ABSTRACT
In 2014 and 2015, Ohio vineyards were exposed to multiple freeze events of –20 °C or lower, resulting in vine dieback, i.e., complete damage of above ground parts in Vitis vinifera. Grapevines that sustained dieback were rehabilitated for trunk replacement by training 1-year-old shoots with two distinct morphologies, based on internode diameter of large (L) and normal (N). This study evaluated the impact of cane morphology (L and N) in V. vinifera Cabernet franc on freezing tolerance (FT) of bud, phloem, and xylem tissues in relation to their respective anatomical structures and carbohydrate concentrations. Compared to N canes (7–9-mm diameter), L canes (10–15 mm) in Cabernet franc were considered vigorous and had the following morphological characteristics: long and heavy, with long and wide internode, and presence of numerous laterals. Furthermore, cane anatomy was also different with L canes having a significantly higher number of vascular transport units, xylem vessels, and phloem fibers than those in N canes. Freezing tolerance of buds and phloem was also different between the two cane types, with L canes being more cold sensitive than N canes, especially during fall acclimation and late-winter deacclimation. Sugar concentrations, however, were not different between L and N canes. These results suggest that cane morphology and anatomy play a significant role in affecting FT and the large and abundant anatomical structures of phloem and xylem contributed to the reduced FT of these tissues. In all practicality, this study suggests the best cultural practice for trunk replacement, and vine recovery should include the removal of the undesirable vigorous and cold sensitive canes during pruning.
Introduction
Ohio has a thriving grape and wine industry that ranks among the top 10 states in the United States in grape acreage, grape production, and number of wineries. However, the sustainability and expansion of this industry is limited by climatic constraints, primarily seasonal freezing temperatures. Freezing damage is the leading environmental stress that causes crop loss to the grape industry in Ohio and surrounding states (Zabadal et al., Citation2007). Ohio grape growers experienced major consecutive freezing damage episodes over the last 10 years with unprecedented crop and vine losses valued at $12 million following the “polar vortex” event in 2014 (Dami et al., Citation2014; Dami and Lewis, Citation2014).
In order to mitigate freezing damage in grapevines, various protection methods have been developed and implemented prior to, during, or after a freezing event, with the purpose of improving the freezing tolerance (FT) or recovery of grapevines (Howell, Citation1988; Poling, Citation2008; Pool and Howard, Citation1985). Managing grapevines after winter damage is an important practice to accelerate vine recovery and resume normal production in subsequent years. Trunk renewal, by retraining 1-year-old canes, is a key cultural practice following freezing injury. In Jan. and Feb. 2014, there were five freezing events of air temperature below –20 °C (–20.1 to –31 °C) at The Ohio State University research vineyard. These extreme and repeated sub-freezing events resulted in dieback, i.e., complete damage (100%) of above-ground vine parts, including buds, canes, and trunks of all V. vinifera cultivars (Dami et al., Citation2016). Only buds at the base of trunks and protected by soil hilling survived ( and ). The practice of soil hilling (covering the graft union with soil) protects the buds at the base of the scion for trunk renewal (Pool and Howard, Citation1985; Zabadal et al., Citation2007). New shoots matured to canes, during the 2014 growing season, were again exposed to nine freezing events of air temperature below –20 °C (–20.3 to –22.8 °C) in Jan. and Feb. 2015. As a result, 1-year-old buds and canes were damaged except for the basal buds protected by soil hilling. These back-to-back damaging events presented an opportunity to conduct a trunk renewal study to provide research-based guidelines on optimum cultural practices for vine recovery. The purpose of this research was to evaluate FT or cold hardiness, carbohydrate metabolism, and internal structures (anatomy) in dormant grapevine canes with different morphology (cane size). The central hypothesis was that cane FT was impacted by cane morphology, anatomy (internal structures), and carbohydrate (sugar) concentration. The central hypothesis of this research was tested by pursuing the following specific research objectives: (1) Determine morphological characteristics of cane with different sizes, based on cane diameter. (2) Evaluate the internal (anatomical) structures, FT, and carbohydrates of cane vascular tissues at various stages of cold acclimation in relation to cane morphology.
Figure 1. Trunk renewal of severely freeze-damaged Cabernet franc grapevines. (A) Vine dieback (100% bud, cane, cordon, and trunk damage) with new shoots emerging in the spring. (B) Fan-trained shoots in mid-summer. Note dead trunks and cordons were removed. (C) Mature shoots (canes) in the fall with two distinct internode sizes, medium or normal (N) and large (L) or bull. (D) Two-node cuttings showing cane size based on internode diameters and compared to pencil size.
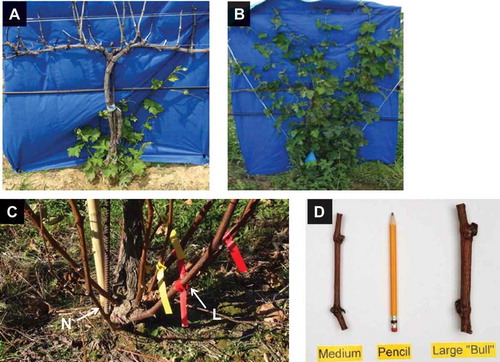
Materials and methods
Plant material and treatments
This study was conducted at the Ohio State University research vineyard in Wooster (40.7384 latitude and –81.9032 longitude, elevation, 355 m asl; soil series, Riddles silt loams) in northeast Ohio (USDA Plant Hardiness Zone 6a [–23.3 to –20.6 °C]; ARS-USDA 2012). Vitis vinifera Cabernet franc clone FPS11 grafted onto V. riparia × V. rupestris 101-14 were planted in 2010 in a north-south orientation and vines were spaced 1.8 m × 2.7 m (vine × row). Grapevines were trained to bilateral cordons (1.05-m high) with vertical shoot positioning (also known as VSP) during the growing season (), and spur pruned to 15 buds per meter of cordon during the dormant season. Following the freeze events in 2014 and 2015 and vine dieback, newly emerged shoots at the base of trunks and above the graft union were trained to a fan system (; Zabadal et al., Citation2007). These shoots had primarily two sizes, large (L) and normal (N). This study used 25 grapevines with L and N shoots/canes for trunk replacement. On 6 Aug. 2015, shoot/cane size was determined with a caliper (Mitutoyo-Digimatic Caliper, Kawasaki, Japan) by measuring the diameter of internodes 2 (cane tissue between node 2 and 3) and 3 (cane tissue between node 3 and 4), which averaged 13 mm (10–15 mm) in L and 8 mm (7–9 mm) in N ( and ).
Weather conditions during the study
Hourly maximum and minimum temperatures (°C) were recorded throughout the duration of the study using a temperature logger (Watchdog Model #A110, Spectrum Technologies, Aurora, IL, USA) installed at the north end of the vineyard. Temperature data were downloaded monthly to a laptop computer, and both the fall frost date (first occurrence of temperature below 0 °C in the fall) and total number of frost-free days (FFD; number of successive days with a minimum daily temperature at or above 0 °C) were determined from the temperature logger data. Growing degree-days (GDD in °C) represents the cumulative heat units during the growing season, and was calculated by adding the daily mean temperature above 10 °C from 1 Apr. through 31 Oct.
Vegetative growth and cane morphology and anatomy
Vegetative growth and cane morphology
During the 2015 season, vine vigor was assessed by measuring the following vegetative growth characteristics in L and N shoots/canes including: total L and N shoots per vine that emerged above the graft union, main and lateral shoot length between node 2 and 12, and internode length (shoot length/node number) and weight. Pruning weight per vine was also determined by adding the weights of all canes collected for freezing tests (see below) throughout the dormant season and those removed after final vine pruning in March 2016. During pruning, grapevines were trained back to the bilateral cordon system by retaining two 2-year-old trunks and one to three 1-year-old canes as spare parts for future trunk replacement.
Cane anatomy
From 6 Aug. 2015 to 28 Mar. 2016 (six collections), internode 2 (cane tissue between node 2 and 3) and internode 3 (cane tissue between node 3 and 4) were sampled for anatomical analysis from the L and N canes. On each collection date, the internode tissue was excised by hand into cross sections (5-mm thick) using a razor blade and stored in a vial containing water (25 mL) at 4 °C. Cross-section samples were then transferred to a glass petri dish and submerged in 70% ethanol for light microscopy examination (Leica model S6D, Wetzlar, Germany) within 1 week from field collection. Images of internode cross sections were taken using a digital camera (Leica model MC170 HD, Wetzlar, Germany) mounted on the microscope. Digital images were collected and analyzed using ImageJ software (National Institute of Health, Bethesda, MD, USA) to quantify anatomical structures including surface areas of pith, phloem, and xylem; vascular transport units (VTU; defined as the area bordered by adjacent xylem and phloem rays [see ]), and the number of xylem vessels and phloem fibers per VTU.
Figure 2. Cross section of internode cane tissues with large and normal sizes in Cabernet franc grapevines collected on 23 Nov. 2015: (A) large cane (3× magnification), (B) normal cane (3× magnification), and (C) large cane (200× magnification). The solid lines delineate the boundaries of a vascular transport unit (VTU), which consists of a phloem VTU and xylem VTU. The letters “v” and “f” indicate xylem vessel and phloem fiber, respectively.
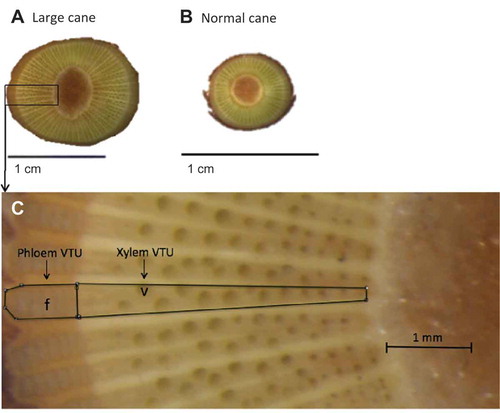
Freezing tolerance
Canes were collected monthly from the vineyard between Aug. 2015 and Mar. 2016 corresponding to the same collection times for anatomical analysis. On each collection date, 15 canes of each size (L and N) were randomly sampled, placed in plastic bags to prevent moisture loss, and brought to the laboratory. Bud and cane tissues between node 4 and 12 were used for freezing tests. The FT of bud (primary, secondary, and tertiary) and cane (phloem and xylem) tissues was determined based on the oxidative tissue browning method (Zabadal et al., Citation2007). Briefly, L and N canes were kept in two separate groups then excised into two-node cuttings. Each group of cuttings was randomly pooled into three equal sub-groups (replications) and wrapped in moist cheesecloth, then covered in Aluminum foil. A thermocouple was attached to the surface of the cane internode to monitor cane temperature within each casing. The casings were placed overnight in a refrigerator at 4 °C. The foil casings were then placed into a programmable Environmental Chamber (Thermal Products Solutions, New Columbia, PA, USA). The environmental chamber was programmed to lower the temperature at a rate of 4 °C/h from –2 °C to a target lowest (killing) temperature that varied with time of collection. Each casing was removed from the chamber at targeted thermocouple readings and at 2 °C increments, kept at 4 °C overnight, then placed at room temperature for 48 h before the bud and cane tissue assessment was conducted. A cross-section cut into the bud exposed the bud tissues (primary, secondary, and tertiary) for visual injury assessment. Using a pair of pruners, a cross-section cut of the internode was made to expose the phloem and xylem for visual injury assessment. Bud, phloem, and xylem tissues that maintained green color after freezing were recorded as alive, while any brown tissue observed was recorded as injured. The percent damage of each tissue was computed by dividing the number of damaged samples by the total number of samples and multiplying by 100 at each target temperature. Using the damage percentage at each target temperature, and curve fitting method (Sigmaplot, San Jose, CA, USA), the temperature that corresponded to 50% injury was recorded as LT50. Furthermore, on each collection an additional set of 2-node cuttings (control) were kept at 4 °C and evaluated to account for field damage and adjust LT50 accordingly.
Soluble sugars
Canes were collected monthly from the vineyard between Aug. 2015 and Mar. 2016, corresponding to the collection times for anatomical analysis and freezing test determination. A segment (3–5 cm long) of internode 2 (cane tissue between basal node 2 and 3) and internode 3 (between basal node 3 and 4) located at the proximal end of the cane at trunk base were sampled from the L and N canes and stored at –80 °C until further analysis. After bark tissue removal, the internode samples were ground in liquid nitrogen using a ‘Cryomill a11 basic’ (IKA Company, Wilmington, NC, USA). The samples were freeze-dried then extracted three times in 75% ethanol, with lactose included in the extraction buffer as an internal standard. Extracts were combined and samples were concentrated under nitrogen gas. Samples were re-dissolved in water and filtered through a 0.2-μM nylon filter. The concentrations of soluble sugars in cane internodes were quantified using a free zonal capillary electrophoresis (Zhao et al., Citation2016) and replicated four times.
Statistical analysis
Statistical analyses of cane morphology and anatomy, FT (LT50), and soluble sugars were conducted using a paired T-test at p < 0.05. Pearson analysis was used to determine the correlation coefficients between total sugars in the internode tissue and phloem LT50.
Results
Weather
Hourly minimum and maximum air temperatures from 1 Jan. 2015 to 30 Apr. 2016 are summarized in . The lowest temperature recorded was –22.8 °C on 20 Feb. 2015 and –18.7 °C on 8 Jan. 2016. The 2015 growing season had the following characteristics: last spring frost occurred on 6 Apr. 2015 and the first fall frost occurred on 17 Oct. 2015, resulting in 196 FFD and accumulating 1693 GDD.
Vegetative growth and cane morphology and anatomy
Vegetative growth and cane morphology
The mean number of shoots/canes per vine, which emerged at the base of trunks and above the graft union, was 10 (range of 6 to 22) and consisted of 60% L and 40% N canes (). Shoots grew nearly 4.3 m long (3–4.6 m) and possessed 40 nodes per shoot (35–45 nodes). Shoots and internodes in L were 20% longer than those in N ( and ). Lateral shoots were present in both cane types, but more abundant (two-fold more total laterals, and six-fold more laterals >30 cm) in L than in N canes (). Internodes were 188% heavier in L than in N canes (). At harvest, newly trained shoots produced a modest crop that averaged 10 clusters/vine and a yield of 0.23 kg/vine. The pruning weight per vine averaged 2.3 kg (or 1.28 kg per meter of cordon) with L canes weighing 123% heavier than N canes ().
Figure 4. Vegetative growth characteristics of shoots/canes with large and normal sizes in Cabernet franc grapevines: (A) number of shoots per vine, (B) shoot length between node positions 2 and 12 (measured on 6 Aug. 2015), (C) internode length between two consecutive nodes (6 Aug. 2015), (D) number of laterals per shoot between node positions 2 and 12 (6 Aug. 2015), (E) weight of two-node cutting (averaged over multiple collections between Oct. 2015 and Mar. 2016), and (F) pruning weight per vine. Means ± SE with different letters between L and N are significantly different by T-test at P < 0.05.
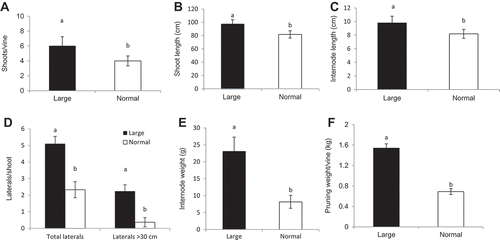
Cane anatomy
Samples for anatomical characterization were collected six times between Aug. 2015 and Mar. 2016. There was no change in parameters measured () in either cane type during that time period. Therefore, the results presented are means of measurements taken in all six collections. The surface areas of pith, xylem, and phloem of the L canes were two- to three-fold larger than those in N canes (). The surface area of xylem VTU was nearly four-fold larger in L than in N canes (). Similarly, the surface area of phloem VTU was two-fold larger in L than in N canes (). Furthermore, the VTUs per cross section were more numerous (22% more) in L than in N canes (). The number of xylem vessels located within each VTU was 47% higher in L than in N canes. The number of phloem fibers located within each VTU was also 25% higher in L than in N canes ().
Figure 5. Cross-section anatomical characteristics of cane internodes with large and normal sizes in Cabernet franc grapevines: (A) surface area of pith, xylem, and phloem; (B) vascular transport unit (VTU) area of xylem and phloem; (C) vascular transport unit (VTU) number; and (D) number of xylem vessels and phloem fibers within each VTU. Means ± SE with different letters are significantly different by T-test at P < 0.05.
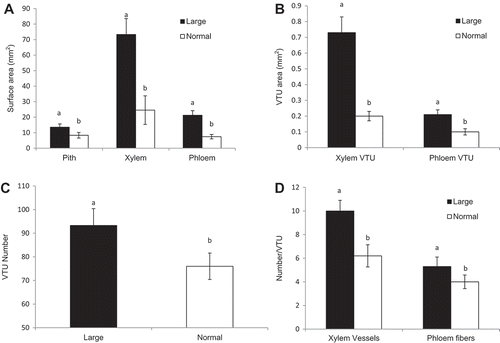
Freezing tolerance
The FT of primary, secondary, tertiary, and phloem tissues followed the typical pattern of cold acclimation-deacclimation in grapevines during the dormant season. In October, LT50 of all four tissues ranged between –7.8 °C (primary bud in L) and –10.6 °C (phloem in N). In December, LT50 decreased more than two-fold since October and ranged between –18.3 °C (secondary bud in L) and –21.9 °C (tertiary bud in N) and remained at its lowest level through February, when all tissue began to deacclimate. By the end of March, LT50 increased (FT decreased) and reached LT50 values of –7.8 °C (primary bud in L) to –14 °C (phloem in N; ). In October, LT50 of all tissues was not different between L and N canes. However, N canes had lower primary bud LT50 than L canes in November and December (). N canes also had lower secondary and tertiary bud LT50 than L canes in December ( and ). In March, all four tissues had lower LT50 in N than in L (). Phloem LT50 was lower in N than in L canes in December and March (). In this study, the FT of xylem was not determined since the lowest target temperature used was not cold enough to kill 50% of the tissues. In fact, at the lowest target temperature of –24 °C, xylem injury was only 27% in L and 10% in N on 23 Feb. 2015. It is also noted that there was no damage in any control canes (not subjected to freezing test) from all collections except in February, when primary buds sustained similar injury in L and N canes of 3%. Furthermore, among the four tissues tested throughout the dormant season, xylem had the highest FT (lowest LT50) followed by, in order of decreasing FT, phloem, tertiary, secondary, and primary buds.
Soluble sugars
In all collection dates, the following soluble sugars were identified in the internode tissue, fructose, galactinol, glucose, myo-inositol, raffinose, sucrose, and stachyose (). Due to their predominant concentration and importance in grapevines, only fructose, glucose, raffinose, sucrose, and stachyose are presented. The total and individual soluble sugars followed the typical pattern of seasonal changes during the cold acclimation-deacclimation in grapevines. In fact, sugars were at their lowest concentration in August and then increased steadily during fall acclimation (October–December), reached maximum concentration in mid-winter (January–February), then decreased to the lowest level in March (). The total sugar concentration doubled in January as compared to that in August (); and among individual sugars, raffinose and stachyose had the highest concentration increase of 17- and 11-fold, respectively ( and ). When comparing individual sugar concentrations from October to January, fructose had the largest increase in concentration (53-fold) followed by glucose (30-fold; see and ) and sucrose had the smallest increase (two-fold; Figure 7D).
Figure 7. Seasonal changes (8 Oct. 2015 to 28 Mar. 2016) of sugar concentrations (mg/g DW) in large and normal canes in Cabernet franc grapevines: (A) total sugars, (B) glucose, (C) fructose, (D) sucrose, (E) raffinose, and (F) stachyose. Sugars were extracted from internode tissue between node 1 and 2. *, **, *** indicate significant difference at P < 0.05, 0.01, 0.001, respectively.

When comparing total and individual sugars between canes of different sizes, we found that only in August, N canes had significantly higher concentrations of glucose and fructose than those in L canes. However, no differences of sugar concentrations were found on any other date ( and ). Although the total soluble sugar concentration did not differ between cane size, a very significant negative correlation (R = –0.983, p < 0.001) was found between phloem LT50 and soluble sugar concentrations.
Discussion
The extent of cold injury in grapevines varies with the severity of the freeze event (lowest temperature and duration of exposure) and the FT status of the genotype and its plant parts at the time of event occurrence (Zabadal et al., Citation2007). In this study, we confirmed the differences of FT between vine part organs with primary buds being the most cold sensitive and xylem the least to freeze stress and subsequent injury (Zabadal et al., Citation2007). Therefore, cold damage is often assessed first in dormant buds (typically primary and secondary). Following winter injury, vine recovery management strategy involves an adjustment of pruning and training practices with the goal to re-establish vine structure and resume pre-damage production. If freeze injury is restricted to buds, the recovery of crop production can be achieved by increasing the number of buds retained after pruning in proportion to the level of bud injury (Zabadal et al. Citation2007). It has been demonstrated that pruning adjustment after cold injury led to a near normal crop in several grape cultivars (Dami et al., Citation2012; Keller and Mills, Citation2007; Wolfe, Citation2000).
The 2014 and 2015 polar vortex freeze events (14 events of air temperature below –20 °C with an extreme of –31 °C) were severe and caused trunk damage in Cabernet franc. In this situation, vine recovery was accomplished by retraining new shoots for trunk replacement. The low yield (low sink) combined with high vegetative growth (high source) of recovering vines led to an imbalance between vegetative and reproductive growth including the emergence of highly vigorous “bull” shoots. In order to restore growth balance, grapevines are managed differently by training multiple shoots (called suckers) from the trunk base on a fan-like shape, thus the name, fan training system (Wolfe, Citation2000; Zabadal et al., Citation2007). Despite training multiple shoots (averaging 10 shoots per vine) and converting Cabernet franc from VSP to fan training system, vines still produced 60% of L shoots. We measured several parameters to characterize vine vigor, including shoot length, internode length and diameter, and pruning weight. The L shoots were long, heavy with long internode and wide diameter, and possessed long and numerous laterals. The abundance of lateral shoot development in L canes is an indication of vigor and has been reported to be directly proportional to the vigor of the main shoot (Dry and Loveys, Citation1998). Therefore, it is concluded that the L shoots are considered excessively vigorous with similar vegetative and morphological characteristics reported previously (Byrne and Howell, Citation1978; Howell and Shaulis, Citation1980; Ru, Citation2011).
Excessive cane vigor leads to increased cold damage (Byrne and Howell, Citation1978; Howell and Shaulis, Citation1980). The current study confirmed this observation using controlled freezing tests and showed differences in LT50 as high as 5 °C between L and N canes. The repeated measurements of LT50 throughout the dormant season also showed that L canes were less cold hardy than N canes, particularly during acclimation (corresponding to tissue dehydration in fall) and deacclimation (tissue rehydration in late winter-early spring), but both had similar FT in mid-winter (January). It is possible that the compartmentalization of extra-(free) and intra-cellular (bound) water during these transition stages was not complete and/or altered in L canes (due to a more complex vascular system) leading to the presence of more freezable water thus cold injury (Levitt, Citation1980; Sakai and Larcher, Citation1987). Even though water content in the internode was similar in both L and N canes (data not shown), it was not measured in specific tissues to account for water content and distribution between phloem, xylem, and bud tissues. It is also known that grape buds supercool to avoid intracellular freezing, whereas cane and trunk cells tolerate freezing temperatures by intracellular dehydration (Jones et al., Citation1999). Even though bud and cane tissue have different FT mechanisms, both tissues sustained extensive freeze damage in L canes.
In order to advance our understanding of the reasons behind the substantial difference of FT between L and N canes, we characterized the anatomical and biochemical (sugars) responses in respective cane type. The anatomical features of L canes were significantly and consistently different than those in N canes. The L canes possessed a significantly higher number of VTU, xylem vessels, and phloem fibers than those in N canes. The xylem VTU occupied cross-sectional areas four-fold larger in L than in N canes. Schubert et al. (Citation1999) reported a positive relationship between increased xylem vessels and shoot length (vigor). Nevertheless, it seems counterintuitive that vines produce large shoots with large anatomical structures, which may jeopardize their survival the following winter. One possible explanation for this phenomenon is that the anatomical structures of L canes in grapevine, which is a liana (climbing woody plant), were built as a mechanism to escape from biotic or abiotic stress (in this case freezing stress) near the ground and transport a large amount of water and nutrients (increased vessel number) long distance (long shoots) with a strong support system (increased VTU and fibers) by climbing over trees to ensure the production of seeds for procreation at a more distal location. These characteristics are adaptive strategies for efficient capacity of water conducting in angiosperms (Taiz and Zeiger, Citation2002; Tyree and Zimmermann, Citation2002). Other possible explanations of the phenomenon of increased freeze injury associated with plant anatomy are proposed as follows. First, the increased number of xylem and phloem VTU (tissues/cells) may also increase the sites of ice nucleation thus injury (Levitt, Citation1980; Sakai and Larcher, Citation1987). Second, L canes might have increased cavitation, which was reported to increase the susceptibility of xylem with large vessels to freezing injury (Tyree et al., Citation1994). Third, the large tissue/cell size may have contributed to their inability to contract, which is a critical process of cell dehydration during cold acclimation, which may have led to increased freeze injury in L canes (Levitt, Citation1980; Pearce and Ashworth, Citation1992; Singh and Miller, Citation1985).
In this study, the seasonal changes of soluble sugars in Cabernet franc proceeded as expected and concur previous reports (Grant and Dami, Citation2015; Hamman et al., Citation1996; Jones et al., Citation1999). In fact, soluble sugar concentrations increased in the fall when Cabernet franc acclimated in response to low temperatures, reached the maximum level during the coldest months in mid-winter, and decreased in the spring during deacclimation. Soluble sugars have been implicated in the FT of herbaceous and woody plants including grapes; the correlation between sugar accumulation and increased FT is both qualitative and quantitative (Grant and Dami, Citation2015; Sakai and Larcher, Citation1987). Furthermore, a negative correlation between phloem LT50 and soluble sugar concentrations was also found and agrees with previous reports (Hamman et al., Citation1996, Grant and Dami, Citation2015; Grant et al., Citation2013). Possible roles reported for sugars in relation to FT included enhancing supercooling, preventing or slowing crystallization of ice, and increasing structural stability of cell membranes (Sakai and Larcher, Citation1987). In this study, due to the difference of FT between L and N canes, it was hypothesized that sugar levels would also be different between the two cane types; however, it was not the case. We attribute this finding to the lack of specificity of the tissue analyzed. In fact, soluble sugars were extracted from cane tissues (i.e., xylem, phloem, cambium, and pith combined), but not from buds. Even though the sugar concentration per dry weight of a cane segment was not different, it is possible that the partitioning of specific sugars is different between L and N; thus, the sugar concentration would be different if analyzed in a specific tissue (e.g., buds or phloem/cambium tissue only). We predict sugar levels in buds to be lower in L than in N canes. However, this hypothesis needs further investigation. The only significant difference of sugar concentration occurred in early August with N cane tissue having higher glucose and fructose than L cane tissue. There was a significant level of glucose and fructose in August then a sudden drop between August and October. In August, shoots were still green and did not mature (formed periderm) yet. Therefore, we attribute the sudden August “surge” of fructose and glucose to the photosynthetic nature of the internode tissues.
In summary, this study confirmed the increased freezing injury of L (bull) canes as compared to N canes in Cabernet franc grapevines when exposed to similar subfreezing temperatures. It was also confirmed that vigorous shoots/canes, which correspond to the L canes in this study, have the following morphological characteristics: long and heavy canes with long and wide internode, and presence of numerous laterals. Among the three main parameters characterized in internode tissues, FT and anatomical structures, but not sugars, exhibited the most significant changes. We, therefore, conclude that the large and abundant anatomical structures of phloem and xylem contributed to the reduced FT of these tissues. The new knowledge gained about the influence of cane morphology on freezing tolerance of cane and bud tissues in Cabernet franc could be extrapolated to other cultivars, especially vigorous ones. However, the findings about the sugar level were not conclusive. This can be addressed in future studies by measuring sugar concentrations in specific tissues, such as buds. The findings of this study have also practical applications that are beneficial to grape growers. In order to mitigate the vicious cycle of excessive vigor—bull cane production—and subsequent cold injury, cane selection for trunk renewal and vine recovery after cold damage is critical. Leaving multiple shoots (>4) provides the opportunity to select canes of normal size for trunk replacement and to remove the undesirable vigorous and cold sensitive canes. In addition to the impact on trunk renewal, cane morphology would also influence the fruitfulness and winter survival of canes and spurs retained after pruning.
Acknowledgments
We thank Joshua Blakeslee and Ann Channon for carbohydrate analysis, and Tea Meulia at the Molecular and Cellular Imaging Center for advice and assistance in sample preparation for anatomical studies. We also thank Martin Goffinet, Emeritus at Cornell University, for his advice on anatomical measurements and quantification. We are grateful to Bruce Williams and Logan Walter for vineyard and field maintenance.
Funding
This research was funded by the Department of Horticulture and Crop Science, USDA/NIFA Specialty Crops Research Initiative Award Number 2010-51181-21599 USDA-NIFA, and Ohio Grape Industry Program.
Additional information
Funding
Literature cited
- Byrne, M.E., and G.S. Howell. 1978. Initial response of Baco noir grapevines to pruning severity, sucker removal, and weed control. Amer. J. Enol. Viticult. 29:192–198.
- Dami, I.E., D. Kinney, and S. Li. 2014. Polar vortex and its impact on grapes. Ohio Grape-Wine Electronic Newsletter. 10 Jan., Issue: 2–6.
- Dami, I.E., and D. Lewis. 2014. 2014 Grape winter damage survey report HCS Series #816. Dept. Hort. Crop Sci., Ohio State Univ., OARDC, Wooster. 16 May 2014. <http://hdl.handle.net/1811/60447≥.
- Dami, I.E., S. Ennahli, and Y. Zhang. 2012. Assessment of winter injury in grape cultivars and pruning strategies following a freezing stress event. Amer. J. Enol. Viticult. 63:106–111.
- Dami, I.E., S. Li, and Y. Zhang. 2016. Evaluation of primary bud freezing tolerance of twenty-three winegrape cultivars new to the eastern United States. Amer. J. Enol. Viticult. 67:139–144.
- Dry, P.R., and B.R. Loveys. 1998. Factors influencing grapevine vigour and the potential for control with partial rootzone drying. Aust. J. Grape Wine Res. 4:140–148.
- Grant, T.N., and I.E. Dami. 2015. Physiological and biochemical seasonal changes in Vitis genotypes with contrasting freezing tolerance. Amer. J. Enol. Viticult. 64:195–202.
- Grant, T.N., J. Gargrave., and I.E. Dami. 2013. Morphological, physiological, and biochemical changes in Vitis genotypes in response to photoperiod regimes. Amer. J. Enol. Viticulture. 64:466–475.
- Hamman, R.A., I.E. Dami, T.M. Walsh, and C. Stushnoff. 1996. Seasonal carbohydrate changes and cold hardiness of chardonnay and Reisling grapevines. Amer. J. Enol. Viticult. 47:31–36.
- Howell, G.S. 1988. Cultural manipulation of vine cold hardiness. Proc. Intl. Symp. Cool Climate Viti. Enol., Auckland, New Zealand, Jan. 1988, pp. 98–102.
- Howell, G.S., and N.J. Shaulis. 1980. Factors influencing within-vine variation in the cold resistance of cane and primary bud tissues. Amer. J. Enol. Viticult. 31:158–161.
- Jones, K., J. Paroschy, B. Mckersie, and S. Bowley. 1999. Carbohydrate composition and freezing tolerance of canes and buds in Vitis vinifera. J. Plant Physiol. 155:101–106.
- Keller, M., and L.J. Mills. 2007. Effect of pruning on recovery and productivity of cold-injured Merlot grapevines. Amer. J. Enol. Viticult. 58:351–357.
- Levitt, J. 1980. Responses of plants to environmental stresses. Academic Press, New York, NY.
- Pearce, R.S., and E.N. Ashworth. 1992. Cell shape and localization of ice in leaves of overwintering wheat during frost stress in the field. Planta 188:324–331.
- Poling, E.B. 2008. Spring cold injury to wine grapes and protection strategies and methods. HortScience 43:1652–1662.
- Pool, R.M., and G.E. Howard. 1985. Managing vineyards to survive low temperatures with some potential varieties for hardiness. Proc. Intl. Symp. Cool Climate Viti. Enol., Corvallis, OR, 25–28 June 1985, pp. 184–197.
- Ru, S. 2011. Mechanistic view of shoot vigor in grapevines: Xylem anatomy, hydraulics and reproductive growth. Master’s thesis, Wash. State Univ., Pullman, Master’s thesis.
- Sakai, A., and W. Larcher. 1987. Frost survival of plants: Responses and adaptation to freezing stress (Ecol. Studies 62). Springer-Verlag, Berlin.
- Schubert, A., V. Novello, and E. Peterlunger. 1999. Shoot orientation affects vessel size hydraulic conductivity and shoot growth rate in Vitis vinifera L. Plant Cell Environ. 22:197–204.
- Singh, J., and W. Miller. 1985. Biophysical and ultrastructural studies of membrane alterations in plant cells during extracellular freezing: Molecular mechanism of membrane injury, p. 62–71. CRC Press Inc., Boca Raton, FL.
- Taiz, L., and E. Zeiger. 2002. Plant physiology, 3rd ed. Sinauer Associates Inc., Sunderland, MA.
- Tyree, M.T., K.J. Kolb, S.B. Rood, and S. Patino. 1994. Vulnerability to drought-induced cavitation of riparian cottonwoods in Alberta: A possible factor in the decline of an ecosystem? Tree Physiol. 14:455–466.
- Tyree, M.T., and M.H. Zimmermann. 2002. Xylem structure and the ascent of sap, 2nd ed., 283 p. Springer, Berlin.
- Wolfe, W. 2000. Vine and vineyard management following low temperature injury, p. 101–110. In J.M. Rantz (ed.). Proceedings of the ASEV 50th Anniversary Annual Meeting. American Society for Enology and Viticulture, Davis, CA.
- Zabadal, T.J., I.E. Dami, M.C. Goffinet, T.E. Martinson, and M.L. Chien. 2007. Winter injury to grapevines and methods of protection. Mich. State Univ. Ext. Bul. 2930.
- Zhao, L., A.M. Channon, N. Chattopadhyay, I.E. Dami, and J.J. Blakeslee. 2016. Quantification of carbohydrates in grape tissues using capillary zone electrophoresis. Front. Plant Sci. 7(818):1–14.