Abstract
Spatiotemporal characteristics and anthropogenic signals of aerosol optical thickness (AOT) distributions over Korea are investigated in this study using AOT time-series data from the Moderate Resolution Imaging Spectroradiometer (MODIS). AOT observations, a quantitative measure of the atmospheric quality, had significant geographical variations during the study period (2000–2010). Comparing metro cities or counties with similar populations, western regions showed higher AOT values than eastern regions. Particular matter with a diameter < 10 µm (PM10), including aeolian dust or yellow dust, is the primary component of atmospheric aerosols, and their transport into the region has shown a strong seasonal pattern with its peak from March to April and lows from July to September. These seasonal dust patterns, however, did not correspond well to temporal AOT records, which typically reached the maximum level in June nationwide. Rather, widespread regional fire events and humidity showed significant correlations with AOT time-series. This correlation rapidly increased as the range of fire occurrence was extended to the west as far as 115°E. The relative humidity also had a significant correlation with AOT during the month of June. In addition to urban emission of anthropogenic aerosols, regional biomass burning and secondary growth of hygroscopic aerosols are considered important contributors to the degradation of the atmospheric environment during the non-Asian Dust season over the Korean Peninsula.
Introduction
Atmospheric aerosols, suspended particles (solid or liquid) in a gas medium, are known as a significant contributor to the urban air quality, public health, and hydrologic cycles, and considered one of the key variables in climate modeling because they play an important role in the interactions between solar radiation and the atmosphere radiative forcing in climate change (Dockery and Pope Citation1994; Kwon et al. Citation2002; Lee et al. Citation2009; Remer et al. Citation2005; Valipour Citation2014a; Valipour and Eslamian Citation2014; Valipour Citation2015b, Citation2015c, Citation2015d). Unfortunately, the observation network of regional aerosols is geographically limited, and the impacts of their spatiotemporal distributions and dispersions on climate changes still remain uncertain (IPCC Citation2013). Long-term monitoring of the natural and anthropogenic aerosols is still technically limited due to the lack of continuous atmospheric data over the country. In fact, it was only lately that real-time PM10 or PM2.5 concentrations have formally been collected for public health and environmental purposes. The atmospheric conditions of East Asia have been changing rapidly over recent decades, and natural and anthropogenic aerosols are commonly documented in the region. Recent studies reported that atmospheric aerosol concentrations showed regional and seasonal characteristics in part due to industrialization, urbanization, the Asian Dust, and natural or man-made fires (Kim et al. Citation2007; Lee et al. Citation2005; Lee, Yang, and Wenig Citation2010; Richter et al. Citation2005; Song et al. Citation2009).
Historically, the inflow of yellow dust, originated from inland China and inner Mongolia, has had a significant impact on the atmospheric quality of the Korean Peninsula. Particular matter with a diameter < 10 µm (PM10), including aeolian dust or the Asian Dust, is the primary component of atmospheric aerosols, and its transportation into the region has shown a strong seasonal pattern with its peak from March to April. In general, 87% of dust events in Korea occurred in spring (Kim Citation2008). The major paths of the dust phenomenon were recently analyzed based on the National Oceanic and Atmospheric Administration (NOAA) Hybrid Single-Particle Lagrangian Integrated Trajectory (HYSPLIT) model. The origins of Asian Dust were categoried into one of four regions, including Inner Mongolia, Gobi, Manchuria, and Loess Plateau (Kim and Lee Citation2013). In addition, rapid growth of industrial and agricultural activities in East China has become an important anthropogenic source of fine aerosols (Bergin et al. Citation1999; Wang, Zhang, and Pu Citation2001; Valipour Citation2014b, Citation2015a). Therefore, industrial areas of inland China and the Gobi desert are among the primary external sources of atmospheric aerosols. A recent study reported that 69.3% and 30.7% of the background sources of high-PM10 were categorized as external and internal origins, respectively, in Seoul, Korea (Lee, Ho, and Choi Citation2011). Another important external aerosol source is fire activities in Asian regions. Recent studies have focused on atmospheric pollution caused by biomass burning and its long-range transport. East Asia is an important source region of biomass burning, and substantial pollutants are emitted from biomass activities in the region. Especially, burning of the crop residue during the post-harvest seasons in eastern China may contribute to the regional degradation of the atmospheric environment (Cheng et al. Citation2014; Shin and Lee Citation2016; Yan, Ohara, and Akimoto Citation2006).
Aerosol measurements have been traditionally recorded in ground weather stations or by aircrafts. Active sensor systems, such as lidar, can also be used to determine the vertical distributions of atmospheric aerosols, but operational costs are highly expensive and only practical for geographically limited areas. Since intense urbanization and industrialization in the eastern China and Korean Peninsula had direct and sensitive impacts on the atmospheric quality of the region from ecological and socioeconomic perspectives, broad-scaled satellite-based technologies are required for broad-scaled aerosol monitoring in a systematic, continuous, timely manner. Aerosol detection algorithms were significantly improved, and aerosol properties, such as aerosol optical thickness (AOT) and aerosol types, are retrieved by satellite sensors, providing a good agreement with ground-based measurements (Holben et al. Citation1998; Remer et al. Citation2002). AOT describes the atmospheric attenuation of sunlight by a column of aerosol, and it assesses the vertically integrated amount of aerosols in the atmosphere (Kaufman, Tanré, and Boucher Citation2002). One of the important aspects in measuring AOT is that small solid or liquid particles suspended in the atmosphere play a major role in the energy balance of the Earth. This aerosol optical parameter is a key component of radiative forcing of climate by reflecting sunlight back to space with its impact on cloud microphysics and albedo (Kaufman et al. Citation1997). Since aerosols are highly variable in space, time and composition, understanding the amount, distribution, and changes in these characteristics is necessary for meaningful estimates of their effects on anthropogenic climate forcing, forecasting the air quality and public health (Barnaba et al. Citation2011; Remer et al. Citation2008). Along with the importance of AOT measurements, AOT retrieving methods have been continuously developed since the routine operation of satellite earth observations in the 1970s (Herman et al. Citation1997; Knapp and Stowe Citation2002; Lee et al. Citation2009; McCormick et al. Citation1993; Rao, McClain, and Stowe Citation1989; Remer et al. Citation2005). Advances in aerosol remote sensing allowed researchers to analyze satellite-measured aerosol datasets for scientific uses at various scales (Kaufman, Tanré, and Boucher Citation2002; Lee et al. Citation2009).
The Moderate Resolution Imaging Spectroradiometer (MODIS), a hyperspectral earth-observing satellite sensor operated by the National Aeronautics and Space Administration (NASA), provides daily satellite coverage at a global scale. The MODIS system is one of the main instruments on the Terra satellite, a part of NASA’s Earth Observing System (EOS), and it has been acquiring aerosol observations since 2000. MODIS AOT data are validated by a network of ground sunphotometers worldwide, called AERONET and broadly used in aerosol research (Holben et al. Citation1998; Remer et al. Citation2002; Rahimia et al. Citation2015).
In East Asia, MODIS AOT records showed strong seasonal patterns, but their annual variations differed from region to region. MODIS AOT measurements did not show a consistent relationship with PM10 (Song et al. Citation2009). Interestingly, monthly mean AOT reached its peak in June, which is about two months later than the typical dust season in Korea (Kim et al. Citation2007). Therefore, further investigation on anthropogenic sources of aerosol emission other than Asian Dust is required to explain temporal discrepancy between surface PM10 and columnar aerosol concentrations, represented by MODIS AOT. Hygroscopic effects and biomass burning have been suspected as two important factors that may contribute to increases in atmospheric aerosol concentrations in the region. Thus, this study was conducted to identify environmental or anthropogenic signals from geographic aerosol distributions and their seasonal characteristics over South Korea analyzing the relationships between decadal MODIS products of AOT and active fire time-series, PM10, land use, and humidity datasets.
Materials and methods
Study area
The Korean Peninsula is the eastmost part of Northeast Asia and located west of the Pacific Ocean (). Located between 33°06′43″N and 43°00′42″N paralles and 124°11′04″E and 131°52′22″E meridians, the peninsula is characterized by a wide range of climatic regimes and apparent seasonality. The mean annual temperature of Korea ranges from 6°C to 16°C, and it varies substantially depending on proximity to the ocean, elevation, and latitude. Seasonal variations of precipitation are also significant with the mean of about 1200 mm, and the East Asian monsoon strongly contributes to the climate of the region. The summer monsoon is characterized by hot and moist air blowing from the West Pacific and East China Sea. The frontal systems of monsoons and typhoons bring torrential rain in the summer, and more than 60% of annual total precipitation occur between June and September. The winter climate is characterized by a high pressure system, the Siberian High, over the northern part of Asia. The dry, cold northwesterly winds have direct impacts on the Korean peninsula, and winter precipitation, on average, is less than 10% of the annual total (NGII Citation2010; Nieuwolt Citation1977).
Figure 1. Study area. South Korea is divided into 16 administrative units, including 7 metro cities and 9 provinces, including one island province (Jeju).
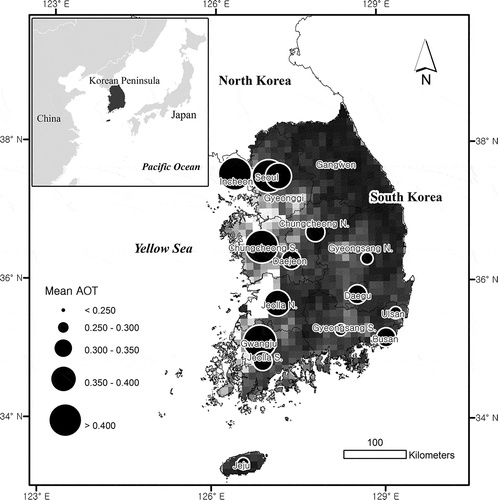
Sixteen administrative units covering the entire South Korea were selected as the study area. They consist of highly urbnaized areas, eight rural provinces and a volcanic island. Uranized areas are all metropolitan cities, including Seoul, Busan, Incheon, Daejeon, Daegu, Gwangju, and Ulsan. Rural provinces include Gyeonggi Province, South Chungcheong Province, North Chungcheong Province, South Kyeongsang Province, North Kyeongsang Province, South Jeolla Province, North Jeolla Province, and Gangwon Province. Jeju is the only island province of the nation.
Satellite data and process
MODIS MOD04 AOT time series from 2000 to 2010 were collected through NASA’s Goddard Space Flight Center (LAADS, http://ladsweb.nascom.nasa.gov/). It has been difficult to retrieve aerosol content over land on a broad scale until MODIS was launched because earlier satellite measurements were limited to no more than two channels and high surface reflectance was a challenging problem. The “deep blue” approach uses the blue channel, where the surface reflectance is relatively lower, to find “dark” pixels. For these dark pixels, the contribution of surface reflectance radiance can be better separated from atmospheric conditions, and aerosol properties are retrieved by the method (Hsu et al. Citation2004).
Land aerosol observations are also based on near-infrared (2.1 μm, 3.8 μm) channels, which are relatively free from atmospheric interference, and AOT is determined using lookup tables (LUT) that simulate spectral reflectance for expected aerosol properties (Levy et al. Citation2010). The data are 10-km spatial resolution, and individual data grid unit is composed of 135-by-203 10-km pixels. MODIS AOT were rigorously validated by comparison with collocated ground-based AERONET measurements. MODIS AOT retrievals over land were known within an expected error envelope of ±(0.05 + 0.15 AOT) (Chu et al. Citation2002; Hsu et al. Citation2004, Citation2006; Levy et al. Citation2007, Citation2010; Remer et al. Citation2005, Citation2008). The column concentration of atmospheric aerosols are determined from a light extinction coefficient, which is the fraction of light that is scattered or absorbed by atmospheric aerosol particles and molecules as a function of altitude. AOT is the sum of light extinction coefficients integrated over a vertical column, and it estimates the amount of aerosols for the whole atmospheric column (Kaufman et al. Citation1997; Levy et al. Citation2007; Wong, Nichol, and Lee Citation2009):
where τ and σ represent AOT and light extinction, respectively. MODIS AOT values are determined from a LUT that has pre-established the relationship between surface reflectance and AOT measurements (Kaufman et al. Citation1997; Levy et al. Citation2007, Citation2010). AOT data were processed with ERDAS IMAGINE 9.1. Monthly mean AOT was calculated per study unit, and correlation coefficient analysis was conducted using the Statistical Package for Social Science (SPSS) software package.
Regional fire event data, MODIS Global Monthly Fire Location Product (MCD14ML), were collected from MODIS Active Fire and Burned Area Products web interface (http://modis-fire.umd.edu/). The fire location data contain the geographic coordinates, date, and brightness temperatures for each pixel detected daily by MODIS sensors. The fire product files are created separately on a monthly basis, and distributed as a plain ASCII format with fixed-width fields (Giglio Citation2013). Active fire detection for MODIS is originally based on algorithm s developed for the AVHRR and TRMM VIRS (Giglio, Kendall, and Justice Citation1999). Brightness temperatures measured by two channels, 4 and 11 µm, are used for fire detection. If the fire emits energy strong enough, absolute fire detection is used. However, if it does not, a relative detection approach is adopted instead. The latter method compares the target pixel’s temperature with the background thermal emission of surrounding pixels to identify fires (Justice et al. Citation2002). A similar method is applied to the basic MODIS level 2 fire product, MOD14 Fire and Thermal Anomalies. This product is defined in the MODIS orbit geometry covering approximately 2340 × 2030 km (swath) and used to generate all higher-level fire products. Each location stored in the MCD14ML product represents the center of a 1 km pixel that is flagged by the MOD14 Fire and Thermal Anomalies algorithm as containing one or more fires within the pixel (Giglio Citation2013). The daily fire location data were collected over 100°E–130°E in longitude and 20°N–50°N in latitude to broadly cover the effective range of biomass burning activities around Korean Peninsula (Lee et al. Citation2005; Lee Citation2012; Shin and Lee Citation2016). For further spatial analysis, individual fire-event data were temporally integrated into monthly intervals and geographically accumulated into1°-by-1° grids for comparison with AOT data. ESRI’s ArcGIS 9.3 software package was used for mapping and spatial analysis of the fire location data.
Ground data
The Asian Dust is a primary concern and an important factor for air-quality control in the region. Asian Dust occurrence (threshold = 300 µm/m3 (hourly mean) and ocular observation), precipitation, and relative humidity (RH) data were obtained from 78 ground monitoring stations nationwide operated by the Korea Meteorological Administration (KMA) for further comparison with MODIS AOT time-series. PM10 concentration data from 2003 to 2010 were collected from 26 weather stations across the nation operated by KMA. Additional PM10 data were collected from the network of the air quality monitoring system operated by the National Institute of Environmental Research (NIER), a comprehensive scientific facility primarily for urban environmental monitoring (262 stations).
Hourly raw data were converted to monthly mean values. In particular, monthly AOT levels of seven major metro cities, Seoul, Busan, Incheon, Daegu, Daejeon, Ulsan, and Gwangju, were compared to RH and rainfall data to investigate the AOT-moisture relations in the urban environment. Land use information was also used to evaluate the influence of land use types on AOT measurements. Three major land use types, urban area, agricultural land, and forests, were selected from ESA GlobCover land use data for further analyses (Sophie et al. Citation2010).
Results and discussion
Spatiotemporal characteristics of AOT
AOT observations, a quantitative measure of the atmospheric quality, had significant geographical variations during the study period (2000–2010). Particularly, the maximums of monthly AOT means showed 1.9-fold to 3.2-fold variations among the 16 study units. It is apparent that AOT time-series has a strong seasonality and geographically uneven distributions (Kim et al. Citation2007; Park Citation2014). AOT measurements were apparently higher in western regions than eastern regions, indicating the strong effects of atmospheric effluents from China (see ). Although there is no apparent boundary between the eastern and western regions, western regions are bordering the Yellow Sea or close to the sea and they are more intimately influenced by emission from eastern China due to seasonal wind patterns (southwestern and northwestern winds) over the year. Comparing metro cities or provinces with similar populations, western regions such as Incheon, Gwangju, and Gyeonggi Province showed higher AOT values than eastern regions including Busan and Kyeongsang provinces.
While Asian Dust (or Yellow Dust) typically blows in March and April in Korea, AOT reached its peak typically in June nationwide (). Particular matter with a diameter < 10 µm (PM10), including aeolian dust or yellow dust, is the primary component of atmospheric aerosols, and its transport to the region has shown a strong seasonal pattern with its peak from March to April and lows from July to September. Therefore, this PM10 measurements represented the seasonal pattern of the transport of dust into the region. On the other hand, the seasonal patterns of MODIS AOT and PM measurements did not corresponded to each other, and their peaks were separated by about two months. As a result, only a weak correlation (r = 0.264) was found between these two different measurements (PM10 and AOT).
Figure 2. Monthly mean AOT, monthly mean PM10 concentrations, and frequencies of Asian Dust by month during the study period. A standard error bar for each month is also provided.
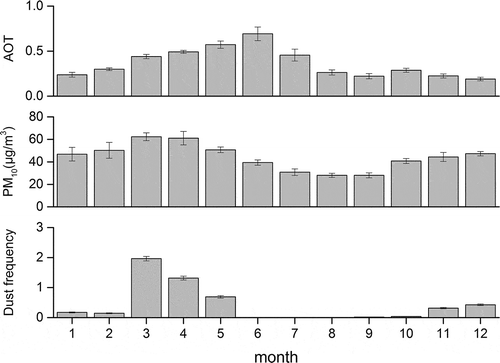
While the KMA observation sites are distributed throughout the country as a national network, the NIER air quality monitoring system is primarily located in urban areas. Comparisons of PM10 measurements between the two systems showed a very similar seasonal pattern (r = 0.945, p < 0.01). However, the NIER-based urban PM10 concentrations were significantly higher than those of KMA’s national mean PM10 (). This result confirms that urban pollutants as well as Asian Dust had a significant influence on the air quality over the Korean Peninsula (Kwon et al. Citation2002; Lee, Ho, and Choi Citation2011).
Figure 3. Seasonal patterns of national (KMA) and urban (NIER) PM10 network observations temporally well corresponded to each other. However, urban PM10 concentrations were significantly higher than national monthly mean PM10.
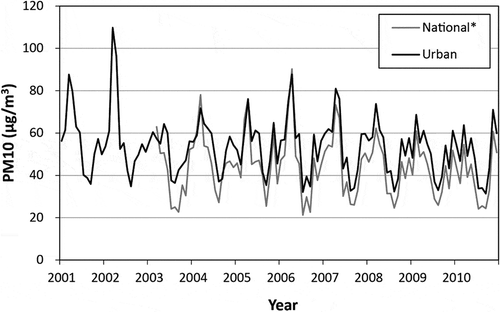
Comparative analyses of AOT data collected only in July and August, the two Asian Dust-free months during the study period, also showed that human activities were an important contributor to the aerosol concentrations of urban environments. This urban factor in the atmospheric aerosol distributions was uncovered by population size of major cities. Most metropolitan cities including Seoul (10 million), Busan (3.5 million), Incheon (2.9 million), Daegu (2.5 million), and Gwangju (1.5 million) ranked high on the two-month mean AOT observations.
Correlation analyses showed that population densities and AOT measurements had a varying degree of correlations depending on seasonal periods. For example, in spring, where the Asian Dust is typically strongest over the year, AOT had a weaker correlation (r = 0.506, p < 0.05) with a population density compared to summer (r = 0.641, p < 0.01) and fall (r = 0.556, p < 0.05). Their highest correlation was observed during the summer (June, July, and August), where Asian Dust days were rarely observed (). Spring and winter are the two seasons, where Asian Dust occurred most frequently, but their correlations with AOT values were among the lowest (statistically no relationship in winter (r = 0.156)). Since it was reported that the ratio of external and internal sources decreased in summer and increased in winter and spring (Lee, Ho, and Choi Citation2011), it is likely that summer-high AOT time-series is strongly influenced by external sources. Although the seasonal and spatial distributions of PM10 concentrations at the surface are clearly influenced by external anthropogenic emissions in spring, the summer-high AOT phenomenon is attributed to additional processes including regional patterns of synoptic pressure systems, secondary aerosol formation, or growth of hygroscopic aerosols due to increasing humidity in summer (Curci et al. Citation2015; Kim et al. Citation2007; Kim, Kim, and Sohn Citation2012; Lee, Ho, and Choi Citation2011; Takemura et al. Citation2001).
AOT–RH relations
Secondary growth of hygroscopic aerosols has been known as an important factor for increasing AOTs in summer in East Asia including Korea and China (Kim, Kim, and Sohn Citation2012; Song et al. Citation2009). Anthropogenic transport of water-soluble pollutants has been known as an influential component of spring aerosols over Korea, and it is associated with high emission of anthropogenic aerosols in East Asia (Choi et al. Citation2001; Kirillova et al. Citation2014).
Aerosols are known to have various degrees of hydroscopic growth rate with ambient moisture (Chin et al. Citation2002). As relative humidity increases, the growth factors of particle radius of aerosols continue to increase. In short, increases in relative humidity in summer lead to high mass extinction efficiency, the humidification effect, and make AOT more sensitive to mass changes of fine-mode aerosols in particular (Chu et al. Citation2002; Wang and Christopher Citation2003). Regional studies quantitatively analyzed the dependency of aerosol optical properties on relative humidity. It was reported that anthropogenic gases and smoke aerosols from East Asia seemed influential, and the hygroscopic growth factor of the aerosols was substantially elevated in the region (Choi and Kim Citation2010; Kim et al. Citation2006; Streets et al. Citation2003). This known relationship between AOT and relative humidity was well represented for the seven major metro cities until June, when the Asian monsoon typically starts in the region ().
Figure 5. A relationship between monthly AOT measurements and relative humidity. AOT rapidly increased until June, when the Asian monsoon typically starts in the region.
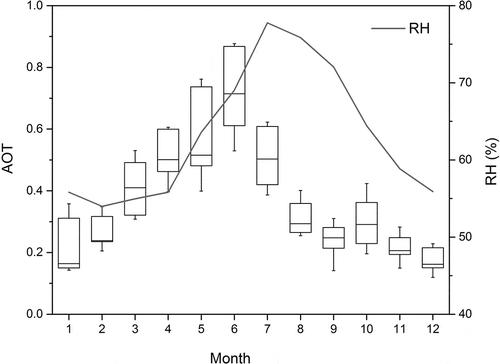
AOT means in June had significantly strong correlations primarily with relative humidity (). While spring-high concentrations of PM10 are strongly correlated with coarse particles, such as Asian Dust, summer-high AOTs are in large part attributed to fine-mode anthropogenic aerosols, which are a more contributing component compared to the dust component. The particle-size distribution of aerosols can be indirectly inferred by analyzing Ångstöm exponent (AE) data of the MODIS product. Kim, Kim, and Sohn (Citation2012) presented that AE values were low in spring (AE = 0.80 ± 0.21 in March) and high in summer (AE = up to 1.30 ± 0.37 in August), and the result indicated that the region was influenced by coarse particles in spring and by fine particles in summer. Lee (Citation2012) also reported that AE was highest in desert areas (<0.5), and an intermediate level in industrialized areas (0.8–1.0), and lowest in Siberia and other fire-prone areas (1.5–2.0) in East Asia. In addition, Lee, Ho, and Choi (Citation2011) reported that anomalous wind and pressure fields are key factors that determine the ratio of external/internal PM10 sources. Considering the significant amount of rainfall and low ratios of external/internal PM10 sources in summer, internal urban emission of anthropogenic aerosols or non-eaolian dust sources are a potential factor for the summer-high AOT of the non-Asian Dust season. Moreover, upper part of the planetary boundary layer is known to play an important role in determining aerosol concentrations, and it is largely driven by the vertical profile of relative humidity in summer months (Curci et al. Citation2015). The seasonal peak pattern associated with increasing RH was widely observed in the eastern coastal regions of China as well as Japan from both MODIS AOT and AERONET Sun-sky radiometer measurements (Kim et al. Citation2007).
Table 1. AOT means in June and their correlations (Pearson’s correlation coefficients) with RH and rainfall for each city.
Impact of biomass burning
Widespread fires were observed in the region, and total fire counts varied annually from 16,035 to 41,773 during the study period. Seasonally, most fires occurred from March to June, a spatially collective pattern became apparent in the far eastern area of China in June (). The regional fire counts showed a seasonal pattern similar to AOT time series, and they were temporally well correlated with monthly AOT records during the study period ( a,b). The correlation coefficients varied with geographic limits of the fire occurrence ranging from r = 0.374 to r = 0.605. In fact, the degree of correlation increased as the geographical range of fire counts was extended to the west, and it reached the maximum at 115°E ( c). Knowing that AOT measurements were intimately influenced by fire counts between 115°E and 130°E, and fire occurrence showed a collective pattern in eastern China in early summer, regional fire activities may have a significant impact on monthly AOT. MODIS-based studies recently conducted over East Asia suggested that anthropogenic contribution from fossil and biomass-fuel combustion dominated East China and found a meaningful correlation between fire counts and AOT of carbon monoxide and black carbon in the region (Cheng et al. Citation2010; Shin and Lee Citation2016). Therefore, the reasonably strong correlation between fire counts and AOT time series can be provided as an additional evidence for the regional influence of biomass burning on atmospheric aerosol concentrations.
Figure 6. Monthly means of fire counts (2000–2010). Monthly mean counts of fires. The number of fires were spatially integrated by 1 degree grids. Most fires occurred from March to June, an spatially collective pattern was apparent in the far eastern area of China in June, ranging from 115°E to 130°E in longitude and from 30°N to 40°N in latitude.
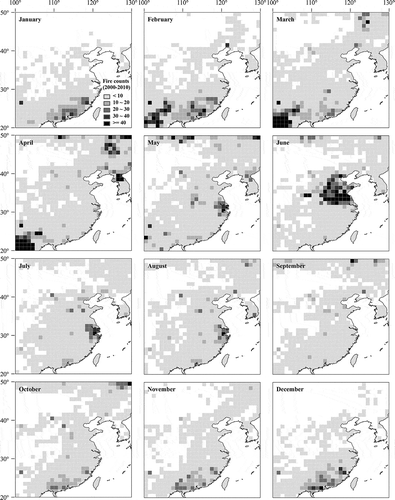
Figure 7. Time-series (2000–2010) of AOT and fire counts were compared to each other (a and b). Part of monthly AOT data in 2009 (June–September, November and December) were not available (a). Monthly AOT was temporally well correlated with fire counts that were geographically integrated into 1 degree-by-1 degree grids. Correlation between monthly AOT and fire counts rapidly increased as the geographical range of fire events was extended to the west, and it reached its highest level (r = 0.605) at 115°E (c).
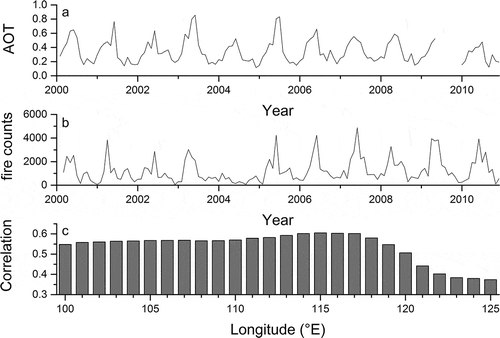
Land use relations
Human impacts on the aerosol distributions were also noticeable on different land use types. Urban areas, forests, and agricultural land, the three primary land use types of the region, were considered. Analyses showed that monthly mean AOTs of the three land use types were similar to each other, but AOTs in urban areas were significantly higher than those in cultivated land or forests ().
Figure 8. Different AOT levels were observed on three different land use types, urban areas, agricultural lands, and forests. The atmosphere was least affected by aerosols over forests, and most densely polluted over urban areas.
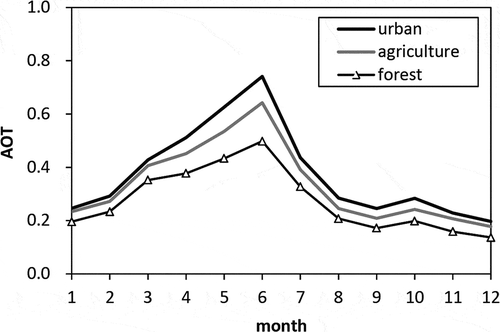
Monthly AOT differences between urban areas and forests (AOTu-f) increased with increasing AOT of urban areas (AOTu). The urban AOT departure from agricultural AOT showed the same pattern (). This outcome is another indication of human impact on aerosol contents in the atmosphere. The urban AOT also represented an east-low and west-high pattern, and the maximum-to-minimum AOT ratio of the urban areas increased from 1.95 (all land uses) to 1.97 (urban only). This result provides important implications for public health, especially respiratory diseases, in urban areas. Satellite-based air quality information, such as atmospheric aerosol content, provides critical geographical insights for respiratory disease management on a broad scale.
Conclusions
External sources of aerosols, including the Asian Dust, are an important, primary component of atmospheric aerosol contents in Korea. A strong correlation between PM10 concentrations and the inflow of the Asian Dust to the Korean Peninsula indicates that the external source of dusts is the primary component of atmospheric aerosols. In fact, annual mean AOT concentrations were higher in the western part than the eastern part of the nation, indicating the strong effects of atmospheric effluents from China and inland Mongolia. However, the seasonal pattern of AOT did not corresponded to that of PM10 measurements. Since PM10 measurements typically represents the air quality at the surface, their temporal variations often do not agree with atmospheric aerosol concentrations throughout the year. While Asian Dust typically blows in March and April in Korea, AOT observations peaked in June nationwide. This result indicated that the Asian Dust is not the only external source of pollutants for the Korean Peninsula.
The summer-high pattern of AOT was in part explained by relative humidity. AOT reached its in summer, the non-Asian Dust season in the region, and significant correlations between AOT and relative humidity during the AOT-peak month indicated that secondary formation of aerosols can be an important contributor to the urban air quality. Human influences on the aerosol distributions turned out obvious from land use-based AOT analyses. AOT levels of urban areas were significantly higher than those of cultivated land or forests, representing strong humans impacts on the air quality. AOT departure of urban areas from forest and agricultural land constantly increased as urban AOT increased on the national scale.
Widespread regional fire activities also had a significant correlation with AOT time-series. This correlation rapidly increased as the geographic range of fire occurrence was extended to the west. Study results showed that AOT measurements were intimately influenced by fire counts between 115°E and 130°E, and a spatially collective pattern was found on the distribution of fire occurrence in eastern China in early summer. These results agreed well to recent MODIS-based studies over East Asia and indicated that common fire activities had a significant impact on the spatiotemporal distributions of atmospheric aerosols in the region. Knowing that high AOT is more strongly associated with non-Asian Dust sources and anthropogenic emissions of aerosols become a significant component of air-quality control in urban areas, quantitative evaluation of the sources of aerosol emission should be further conducted.
Air quality degradation is a widely known national issue, and more dependable, broad-scaled aerosol monitoring is urgently needed for proper air quality management. Direct estimation of aerosol composition from satellite-measured data still remains as part of potential uncertainty in the study. Cross-checks of ground-based aerosol measurements and satellite records need to be accumulated for broader areas to improve stability of MODIS data applications. In addition, proportions of internal and external sources of aerosol concentrations are still under investigation and should be determined more accurately on the basis of geographic and temporal dimensions. It should also be noted that more dependable AOT studies are expected in near future as new data collection (C006) of MODIS products becomes available. For example, there are improvements in small-fire detection, removal sun-glint effects, fire-detection confidence calculation of MODIS fire datasets. Since satellite-based fire event data are produced in a timely manner on various temporal resolutions, aerosol and fire data analyses will be valuable for quicker and further detailed aerosol monitoring using better temporal intervals shorter than a month.
Acknowledgments
This research was financially supported by the Basic Science Research Program through the National Research Foundation (NRF) of Korea funded by the Ministry of Education, Science and Technology (grant number NRF-2010-0024819).
Disclosure statement
No potential conflict of interest was reported by the authors.
Additional information
Funding
References
- Barnaba, F., F. Angelini, G. Curci, and G. P. Gobbi. 2011. “An Important Fingerprint of Wildfires on the European Aerosol Load.” Atmospheric Chemistry and Physics 11: 10487–10501. doi:10.5194/acp-11-10487-2011.
- Bergin, M. H., G. R. Cass, J. Xu, C. Fang, L. M. Zeng, T. Yu, L. G. Salmon, et al. 1999. “Aerosol Radiative, Physical and Chemical Properties in Beijing during June.” Journal of Geophysical Research 106: 17969–17980. doi:10.1029/2001JD900073.
- Cheng, C. E., V. Ramanathan, G. Carmichael, S. Kulkarni, Y. Tang, B. Adhikary, L. R. Leung, and Y. Qian. 2010. “Anthropogenic Aerosol Radiative Forcing in Asia Derived from Regional Models with Atmospheric and Aerosol Data Assimilation.” Atmospheric Chemistry and Physics 10: 6007–6024. doi:10.5194/acp-10-6007-2010.
- Cheng, Z., S. Wang, X. Fu, J. G. Watson, J. Jiang, Q. Fu, C. Chen, et al. 2014. “Impact of Biomass Burning on Haze Pollution in the Yangtze River Delta, China: A Case Study in Summer 2011.” Atmospheric Chemistry and Physics 12: 4573–4585. doi:10.5194/acp-14-4573-2014.
- Chin, M., P. Ginoux, S. Kinne, O. Torres, B. N. Holben, B. N. Duncan, R. V. Martin, J. A. Logan, A. Higurashi, and T. Nakajima. 2002. “Tropospheric Aerosol Optical Thickness from the GOCART Model and Comparisons with Satellite and Sun Photometer Measurements.” Journal of Atmospheric Science 59: 461–483. doi:10.1175/1520-0469(2002)059<0461:TAOTFT>2.0.CO;2.
- Choi, E. K., and Y. P. Kim. 2010. “Effects of Aerosol Hygroscopicity on Fine Particle Mass Concentration and Light Extinction Coefficient at Seoul and Gosan in Korea.” Asian Journal of Atmospheric Environment 4-1: 55–61. doi:10.5572/ajae.2010.4.1.055.
- Choi, J. C., M. Lee, Y. Chun, J. Kim, and S. Oh. 2001. “Chemical Composition and Source Signature of Spring Aerosol in Seoul, Korea.” Journal of Geographical Research 106: 18067–18074.
- Chu, D. A., Y. J. Kaufman, C. Ichoku, L. A. Remer, D. Tanré, and B. N. Holben. 2002. “Validation of MODIS Aerosol Optical Depth Retrieval over Land.” Geophysical Research Letters 29. doi:10.1029/2001GL013205.
- Curci, G., L. Ferrero, P. Tuccella, F. Barnabam, F. Angelini, E. Bolzacchini, C. Carbone, et al. 2015. “How Much Is Particulate Matter Near the Ground Influenced by Upper-Level Processes within and above the PBL? A Summertime Case Study in Milan (Italy) Evidences the Distinctive Role of Nitrate.” Atmospheric Chemistry and Physics 15: 2629–2649. doi:10.5194/acp-15-2629-2015.
- Dockery, D. W., and C. A. Pope. 1994. “Acute Respiratory Effects of Particulate Air Pollution.” Annual Review of Public Health 15: 107–132. doi:10.1146/annurev.pu.15.050194.000543.
- Giglio, L. 2013. MODIS Collection 5 Active Fire Product User’s Guide, Version 2.5. College Park: University of Maryland. http://modis-fire.umd.edu/files/MODIS_Fire_Users_Guide_2.5.pdf.
- Giglio, L., J. D. Kendall, and C. O. Justice. 1999. “Evaluation of Global Fire Detection Algorithm Using AVHRR Infrared Data.” International Journal of Remote Sensing 20: 1947–1985. doi:10.1080/014311699212290.
- Herman, J. R., P. K. Bhartia, O. Torres, C. Hsu, C. Seftor, and E. Celarier. 1997. “Global Distribution of UV-Absorbing Aerosols from Nimbus 7/TOMS Data.” Journal of Geophysical Research 102: 16911–16922. doi:10.1029/96JD03680.
- Holben, B. N., T. F. Eck, I. Slutsker, D. Tanré, J. P. Buis, A. Setzer, E. Vermote, et al. 1998. “AERONET-A Federated Instrument Network and Data Archive for Aerosol Characterization.” Remote Sensing of Environment 66: 1–16. doi:10.1016/S0034-4257(98)00031-5.
- Hsu, N. C., S. C. Tsay, M. D. King, and J. R. Herman. 2004. “Aerosol Properties over Bright-Reflecting Source Regions.” IEEE Transactions on Geoscience and Remote Sensing 42: 557–569. doi:10.1109/TGRS.2004.824067.
- Hsu, N. C., S. C. Tsay, M. D. King, and J. R. Herman. 2006. “Deep Blue Retrievals of Asian Aerosol Properties during ACE-Asia.” IEEE Transactions on Geoscience and Remote Sensing 44: 3180–3195. doi:10.1109/TGRS.2006.879540.
- IPCC. 2013. Climate Change 2013: The Physical Science Basis. Contribution of Working Group I to the Fifth Assessment Report of the Intergovernmental Panel on Climate Change. [Stocker, T.F., D. Qin, G.-K. Plattner, M. Tignor, S.K. Allen, J. Boschung, A. Nauels, Y. Xia, V. Bex and P.M. Midgley (eds.)]. Cambridge: Cambridge University Press.
- Justice, C. O., L. Giglio, S. Korontzi, J. Owens, J. T. Morisette, D. Roy, J. Descloitres, S. Alleaume, F. Petitcolin, and Y. Kaufman. 2002. “The MODIS Fire Products.” Remote Sensing of Environment 83: 244–262. doi:10.1016/S0034-4257(02)00076-7.
- Kaufman, Y. J., D. Tanré, and O. Boucher. 2002. “A Satellite View of Aerosols in the Climate System.” Nature 419: 215–223. doi:10.1038/nature01091.
- Kaufman, Y. J., D. Tanré, L. Remer, E. F. Vermote, A. Chu, and B. N. Holben. 1997. “Operational Remote Sensing of Troposphere Aerosol over Land from EOS Moderate Resolution Imaging Spectroradiometer.” Journal of Geophysical Research 102: 17051–17067. doi:10.1029/96JD03988.
- Kim, H., J. Kim, and J. Sohn. 2012. “An Analysis of MODIS Aerosol Optical Properties and Ground-Based Mass Concentrations in Central Korea in 2009.” Journal of the Korean Earth Science Society 33: 269–279. doi:10.5467/JKESS.2012.33.3.269.
- Kim, J. 2008. “Transport Routes and Source Regions of Asian Dust Observed in Korea during the past 40 Years (1965-2004).” Atmospheric Environment 42: 4778–4789. doi:10.1016/j.atmosenv.2008.01.040.
- Kim, J., S.-C. Yoon, A. Jefferson, and S.-W. Kim. 2006. “Aerosol Hygroscopic Properties during Asian Dust, Pollution, and Biomass Burning Episodes at Gosan, Korea in April 2001.” Atmospheric Environment 40: 1550–1560. doi:10.1016/j.atmosenv.2005.10.044.
- Kim, S., and S. Lee. 2013. “The Analysis of the Weather Characteristics by Source Region of the Asian Dust Observed in South Korea.” Journal of the Korean Geographical Society 48: 167–183.
- Kim, S. W., S. C. Yoon, J. Kim, and S. Y. Kim. 2007. “Seasonal and Monthly Variations of Columnar Aerosol Optical Properties over East Asia Determined from Multi-Year MODIS, Lidar, and AERONET Sun/Sky Radiometer Measurements.” Atmospheric Environment 41: 1634–1651. doi:10.1016/j.atmosenv.2006.10.044.
- Kirillova, E. N., A. Anderson, H. Han, M. Lee, and Ö. Gustafsson. 2014. “Sources and Light Absorption of Water-Soluble Organic Carbon Aerosols in the Outflow from Northern China.” Atmospheric Chemistry and Physics 14: 1413–1422. doi:10.5194/acp-14-1413-2014.
- Knapp, K. R., and L. L. Stowe. 2002. “Evaluating the Potential for Retrieving Aerosol Optical Depth over Land from AVHRR Pathfinder Atmospheric Data.” Journal of the Atmospheric Sciences 59: 279–293. doi:10.1175/1520-0469(2002)059<0279:ETPFRA>2.0.CO;2.
- Kwon, -H.-J. S.-H., C. Y. Chun, F. Lagarde, and G. Pershagen. 2002. “Effects of the Asian Dust Events on Daily Mortality in Seoul.” Environmental Research 90: 1–5. doi:10.1006/enrs.2002.4377.
- Lee, K. 2012. “Impact of Northeast Asian Biomass Burning Activities on Regional Atmospheric Environment.” Journal of Korean Association of Geographic Information Studies 15: 184–196. doi:10.11108/kagis.2012.15.1.184.
- Lee, K., Z. Li, Y. Kim, and A. Kokhanovsky. 2009. “Atmospheric Aerosol Monitoring from Satellite Observations: A History of Three Decades.” In Atmospheric and Biological Environmental Monitoring, edited by Y. Kim, U. Platt, M. B. Gu, and H. Iwahashi, 13–38. New York: Springer.
- Lee, K. H., J. E. Kim, Y. J. Kim, J. Kim, and W. Von Hoyningen-Huenec. 2005. “Impact of the Smoke Aerosol from Russian Forest Fires on the Atmospheric Environment over Korea during May 2003.” Atmospheric Environment 39: 85–99. doi:10.1016/j.atmosenv.2004.09.032.
- Lee, S., C. H. Ho, and Y. S. Choi. 2011. “High-PM10 Concentration in Seoul, Korea: Background Sources and Related Meteorological Conditions.” Atmospheric Environment 45: 7240–7247. doi:10.1016/j.atmosenv.2011.08.071.
- Lee, Y. C., X. Yang, and M. Wenig. 2010. “Transport of Dusts from East Asian and Non-East Asian Sources to Hong Kong during Dust Storm Related Events 1996-2007.” Atmospheric Environment 44: 3728–3738. doi:10.1016/j.atmosenv.2010.03.034.
- Levy, R. C., L. Remer, S. Mattoo, E. Vermote, and Y. J. Kaufman. 2007. “A Second-Generation Algorithm for Retrieving Aerosol Properties over Land from MODIS Spectral Reflectance.” Journal of Geophysical Research 124: 2046–2070.
- Levy, R. C., L. A. Remer, R. G. Kleidman, S. Mattoo, C. Ichoku, R. Kahn, and T. F. Eck. 2010. “Global Evaluation of the Collection 5 MODIS Dark-Target Aerosol Product over Land.” Atmospheric Chemistry and Physics 10: 10399–10420. doi:10.5194/acp-10-10399-2010.
- McCormick, M. P., D. M. Winker, E. V. Browell, J. A. Coakley, C. S. Gardner, R. M. Hoff, G. S. Kent, et al. 1993. “Scientific Investigations Planned for the Lidar In-Space Technology Experiment (LITE).” Bulletin of the American Meteorological Society 74: 205–214. doi:10.1175/1520-0477(1993)074<0205:SIPFTL>2.0.CO;2.
- NGII (National Geographic Information Institute). 2010. The Geography of Korea. Suwon, Republic of Korea: Ministry of Land, Transport and Maritime Affairs.
- Nieuwolt, S. 1977. Tropical Climatology. New York: John Wiley & Sons.
- Park, S. 2014. “Regional Analyses of Aerosol Optical Thickness over South Korea.” The Geographical Journal of Korea 48: 523–532.
- Rahimia, S., M. A. G. Sefidkouhia, M. Raeini-Sarjaza, and M. Valipourb. 2015. “Estimation of Actual Evapotranspiration by Using MODIS Images (A Case Study: Tajan Catchment).” Archives of Agronomy and Soil Science 61 (5): 695–709.
- Rao, C. R. N., E. P. McClain, and L. L. Stowe. 1989. “Remote Sensing of Aerosols over the Oceans Using AVHRR Data: Theory, Practice, and Applications.” International Journal of Remote Sensing 10: 743–749.
- Remer, L. A., Y. J. Kaufman, D. Tanré, S. Mattoo, D. A. Chu, J. V. Martins, R. R. Li, et al. 2005. “The MODIS Aerosol Algorithm, Products and Validation.” Journal of Atmospheric Science 62: 947–973.
- Remer, L. A., R. G. Kleidman, R. C. Levy, Y. J. Kaufman, D. Tanré, S. Mattoo, J. V. Martins, et al. 2008. “Global Aerosol Climatology from the MODIS Satellite Sensors.” Journal of Geophysical Research 113: D14S07. doi:10.1029/2007JD009661.
- Remer, L. A., D. Tanré, Y. J. Kaufman, C. Ichoku, S. Mattoo, R. Levy, D. A. Chu, et al. 2002. “Validation of MODIS Aerosol Retrieval over Ocean.” Geophysical Research Letter 29: 321–324.
- Richter, A., J. P. Burrows, H. Nüß, C. Granier, and U. Niemeier. 2005. “Increase in Tropospheric Nitrogen Dioxide over China Observed from Space.” Nature 437: 129–132.
- Shin, S., and K. Lee. 2016. “Trend Analysis of Wildland Fires and Their Impacts on Atmospheric Environment over East Asia.” Asian Journal of Atmospheric Environment 10 (1): 22–31.
- Song, C. K., C. H. Ho, R. J. Park, Y. S. Choi, J. Kim, D. Y. Gong, and Y. B. Lee. 2009. “Spatial and Seasonal Variations of Surface PM10 Concentration and MODIS Aerosol Optical Depth over China.” Asia-Pacific Journal of Atmospheric Sciences 416: 33–43.
- Sophie, B., D. Pierre, V. B. Eric, O. Arino, V. Kalogirou, and J. R. Perez. 2010. “GLOBCOVER 2009: Products Description and Validation Report.” UCLouvain and ESA, Accessed 14 February 2016. http://due.esrin.esa.int/files/GLOBCOVER2009_Validation_Report_2.2.pdf
- Streets, D. G., T. C. Bond, G. R. Carmichael, S. D. Fernandes, Q. Fu, D. He, Z. Klimont, et al. 2003. “An Inventory of Gaseous and Primary Aerosol Emissions in Asia in the Year 2000.” Journal of Geophysical Research 108 (D21): 8809. doi:10.1029/2002JD003093.
- Takemura, T., T. Nakajima, T. Nozawa, and K. Aoki. 2001. “Simulation of Future Aerosol Distribution, Radiative Forcing, and Long-Range Transport in East Asia.” Journal of the Meteorological Society of Japan 79: 1139–1155.
- Valipour, M. 2014a. “Comparative Evaluation of Radiation-Based Methods for Estimation of Potential Evapotranspiration.” Journal of Hydrologic Engineering 20 (5): 04014068.
- Valipour, M. 2014b. “Prediction of Irrigated Agriculture in Asia Pacific Using FAO Indices.” Acta Advances in Agricultural Sciences 2 (9): 40–53.
- Valipour, M. 2015b. “Evaluation of Radiation Methods to Study Potential Evapotranspiration of 31 Provinces.” Meteorology and Atmospheric Physics 127 (3): 289–303.
- Valipour, M., and S. Eslamian. 2014. “Analysis of Potential Evapotranspiration Using 11 Modified Temperature-Based Models.” Internal Journal of Hydrology Science and Technology 4 (3): 192–207.
- Valipoura, M. 2015a. “A Comprehensive Study on Irrigation Management in Asia and Oceania.” Archives of Agronomy and Soil Science 61 (9): 1247–1271.
- Valipoura, M. 2015c. “Investigation of Valiantzas’ Evapotranspiration Equation in Iran.” Theoretical and Applied Climatology 121 (1): 267–278.
- Valipoura, M. 2015d. “Study of Different Climatic Conditions to Assess the Role of Solar Radiation in Reference Crop Evapotranspiration Equations.” Archives of Agronomy and Soil Science 61 (5): 679–694.
- Wang, J., and S. A. Christopher. 2003. “Intercomparison between Satellite-Derived Aerosol Optical Thickness and PM2.5 Mass: Implications for Air Quality Studies.” Geophysical Research Letters 30: 2095. doi:10.1029/2003GL-018174.
- Wang, M. X., R. J. Zhang, and Y. F. Pu. 2001. “Recent Researches on Aerosol in China.” Advances in Atmospheric Sciences 18: 576–586.
- Wong, M. S., J. E. Nichol, and K. H. Lee. 2009. “Modeling of Aerosol Vertical Profiles Using GIS and Remote Sensing.” Sensors 9: 4380–43.
- Yan, X., T. Ohara, and H. Akimoto. 2006. “Bottom-Up Estimate of Biomass Burning in Mainland China.” Atmospheric Environment 40: 5262–5273.