passed in breast milk to infants caused the increased susceptibility to acute otitis media and other infectious diseases. The breast milk-fed babies showed other signs of immunological deficiency, including failure to produce a protective primary antibody response to the usual vaccines, and decreased T-helper/T-suppressor cell ratios (Lindstrom et al., Citation1995). In one study, alterations in lymphocyte subset ratios and increased incidence of acute otitis media were directly associated with duration of breast-feeding and organochlorine level in milk (Julien et al., Citation1987). In the early 1990s, the DDE and polychlorinated biphenyl concentrations in Inuit breast milk were four times higher, and ten times higher for mirex, than for women from control groups (Dewailly et al., Citation1993b).
It therefore seems possible that transplacental and/or breast milk exposure to immunosuppressive pesticides may, at least in part, explain our recent observation of seasonal variation in Gambian children's thymic size (Collinson et al., Citation2003). In particular, we found that the mean thymic index, determined by ultrasound by a trained and blinded technician, was significantly lower in hungry season children. The reduced thymic size effect persisted after adjusting for body weight and current infection markers, and was strongest at 8 weeks postpartum (p = 0.001). In agreement with a possible pesticide-related etiology, reduced thymic size was not correlated to seasonal differences in nutritional status, or markers of infection. The children studied were of an age such that limited OC exposure would be predicted, with cypermethrin likely predominating. As previously mentioned, one of the more consistent findings in animal studies that have examined the immunotoxicity of pyrethroids is thymic atrophy (Rashatwar and Matsumura, Citation1985; Enan et al., Citation1996; Madsen et al., Citation1996; Punareewattana et al., Citation2000). At least for the thymic size study, a transplacental contribution to immunotoxicity may have been limited; thymic index measurements in week-old infants were arithmetically but nonsignificantly lower in hungry season births (p = 0.06). However transplacental exposure in the predelivery period should still be considered for the total spectrum of postnatal immune suppression from current pyrethroid use or insecticide mixtures of organophosphates and pyrethroids. A contribution of late gestation exposure fits the early mortality time line, as the months included in the early death syndrome extend somewhat beyond the harvest period ().
Another interesting factor, especially with respect to seasonal calorie deprivation, is the accumulation of pesticides in body fat. Researchers who assayed blood samples from live bottlenose dolphins captured off the Florida coast determined that their immune function had been compromised by high levels of organochlorine contaminants (Lahvis et al., Citation1995). In these dolphins, decreased immune response was highly correlated with increased levels of bioaccumulated organochlorines, and infections they exhibited were also suggestive of immune dysfunction. The organochlorine contaminants stored in the blubber may have been mobilized during periods of sickness and reduced dietary intake, causing further immunosuppression (Lahvis et al., Citation1993, Citation1995). This is not an isolated observation of fat mobilization as a source of increased exposure to DDT, as indeed studies indicate that exposed bats may be affected by DDT released from stored body fat during long migratory periods (USEPA, Citation1989b). Also, it is known that when fat stores are used during periods of starvation, the breakdown products of DDT are released into the blood where they may be toxic to the liver and the nervous system (Klaassen et al., Citation1996). Implications for the Gambian setting are that the maternal negative energy balance and consequent fat mobilization in the hungry season probably increase the exposure of fetus and newborn to higher quantities transplacental or transmammary toxicants.
Conclusion
Infectious diseases whose effects are worsened by malnutrition, especially in children who are the most vulnerable population, account for most deaths in the developing world. Malnutrition and susceptibility to xenobiotic toxicity are also intimately linked. The association of dietary contaminants with immunotoxicity has been demonstrated in adult human studies with different pesticide groups (Lee et al., Citation1964; Banerjee et al., Citation1995), and adds to the list of potential contributory factors to hungry season toxicity in seasonally affected populations such as The Gambia. The serendipitous discovery of these seasonally-predicted mortality patterns emerged from the Keneba Medical Research Council because this is one of the few research facilities in rural Africa where long-term clinical and demographic records are maintained. Accumulating data suggest a new hypothesis is reasonable that pesticide-related developmental immunotoxicity may contribute in part to Gambian seasonal mortality. As such we plan to explore this idea through a number of investigations. Planned studies will include exploring seasonal changes in breast milk levels of pesticides presently in use, and investigating maternal serum levels and body fat changes and directly correlating these with immune parameters over postnatal time in offspring. Levels of persistent OC pesticides in serum of mothers and siblings of early death individuals will also be determined, and compared to appropriate controls. Through these studies, we hope to understand in more detail the contribution, if any, of seasonal variation in pesticide exposure to immune function and survival risk in this population of rural Gambian villagers.
REFERENCES
- Abdel-Khalik M. M., Hanafy M. S., Abdel-Aziz M. I. Studies on the teratogenic effects of deltamethrin in rats. Dtsch. Tierartl. Wochenschr. 1993; 100: 142–143
- Abdel-Rahman A. A., Blumenthal G. M., Abou-Donia S. A., Ali F. A., Abdel-Monem A. E., Abou-Donia M. B. Pharmacokinetic profile and placental transfer of a single intravenous injection of [14C]-chlorpyrifos in pregnant rats. Arch. Toxicol. 2002; 76: 452–459, [PUBMED], [INFOTRIEVE], [CROSSREF]
- Agency for Toxic Substances and Disease Registry (ATSDR). U.S. Department of Health and Human Services, 2003, 〈www.atsdr.cdc.gov/toxprofiles/tp155-c2.pdf〉
- Aguilar A., Raga J. A. The striped dolphin epizootic in the Mediterranean Sea. Ambio 1993; 22: 524–528
- Banerjee B. D., Saha S., Mohapatra T. K., Ray A. Influence of dietary protein on DDT-induced immune responsiveness in rats. Indian J. Exp. Biol. 1995; 33: 739–744, [PUBMED], [INFOTRIEVE], [CSA]
- Banerjee B. D., Ray A., Pasha S. T. A comparative evaluation of immunotoxicity of DDT and its metabolites in rats. Indian J. Exp. Biol. 1996; 34: 517–522, [PUBMED], [INFOTRIEVE], [CSA]
- Barnett J. B., Soderberg L. S. F., Menna J. H. The effect of prenatal chlordane exposure on the delayed hypersensitivity response of BALB/c mice. Toxicol. Lett. 1987; 25: 173–183, [CSA], [CROSSREF]
- Barnett J. B., Gibson L. F., Landreth K. S. Developmental immunotoxicity of chlordane. Developmental Immunotoxicology, S. D. Holladay. CRC Press, Boca Raton, FloridaUSA 2005; pp. 153–168
- Beaman J. R., Finch R., Gardner H., Hoffmann F., Rosencrance A., Zelikoff J. T. Mammalian immunoassays for predicting the toxicity of malathion in a laboratory fish model. J. Toxicol. Environ Health. A 1999; 56: 523–542, [PUBMED], [INFOTRIEVE], [CSA], [CROSSREF]
- Benjaminov O., Hoffer E., Taitelman U., Urbach J., Brandes J. M. Parathion transfer and acetylcholinerterase activity in an in vitro-perfused term human placenta. Vet. Human Toxicol. 1992; 34: 10–12, [CSA]
- Bissacot D. Z., Vassilieff I. HPLC determination of flumethrin, deltamethrin, cypermethrin, and cyhalothrin residues in the milk and blood of lactating dairy cows. J. Anal. Toxicol. 1997; 21: 397–402, [PUBMED], [INFOTRIEVE], [CSA]
- Blakley B. R., Yole M. J., Brousseau P., Boermans H., Fournier M. Effect of chlorpyrifos on immune function in rats. Vet. Human Toxicol. 1999; 41: 140–144, [CSA]
- Blaylock B. L., Abdel-Nasser M., Mcarty S. M., Knesel J. A., Tolson K. M., Ferguson P. W. Suppression of cellular immune responses in BALB/c mice following oral exposure to permethrin. Bull. Environ. Contam. Toxicol. 1995; 54: 768–774, [PUBMED], [INFOTRIEVE], [CSA], [CROSSREF]
- Bonyata K. 2002, 〈www.kellymom.com/health/illness/mom-health-misc.html.〉
- Choi S. M., Yoo S. D., Lee B. M. Toxicological characteristics of endocrine-disrupting chemicals: Developmental toxicity, carcinogenicity, and mutagenicity. J. Toxicol. Environ. Health B Crit. Rev. 2004; 7: 1–24, [PUBMED], [INFOTRIEVE], [CSA]
- Collinson A. C., Moore S. E., Cole T. J., Prentice A. M. Birth season and environmental influences on patterns of thymic growth in rural Gambian infants. Acta Paediatr. Scand. 2003; 92: 1014–1020
- Cooper G. S., Martin S. A., Longnecker M. P., Sandler D. P., Germolec D. R. Associations between plasma DDE levels and immunologic measures in African-American farmers in North Carolina. Environ. Health Perspect. 2004; 112: 1080–1084, [PUBMED], [INFOTRIEVE], [CSA]
- Daniel V., Huber W., Bauer K. Associations of dichlorodiphenyltrichloroethane (DDT) and dichlorodiphenyldichloroethylene (DDE) 4.4 blood levels with plasma IL-4. Arch. Environ. Health 2002; 57: 541–547, [PUBMED], [INFOTRIEVE], [CSA]
- De Guise S., Martineau D., Beland P., Fournier M. Effects of in vitro exposure of beluga whale leukocytes to selected organochlorines. J. Toxicol. Environ. Health 1998; 55: 479–493, [CROSSREF]
- Desi I., Varga L., Dobronyi I., Szklenaric G. Immunotoxicological investigation of the effect of a pesticide: Cypermethrin. Arch. Toxicol. 1985; 8: 305–309
- Dewailly E., Bruneau S., Laliberte C., Belles-Iles M., Weber J. P., Roy R. Breast milk contamination by PCBs and PCDDs/ PCDFs in arctic Quebec: Preliminary results on the immune status of Inuit infants. Dioxin '93. 1993a; pp. 403–406
- Dewailly E., Bruneau S., Laliberte C., Gingras S., Belanger D., Ferron L. Health status at birth of Inuit newborn prenatally exposed to organochlorines. Chemosphere 1993b; 27: 1–3, [CROSSREF]
- Enan E., Pinkerton K. E., Peake J., Matsumura F. Deltamethrin-induced thymus atrophy in male BALB/c mice. Biochem. Pharmacol. 1996; 51: 447–454, [PUBMED], [INFOTRIEVE], [CSA], [CROSSREF]
- Faith R. E., Moore J. A. Impairment of thymus-dependent immune functions by exposure of the developing immune system to 2,3,7,8-tetrachlorodibenzo-p-dioxin (TCDD). J. Toxicol. Environ. Health 1977; 3: 451–464, [PUBMED], [INFOTRIEVE]
- Fine J. S., Gasiewicz A., Silverstone A. E. Lymphocyte stem cell alterations following perinatal exposure to 2,3,7,8-tetrachlorodibenzo-p-dioxin. Mol. Pharmacol. 1989; 35: 18–28, [PUBMED], [INFOTRIEVE], [CSA]
- Galetin-Smith R., Pavkov S., Roncevic N. DDT and PCBs in human milk: Implication for breast feeding infants. Bull. Environ. Contam. Toxicol. 1990; 45: 811–818, [PUBMED], [INFOTRIEVE], [CSA]
- Galloway T., Handy R. Immunotoxicity of organophosphorous pesticides. Ecotoxicology 2003; 12: 345–363, [PUBMED], [INFOTRIEVE], [CROSSREF]
- Gehrs B. C., Smialowicz R. J. Persistent suppression of delayed-type hypersensitivity in adult F344 rats after perinatal exposure to 2,3,7,8-tetrachlorodibenzo-p-dioxin. Toxicology 1999; 134: 79–88, [PUBMED], [INFOTRIEVE], [CSA], [CROSSREF]
- Gogal R. M., Jr., Holladay S. D. Developmental immunotoxicity of therapeutic immunosuppressive drugs. Developmental Immunotoxicology, S. D. Holladay. CRC Press, Boca Raton, FloridaUSA 2005; pp. 179–192
- Gomes M. S., Bernardi M. M., Spinosa H. S. Effects of prenatal pyrethroid insecticide exposure on the sexual development of rats. Vet. Human Toxicol. 1991; 33: 427–428, [CSA]
- Hadnagy W., Leng G., Sugiri D., Ranft U., Idel H. Pyrethroids used indoors—immune status of humans exposed to pyrethroids following a pest control operation—a year follow-up study. Int. J. Hyg. Environ. Health 2003; 206: 93–102, [PUBMED], [INFOTRIEVE], [CSA]
- Classes of Pesticides., W. J. Hayes, E. R. Laws. Academic Press Inc., New York 1990; Vol 3
- Holladay S. D., Lindstrom P., Blaylock B. L., Comment C. E., Germolec D. R., Heindel J. J., Luster M. I. Perinatal thymocyte antigen expression and postnatal immune development altered by gestational exposure to 2,3,7,8-tetrachlorodibenzo-p-dioxin (TCDD). Teratology 1991; 44: 385–393, [PUBMED], [INFOTRIEVE]
- Holladay S. D., Luster M. I. Developmental immunotoxicology. Developmental Toxicology, C. A. Kimmel, J. Buelke-Sam. Raven Press, New York 1994; pp. 93–118
- Holladay S. D., Smialowicz R. J. Development of the murine and human immune system: Differential effects of immunotoxicants depend on time of exposure. Environ. Health. Perspect. 2000; 108: 463–473, [PUBMED], [INFOTRIEVE], [CSA]
- Hrubec T. C., Blaylock B. L., Holladay S. D. Developmental immunotoxicity of halogenated aromatic hydrocarbons. Developmental Immunotoxicology, S. D. Holladay. CRC Press, Boca Raton, FloridaUSA 2005; pp. 139–152
- Ivie G. W., Hunt L. M. Metabolites of cis- and trans-permethrin in lactating goats. J. Argic. Food Chem. 1980; 28: 1131–1138, [CSA], [CROSSREF]
- Julien G., Baxter J. D., Crago M., Ilecki H. J., Therien F. Chronic otitis media and hearing deficit among native children of Kuujjuaraapik (Northern Quebec): A pilot project. Canad. J. Pub. Health 1987; 78: 57–61, [PUBMED], [INFOTRIEVE]
- Kacew S. Current issues in lactation: Advantages, environment, silicone. Biomed. Environ. Sci. 1994; 7: 307–319, [PUBMED], [INFOTRIEVE], [CSA]
- Karpuzoglu-Sahin E., Hissong B. D., Ahmed S. A. Interferon-gamma levels are upregulated by 17-beta-estradiol and diethylstilbestrol. J. Reprod. Immunol. 2001; 52: 113–127, [PUBMED], [INFOTRIEVE], [CSA], [CROSSREF]
- The Basic Science of Poisons., C. D. Klaassen, M. O. Amdur, J. Doull. McGraw-Hill, New York 1996
- Kunisue T., Someya M., Monirith I., Watanabe M., Tana T. S., Tanabe S. Occurrence of PCBs, organochlorine insecticides, tris(4-chlorophenyl)methane, and tris(4-chlorophenyl)methanol in human breast milk collected from Cambodia. Arch. Environ. Contam. Toxicol. 2004; 46: 405–412, [PUBMED], [INFOTRIEVE], [CSA], [CROSSREF]
- Lahvis G. P., Wells R. S., Casper D., Via C. S. In vitro lymphocyte response of bottlenose dolphins (Tursiops truncatus): Mitogen-induced proliferation. Mar. Environ. Res. 1993; 35: 115–119, [CSA], [CROSSREF]
- Lahvis G. P., Wells R. S., Kuehl D. W., Stewart J. L., Rhinehart H. L., Via C. S. Decreased lymphocyte responses in free-ranging bottlenose dolphins (Tursiops truncatus) are associated with increased concentrations of PCBs and DDT in peripheral blood. Environ. Health Perspect. 1995; 103: 67–72, [PUBMED], [INFOTRIEVE]
- Lee M., Harris K., Trowbridge N. Effect of the level of dietary protein on the toxicity of dieldrin for the laboratory rat. J. Nutr. 1964; 84: 136–144, [PUBMED], [INFOTRIEVE]
- Lindstrom G., Hooper K., Petreas M., Stephens R., Gilman A. Workshop on Perinatal Exposure to Dioxins-Like Compounds. I. Summary. Environ. Health Perspect. 1995; 103: 135–142, [PUBMED], [INFOTRIEVE]
- Linzey D., Burroughs J., Hudson L., Marini M., Robertson J., Bacon J. Role of environmental pollutants on immune functions, parasitic infections and limb malformations in marine toads and whistling frogs from Bermuda. Int. J. Environ. Health Res. 2003; 13: 125–148, [PUBMED], [INFOTRIEVE], [CSA], [CROSSREF]
- Loevinsohn M. E. Insecticide use and increased mortality in rural Central Luzon, Philippines. Lancet 1987; 1359–1362, [CROSSREF]
- Madsen C., Claesson M. H., Ropke C. Immunotoxicity of the pyrethroid insecticides deltametrin and alpha-cypermetrin. Toxicology 1996; 107: 219–227, [PUBMED], [INFOTRIEVE], [CSA], [CROSSREF]
- Milston R. H., Fitzpatrick M. S., Vella A. T., Clements S., Gundersen D., Feist G. Short-term exposure of Chinook salmon (Oncoryhnchus tshawytscha) to o,p′-DDE or DMSO during early life-history stages causes long-term humoral immunosuppression. Environ. Health Perspect. 2003; 111: 1601–1607, [PUBMED], [INFOTRIEVE], [CSA]
- Moore S. E., Cole T. J., Collinson A. C., Poskitt E. M., McGregor I. A., Prentice A. M. Prenatal or early postnatal events predict infectious deaths in young adulthood in rural Africa. Int. J. Epidemiol. 1999; 28: 1088–1095, [PUBMED], [INFOTRIEVE], [CSA], [CROSSREF]
- Moore S. E., Collinson A. C., Prentice A. M. Immune function in rural Gambian children is not related to season of birth, birth size, or maternal supplementation status. Am. J. Clin. Nutr. 2001; 74: 840–847, [PUBMED], [INFOTRIEVE], [CSA]
- Muckle G., Ayotte P., Dewailly E., Jacobson S. W., Jacobson J. L. Prenatal exposure of the northern Quebec Inuit infants to environmental contaminants. Environ. Health Perspect. 2002; 109: 1291–1299, [CSA]
- Mulvad G., Pedersen H. S., Hansen J. C., Dewailly E., Jul E., Pederson M. B., Bjerregaard P., Malcom G. T., Deguchi Y., Middaugh J. P. Exposure of Greenlandic Inuit to organochlorines and heavy metals through the marine food-chain: An international study. Sci. Total Environ. 1996; 186: 137–139, [PUBMED], [INFOTRIEVE], [CSA], [CROSSREF]
- Navarro H. A., Basta P. V., Seidler F. J., Slotkin T. A. Neonatal chlorpyrifos administration elicits deficits in immune function in adulthood: A neural effect?. Brain Res. Dev. Brain Res. 2001; 130: 249–252, [PUBMED], [INFOTRIEVE], [CSA], [CROSSREF]
- Neishabouri E. Z., Hassan Z. M., Azizi E., Ostad S. N. Evaluation of immunotoxicity induced by diazinon in C57Bl/6 mice. Toxicology 2004; 196: 173–179, [PUBMED], [INFOTRIEVE], [CSA], [CROSSREF]
- Nunez G. M. A., Estrada I., Calderon-Aranda E. S. DDT inhibits the functional activation of murine macrophages and decreases resistance to infection by Mycobacterium microti. Toxicology 2002; 174: 201–210, [CSA], [CROSSREF]
- Prater M. R., Blaylock B. L., Chen J., Gogal R. M., Jr., Holladay S. D. Combined dermal exposure to permethrin and cis-urocanic acid suppresses the contact hypersensitivity response in C57Bl/6 mice in an additive manner. Photochem. Photobiol. B 2005; 78: 29–34, [CSA], [CROSSREF]
- Proulx J. F. Meningitis in Hudson's Bay, Northern Quebec, Canada. Arctic Med. Res. 1988; 47: 686–687, [PUBMED], [INFOTRIEVE]
- Punareewattana K., Smith B. J., Blaylock B. L., Robertson J. L., Gogal R. M., Jr., Prater M. R., Longstreth J., Holladay S. D. Topical permethrin exposure causes thymic atrophy and persistent inhibition of the contact hypersensitivity response in C57BI/6 mice. Int. J. Toxicol. 2000; 19: 383–389, [CSA]
- Punareewattana K., Smith B. J., Blaylock B. L., Gogal R. M., Jr., Prater M. R., Longstreth J., Holladay S. D. Topical permethrin inhibits antibody production and macrophage function in C57Bl/6 mice. Food Chem. Toxicol. 2001; 39: 133–139, [PUBMED], [INFOTRIEVE], [CSA]
- Rashatwar S. S., Matsumura F. Interaction of DDT and pyrethroids with calmodulin and its significance in the expression of enzyme activities of phosphodiesterase. Biochem. Pharmacol. 1985; 34: 1689–1694, [PUBMED], [INFOTRIEVE], [CSA], [CROSSREF]
- Repetto R., Baliga S. Pesticides and the Immune System: The Public Health Risks. World Resources Institute, Washington, DC 1996
- Sassine A., Moura A., Leo V. M., Bustillos O. V. Cypermethrin residues determination in the milk of a lactating dairy cow by gas chromatography-ion trap mass spectrometry. J. Anal. Toxicol. 2004; 28: 238–241, [PUBMED], [INFOTRIEVE], [CSA]
- Saxena M. C., Siddiqui M. K., Bhargava A. K., Murti C. R., Kutty D. Placental transfer of pesticides in humans. Arch. Toxicol. 1981; 48: 127–134, [PUBMED], [INFOTRIEVE], [CROSSREF]
- Saxena M. C., Seth T. D., Mahajan P. L. Organochlorine pesticides in human placenta and accompanying fluid. Int. J. Environ. Anal. Chem. 1980; 7: 245–251, [PUBMED], [INFOTRIEVE]
- Siddiqui M. K., Saxena M. C., Bhargava A. K., Murti C. R., Kutty D. Chlorinated hydrocarbon pesticides in blood of newborn babies in India. Pesticide Monit. J. 1981; 15: 77–79
- Spencer F., Berhane Z. Uterine and fetal characteristics in rates following a post-implantational exposure to permethrin. Bull. Environ. Contam. Toxicol. 1982; 29: 84–88, [PUBMED], [INFOTRIEVE], [CSA], [CROSSREF]
- Spyker-Cranmer J. M., Barnett J. B., Avery D. L., Cranmer M. F. Immunoteratology of chlordane: Cell-mediated and humoral immune responses in adult mice exposed in utero. Toxicol. Appl. Pharmacol. 1982; 62: 402–408, [PUBMED], [INFOTRIEVE], [CSA], [CROSSREF]
- Thrasher J. D., Heuser G., Broughton A. Immunological abnormalities in humans chronically exposed to chlorpyrifos. Arch. Environ. Health 2002; 57: 181–187, [PUBMED], [INFOTRIEVE], [CSA]
- Thrasher J. D., Madison R., Broghton A. Immunological abnormalities in humans exposed to chlorpyrifos: Preliminary observations. Arch. Environ. Health 1993; 48: 89–93, [PUBMED], [INFOTRIEVE], [CSA]
- Turner P. C., Moore S. E., Hall A. J., Prentice A. M., Wild C. P. Modification of immune function through exposure to dietary aflatoxin in Gambian children. Environ. Health Perspect. 2003; 111: 217–220, [PUBMED], [INFOTRIEVE], [CSA]
- USEPA. Pesticide Fact Sheet Number 199: Cypermethrin. U.S. Environmental Protection Agency Office of Pesticide Programs, Registration Div, Washington, DC 1989a
- USEPA. Pesticide Environmental Fate One Line Summary: DDT (p, p′). U.S. Environmental Protection Agency, Washington, DC 1989b
- Weber H., Birnbaum L. S. 2,3,7,8-Tetrachlorodibenzo-p-dioxin (TCDD) and 2,3,7,8-tetrachlorodibenzo-p-furan (TCDF) in pregnant C57BL/6N mice: Distribution and excretion. Arch. Toxicol. 1985; 57: 159–162, [PUBMED], [INFOTRIEVE], [CROSSREF]
- Wiltrout R. W., Ercegovich C. D., Ceglowski W. S. Humoral immunity in mice following oral administration of selected pesticides. Bull. Environ. Contam. Toxicol. 1978; 20: 423–431
- You L., Gazi E., Archibeque-Engle S., Casanova M., Conolly R. B., Heck H. A. Transplacental and lactational transfer of p,p-DDE in Sprague-Dawley rats. Toxicol. Appl. Pharmacol. 1999; 157: 134–144, [PUBMED], [INFOTRIEVE], [CSA], [CROSSREF]