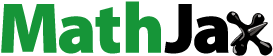
ABSTRACT
Mangifera indica (cv. East Indian) is a premium cultivar in Jamaica and the diaspora, attracting high market value through retail trade. Many Jamaicans prefer to consume tree-ripened East Indian mangoes, citing better taste compared to mangoes ripened during storage. This study was undertaken to evaluate if there was a difference in the biochemical qualities of East Indian mangoes based on whether they were tree ripened or detached from the tree. Quantitative determination of biochemical parameters such as total soluble solids, pH, percent acidity, reducing sugar content, total phenolics, and 2,2-diphenyl-1-picrylhydrazyl (DPPH) free radical scavenging activity were determined. No significant difference was found in the total soluble solids content of tree-ripened (21.93 ± 2.05) and postharvest-ripened mangoes (21.35 ± 1.86). There were however significant differences in the total reducing sugar (tree ripened, 4.50 ± 0.68 and postharvest ripened, 7.94 ± 0.8), titratable acidity (tree ripened, 0.15 ± 0.04 and postharvest ripened, 0.19 ± 0.03), pH (tree ripened, 5.47 ± 0.27 and postharvest ripened, 5.25 ± 0.12), total phenolics (tree ripened, 42.43 ± 5.73 mgGAE/100 g and postharvest ripened, 32.81 ± 5.64 mgGAE/100 g), and DPPH free radical scavenging activity (tree ripened, 49.34 ± 5.94 100 mg/ml and postharvest ripened, 38.84 ± 6.50 100 mg/ml) of the mangoes. These results indicate that there was a significant difference in the biochemical qualities between tree-ripened and postharvest-ripened mangoes, which invariably will contribute to differences in the taste of tree-ripened versus postharvest-ripened mangoes.
Introduction
Mangifera indica (mango) belongs to the Anacardiaceae family and is one of the most commercially cultivated and consumed fruit in tropical and subtropical regions of Africa, Asia, America, and the West Indies. In India and the Philippines, mango serves as the national fruit and is often referred to as the “king of fruits.” There are over 1,000 varieties of the fruit with some 40 species originating in Southeast Asia (Kiple and Ornelas, Citation2000). Forty-eight varieties of mangoes occur in the Cameroons with Palmer, Keitt, and Améliorée being the most popular (Kansci et al., Citation2008). Its distribution to Africa, South America, and the islands of the Pacific has largely been attributed to Portuguese trade routes with the fruit being later introduced to Central America by the Spaniards (Mukherjee and Litz, Citation2009). After the establishment of the fruit in South American countries such as Brazil, it is believed that it was carried to the West Indies sometime in the eighteenth century arriving in Jamaica about 1782 (Morton, Citation1987). More recently, the fruits have been cultivated in Sicily, an island located south of Italy (Lauricella et al., Citation2017).
Mangoes are climacteric fruits (Brecht et al., Citation2008) and have a distinct ripening phase characterized by several biochemical changes, particularly starch–sugar conversions, declining acidity, tissue softening, and color changes among others (Gill et al., Citation2017). During ripening, several cultivars undergo gluconeogenesis and the hydrolysis of starch to simple soluble sugars; sucrose, fructose, and glucose, the latter two being the primary nonreducing sugar components (Wongmetha et al., Citation2015). This conversion is largely responsible for the sweetening and softening of mangoes. Losses in acidity have also been reported in several cultivars (Wongmetha et al., Citation2015). The decrease in acidity is partly attributed to the utilization of organic acids as substrates for increased cellular respiration. Titratable acidity, based predominantly on citric acid, was shown to decrease, while pH values increased in Keitt mangoes (Medlicott and Thompson, Citation1985). These changes have profound effect on the taste of mangoes as acidity is primarily responsible for the sour or tart taste. During ripening, the type and quantity of the phenolic compounds also vary. One notable reaction is the hydrolysis of gallotannins to milder gallic acid (Brecht et al., Citation2008). Phenols are secondary metabolites in plants that are associated with astringency (Robichaud and Noble, Citation1990). Variations in the flavor profile of ripened mangoes are therefore expected.
The metabolic pathways and nature of these biochemical changes appear to be a function of numerous variables including cultivar, maturity stage at harvest, and existing environmental conditions such as temperature (Hossain et al., Citation2014). These topics remain controversial, and in the case of the prolific Jamaican East Indian cultivar, very little data exist on its biochemical changes during ripening on or detached from the parent plant. Considerable studies have however been conducted on other popular cultivars such as Keitt, Alphonso, and Tommy Atkins (Deshpande et al., Citation2017; Yeshitela et al., Citation2004).
Various methods have been employed to determine the maturity of mangoes (Zakaria et al., Citation2012; Jha et al., Citation2006; Jha et al. Citation2010). Harvesting at the right stage of physiological maturity will ensure that the fruit ripens normally while detached from the parent plant. Generally, nondestructive indicators are size, fullness of the shoulders, external color, and specific gravity (Jha et al., Citation2006). In Jamaica, size and color are the predominant maturity indices. This practice is made on the basis that a physiologically mature fruit would have attained full size and continue its normal development whether attached or detached from the plant (Brecht et al., Citation2008). Physiologically mature fruits are often similar in size to tree-ripened fruits. There is a noticeable difference in the taste of tree-ripened versus postharvest-ripened mangoes with consumers preferring tree-ripened mangoes. Postharvest-ripened mangoes have a less sweet taste in comparison to tree-ripened mangoes. This study was undertaken to evaluate the difference in biochemical properties of tree-ripened versus postharvest-ripened mangoes as these parameters will undoubtedly have an impact on the taste of the fruit. The total soluble solids (TSS), pH, percent acidity, reducing sugar content, total phenolics, and free radical scavenging activity of the mangoes were evaluated. The TSSs and reducing sugar composition will impact on the sweetness of the fruit, whereas the pH, percent titratable acidity, and phenolic content will impact on the tartness of the fruit. Mangoes are a good source of β-carotene and phenolics which are antioxidants (Morales et al., Citation2017). The free radical scavenging properties of the fruit were therefore also evaluated.
Materials and Methods
Sampling
Samples of East Indian mangoes (16 tree ripened and 16 green mature) were harvested by hand in Montpellier District, St. Thomas, Jamaica. Fully ripened mature mangoes (golden yellow and yellow-orange skin) were harvested 120 days after anthesis whereas mature-green mangoes of comparable size to tree-ripened mangoes were harvested 112 days after anthesis and allowed to further ripen in the dark at ambient temperature (27°C). The dimensions of the fruit were measured. After attaining full maturity, the mangoes were peeled and stored at −20°C until further analysis. Only wholesome mangoes which were free from pests and disease were selected for the study.
TSSs and pH Determination
The TSS which is also an indicator of fruit maturity was determined using an Extech Instrument Portable Refractometer. The pH of the pulp was measured at ambient temperatures using a calibrated Oakton pH meter.
Titratable Acidity
The mesocarp of the fruit (5 g) was homogenized with water (50 ml) and the mixture titrated against a standardized solution of 0.1 N sodium hydroxide using phenolphthalein as indicator. Results were reported based on percent citric acid.
Reducing Sugar Content
Total reducing sugar content was determined by the Lane–Eynon Method (Citation1923) with modifications. Extracts were diluted in volumetric flasks (100 ml) containing Carrez 1 (potassium hexa-cyanoferrate(II) trihydrate, 15 g/100 ml) and Carrez 2 (zinc sulfate heptahydrate, 30 g/100 ml) solutions (5 ml each). The mixture was allowed to stand overnight before filtering. The filtrate was treated with hydrochloric acid and boiled. The pH of the solution was increased to 6.95 with calcium carbonate and filtered. The clarified extract was titrated against equal portions of Fehling’s solutions A and B (5 ml) using methylene blue as indicator. The titrating mixture was boiled until the appearance of a red-orange end point. The concentration of reducing sugar was calculated utilizing an α-d-glucose calibration curve (y = −0.1073x + 38.814; R2 = 0.9839).
Total Phenolic Content
Mango mesocarp (2 g) was extracted with hot methanol solution (80%, 20 ml). Extracts were agitated (1 h) and filtered. Total phenolic content was determined according to the Folin–Ciocalteu (FC) method (Singleton et al., Citation1999). An aliquot of extract (0.5 ml) was added to diluted 2 N FC reagent (1:10) (2.5 ml) followed by addition of sodium carbonate solution (7.5%, 2 ml). The mixture was incubated in the dark (2 h) prior to reading the absorbance at 765 nm using a UV–visible spectrophotometer. Gallic acid was used to generate a standard calibration curve (y = 0.0125x + 0.0163; R2 = 0.9986).
2,2-Diphenyl-1-Picrylhydrazyl Radical Scavenging Assay
The 2,2-diphenyl-1-picrylhydrazyl (DPPH) assay was used to determine the free radical scavenging capacity of mango extracts according to the method of Brand-Williams et al. (Citation1995) with some modifications. Extracts were reacted with the stable DPPH radical (0.5 mM) in methanol. The reaction mixture consisted of extract (0.2 ml), absolute methanol (3 ml), and DPPH solution (0.3 ml). The mixture was vortexed and incubated in the dark (60 min). The absorbance was read at 517 nm. A mixture containing DPPH solution and methanol was used as blank. The radical scavenging capacity was calculated utilizing the following equation:
where Ab and As are the absorbance of the blank and sample, respectively.
Data and Statistical Analysis
All determinations were performed in duplicate and results expressed as mean ± one standard deviation. Microsoft Excel 2016 was used to analyze the data. The t-test (two-sample assuming equal variances) was utilized for statistical analysis of the data.
Results and Discussion
Mangoes are high yielding fruits varying in size, shape, color, texture, and flavor (Mukherjee and Litz, Citation2009). They are large stone fruits (drupe) containing an edible resinous mesocarp (flesh). The exocarp (skin) is leathery in texture with colors ranging from green, purple-red, orange, and yellow. The fruit is reported as having several medicinal properties which includes anti-inflammatory (Kim et al., Citation2016), antidiabetic (Gondi and Prasada Rao, Citation2015), and anticancer properties (Corrales-Bernal et al., Citation2014). These properties have been attributed to the presence of polyphenolic compounds (Masibo and He, Citation2008). The fruit may be eaten raw or processed to produce chutneys, juice, and sauces.
Physical Characteristics
Tree-ripened mangoes had an average length of 12 cm and a shoulder width of 7.5 cm (). The fruits exhibited a bright yellow/yellow-orange coloration upon ripening (). Mature-green mangoes displayed pronounced yellow spotting on the skin at harvest (). During the ripening of mature-green mangoes, there was an average weight loss of 32.91 ± 4.05 g which was expected due to increased biochemical activities such as respiration. As the mature-green mangoes ripened, a slight shriveling of the skin was observed which was due to moisture loss ().
Table 1. Mango weight and dimensions.
Biochemical Properties of Mangoes
During fruit ripening, many physiological changes occur in the fruit due to various enzymatic reactions. The biochemical properties of mangoes are influenced by several parameters such as fruit maturity and postharvest storage temperature. Mangoes are typically harvested prior to obtaining full maturity to reduce spoilage and waste. Proper postharvest handling procedures are therefore required for shelf-life extension. Biochemical properties investigated in the current study included TSS, titratable acidity, reducing sugars, and total phenolic content (Hossain et al., Citation2014).
Titratable Acidity and pH
During ripening, losses in acidity have been reported in numerous cultivars. This may be reflected as a change in the titratable acidity of the fruit. During respiration, organic acids are consumed resulting in a decline in acidity. Malic acid and citric acid are the main organic acids in Keitt mangoes. Titratable acidity based on citric acid was shown to decrease, with a corresponding increase in pH values in Keitt mangoes (Medlicott and Thompson, Citation1985). A significant difference was found between the titratable acidity and pH of tree-ripened (0.15 ± 0.04% and 5.47 ± 0.27) and postharvest-ripened mangoes (0.19 ± 0.03% and 5.25 ± 0.12). Titratable acidity was higher in the postharvest-ripened mangoes with a corresponding decrease in pH (). These findings indicate that postharvest-ripened mangoes may exhibit a tarter (sour) taste in comparison to tree-ripened mangoes.
Table 2. Comparison of biochemical characteristics of tree-ripened and postharvest-ripened mangoes.
The low percent titratable acidity for fully ripened mangoes is expected. This is partly attributed to the utilization of acids as substrates for respiration and oxidation. In Pakistani varieties such as Malda and Anwar Ratual, percent acidity decreased from 0.84 to 0.112 and 2.12 to 0.39 after 8 days, respectively (Elahi and Khan, Citation1973). A corresponding increase of pH from 3.62 to 5.60 and 3.10 to 4.30 was reported (Elahi and Khan, Citation1973). The titratable acidity of Ashwina hybrid mangoes was also found to decrease during storage and was higher in immature mangoes (Hossain et al., Citation2014). The overall low acidity of the mangoes is partly attributed to the utilization of acids as substrates for respiration (). Respiration is high in climacteric fruits such as mangoes. As the fruit matures, a decrease in the levels of ascorbic acid has also been reported which may be due to oxidation (Hossain et al., Citation2014).
TSSs and Reducing Sugar
The TSS content gives an indication of the sweetness of the fruit. During ripening, starch is converted to sucrose. There is also cell-wall degradation (Hossain et al., Citation2014). These reactions are catalyzed by amylase. Invertase is responsible for the conversion of sucrose to fructose and glucose. Fructose has a higher sweetness index compared to glucose. No significant difference was observed in the average percent TSSs of tree-ripened versus postharvest-ripened mangoes (21.93 ± 2.05% and 21.35 ± 1.86%, respectively). Similar values of 21.62% were reported for Ataulfo (Mexico) and 20.13 ± 0.01 for Amrupali (Bangladesh) (Palafox-Carlosa et al., Citation2012). The high TSS values suggest that East Indian mangoes are very sweet in taste (). In contrast, Ashwina hybrid mangoes are less sweet with a TSS value of 5 when mature and 15 when fully ripened (Hossain et al., Citation2014).
Although no significant difference was observed in the TSS of tree-ripened and postharvest-ripened mangoes, a significant difference was found in the reducing sugar content of the mangoes. The percent reducing sugar content of tree-ripened mangoes was lower (4.50 ± 0.68%) compared to postharvest-ripened mangoes (7.94 ± 0.8%). This difference could be attributed to the type of sugar accumulating during ripening. In Keitt varieties sucrose, a nonreducing sugar was found to be in greatest concentration and fructose, the predominant reducing sugar (Medlicott and Thompson, Citation1985). Similar TSS values in tree-ripened and postharvest-ripened mangoes suggest that sucrose (nonreducing sugar) could possibly be the major sugar accumulating during the ripening of mangoes attached to the parent plant while glucose and fructose (reducing sugars) may be present in higher concentrations in postharvest-ripened mangoes. The increased reducing sugar content could be partly attributable to degradation of nonreducing sugars during storage. The type of sugars accumulated and the quantities during ripening may be a function of cultivar, ripening, and storage conditions. In Ashwina hybrid mangoes, the total sugar and nonreducing sugar content were found to increase during storage (Hossain et al., Citation2014). TSS was also higher in the mature fruit (Hossain et al., Citation2014).
In the ripening of Alphonso mangoes, a reduction in starch content from 18% to 0.1% was reported with an associated rise in total soluble sugars from 1% to 15% (Yashoda et al., Citation2006). These findings support the long-established view that starch is the main substrate utilized to produce soluble sugars in ripened mangoes when detached from the parent plant. While these studies point to postharvest ripening, it is expected that starch is also the primary substrate in tree-ripened mangoes. This is expected as a physiologically mature fruit will continue its normal development whether attached or detached from the plant (Brecht et al., Citation2008).
Studies conducted on several cultivars indicate gluconeogenesis and the hydrolysis of starch to simple soluble sugars; sucrose, fructose, and glucose. It has been shown that starch disappearance was concomitant to a fivefold increase of sucrose content in the postharvest ripening of Keitt mangoes (Silva et al., Citation2008). This conversion is largely responsible for the sweetening and softening of mangoes as they ripen. The difference in the composition of reducing and nonreducing sugars would invariably impact on the perceived taste of the fruits.
Total Phenolic Content and DPPH Radical Scavenging Activity
Mangoes are rich in polyphenolic compounds. Polyphenols that have been identified in the fruit include mangiferin, gallic acid, gallotannins, quercetin, isoquercetin, ellagic acid, and β-glucogallin (Berardini et al., Citation2004, Citation2005). Investigation of various mango cultivars from Bangladesh (Fazli, Langra, Ashwina, Himsagor, and Amrupali) revealed that the Langra cultivar had highest phenolic content and antioxidant properties (Afifa et al., Citation2014). Most of the radical scavenging properties of mangoes have been ascribed to mangiferin which is a potent antioxidant. A strong correlation between total phenolic content and antioxidant activity of mangoes has been established (Abbasi et al., Citation2015; Manthey and Perkins-Veazie, Citation2009; Palafox-Carlosa et al., Citation2012), supporting the widely accepted view that phenolic compounds contribute significantly to antioxidant capacity. Tree-ripened mangoes had a significantly higher phenolic content (42.43 ± 5.73 mgGAE/100 g) and DPPH radical scavenging activity (49.34 ± 5.94 100 mg/ml) when compared to postharvest-ripened mangoes (32.81 ± 5.64 mgGAE/100 g and 38.84 ± 6.50 100 mg/ml, respectively). The results were comparable to the average total phenolic content of 31.2 ± 7.8 mgGAE/100 g reported for Tommy Atkins, Kent, Keitt, and Haden varieties (Manthey and Perkins-Veazie, Citation2009). Ashwina hybrid mangoes also showed an increase in total phenolics as the fruit matured (Hossain et al., Citation2014). Polyphenol oxidase is associated with enzymatic browning and was found to have high levels of activity in ripe mangoes (Hossain et al., Citation2014). A corresponding higher DPPH radical scavenging activity with that of phenolic content was expected (). Tree-ripened mangoes should therefore provide greater health benefits owing to higher concentrations of phytochemicals and antioxidant activities. The total phenolic content of the fruit not only impacts on its medicinal properties but also its taste. Astringency has been attributed to the presence of phenolics (He et al., Citation2015; Joslyn and Goldstein, Citation1964). Tannins interact with salivary proteins and are believed to have a significant impact on the astringency of fruits (He et al., Citation2015). The higher phenolic content of tree-ripened mangoes suggests higher levels of astringency than that of postharvest-ripened mangoes.
Table 3. Comparison of the phenolic content and DPPH radical scavenging activities of tree-ripened and postharvest-ripened mangoes.
Conclusion
The results of this study indicate that there was a significant difference in the biochemical composition of tree-ripened and postharvest-ripened East Indian mangoes. Variations were observed in the reducing sugar content, titratable acidity, pH, and phenolic content of tree-ripened versus postharvest-ripened mangoes. As the mangoes matured, the level of titratable acidity decreased. This may be due to metabolic processes such as respiration that results in the metabolism of fruit acids such as citric acid and malic acid. The high TSS content of the fruits suggests that they are sweet in taste. The difference in perceived taste of tree-ripened versus postharvest-ripened mangoes may be due to differences in the actual carbohydrate composition of the fruit. Postharvest-ripened fruits had higher levels of reducing sugars than tree-ripened mangoes. Higher levels of total phenolics and radical scavenging activity were detected in tree-ripened mangoes. The undertaking of this study has revealed that additional research is required to understand and compare the biochemical compounds and pathways that are associated with ripening on the parent plant and ripening during storage. These variations in the chemical composition of tree-ripened and postharvest-ripened mangoes will have an impact on the perceived taste of the fruits.
References
- Abbasi, A.M., X. Guo, X. Fu, L. Zhou, Y. Chen, Y. Zhu, H. Yan, and R.H. Liu. 2015. Comparative assessment of phenolic content and in vitro antioxidant capacity in the pulp and peel of mango cultivars. Int. J. Mol. Sci. 16:13507–13527. doi: 10.3390/ijms160613507.
- Afifa, K., M. Kamruzzaman, I. Mahfuza, H. Afzal, H. Arzina, and H. Roksana. 2014. A comparison with antioxidant and functional properties among five mango (Mangifera indica L.) varieties in Bangladesh. Int. Food Res. J. 21:1501–1506.
- Berardini, N., R. Carle, and A. Schieber. 2004. Characterization of gallotannins and benzophenone derivatives from mango (Mangifera indica L. cv. “Tommy Atkins”) peels, pulp and kernels by high-performance liquid/chromatography/electrospray ionization mass spectrometry. Rapid Commun. Mass Spectrom. 18:2208–2214. doi: 10.1002/rcm.1611.
- Berardini, N., R. Fezer, J. Conrad, U. Beifuss, R. Carle, and A. Schieber. 2005. Screening of mango (Mangifera indica) cultivars for their contents of flavonol o- and xanthone c-glycosides, anthocyanins, and pectin. J. Agric. Food Chem. 53:1563–1570. doi: 10.1021/jf0484069.
- Brand-Williams, W., M. Cuvelier, and C. Berset. 1995. Use of a free radical method to evaluate antioxidant activity. LWT – Food Sci. Technol. 28:25–30. doi: 10.1016/S0023-6438(95)80008-5.
- Brecht, J.K., M.A. Ritenour, N.F. Haard, and G.W. Chism.2008. Postharvest physiology of edible plants, p. 975–1049. In: S. Damodaran, K.L. Parkin, and O.R. Fennema (eds.). Fennema’s food chemistry. Vol. 2, CRS Press, Florida.
- Corrales-Bernal, A., L. Amparo Urango, B. Rojano, and M.E. Maldonado. 2014. In vitro and in vivo effects of mango pulp (Mangifera indica cv. Azucar) in colon carcinogenesis. Arch. Latinoam. Nutr. 64:16–23.
- Deshpande, A.B., K. Anamika, V. Jha, H.G. Chidley, P.S. Oak, N.Y. Kadoo, K.H. Pujari, A.P. Giri, and V.S. Gupta. 2017. Transcriptional transitions in Alphonso mango (Mangifera indica L.) during fruit development and ripening explain its distinct aroma and shelf life characteristics. Sci. Rep. 7:8711. Article number. doi: 10.1038/s41598-017-08499-5.
- Elahi, M., and N. Khan. 1973. Physicochemical changes in some Pakistani mango varieties during storage ripening. J. Agric. Food Chem. 21:229–231. doi: 10.1021/jf60186a024.
- Gill, P., S.K. Jawandha, N. Kaur, and N. Singh. 2017. Physico-chemical changes during progressive ripening of mango (Mangifera indica L.) cv. Dashehari under different temperature regimes. J. Food Sci. Technol. 54(7):1964–1970. doi: 10.1007/s13197-017-2632-6.
- Gondi, M., and U.J.S. Prasada Rao. 2015. Ethanol extract of mango (Mangifera indica L.) peel inhibits α-amylase and α-glucosidase activities, and ameliorates diabetes related biochemical parameters in streptozotocin (STZ)-induced diabetic rats. J. Food Sci. Technol. 52:7883–7889. doi: 10.1007/s13197-015-1963-4.
- He, M., H. Tian, X. Luo, X. Qi, and X. Chen. 2015. Molecular progress in research on fruit astringency. Mol. 20:1434–1451. doi: 10.3390/molecules20011434.
- Hossain, M.A., M.M. Rana, Y. Kimura, and H.A. Roslan. 2014. Changes in biochemical characteristics and activities of ripening associated enzymes in mango fruit during the storage at different temperatures. BioMed. Res. Int 11. Article ID 232969 2014. doi: 10.1155/2014/232969.
- Jha, S.N., A.R.P. Kingsly, and S. Chopra. 2006. Physical and mechanical properties of mango during growth and storage for determination of maturity. J. Food Eng. 72(1):73–76. doi: 10.1016/j.jfoodeng.2004.11.020.
- Jha, S.N., K. Narsaiah, A.D. Sharma, M. Singh, S. Bansal, and R. Kumar. 2010. Quality parameters of mango and potential of non-destructive techniques for their measurement – A review. J. Food Sci. Technol.. 47:1–14. doi: 10.1007/s13197-010-0004-6.
- Joslyn, M.A., and J.L. Goldstein. 1964. Astringency of fruits and fruit products in relation to phenolic content. Adv. Food Nutr. Res. 13:179–217. doi: 10.1016/S0065-2628(08)60101-9.
- Kansci, G., B.B. Koubala, and I.L. Mbome. 2008. Biochemical and physicochemical properties of four mango varieties and some quality characteristics of their jams. J. Food Process. Preserv. 32:644–655. doi: 10.1111/j.1745-4549.2008.00204.x.
- Kim, H., N. Banerjee, I. Ivanov, C.M. Pfent, K.R. Prudhomme, W.H. Bisson, R.H. Dashwood, S.T. Talcott, and S.U. Mertens-Talcott. 2016. Comparison of anti-inflammatory mechanisms of mango (Mangifera indica L.) and pomegranate (Punica granatum L.) in a preclinical model of colitis. Mol. Nutr. Food Res. 60:1912–1923. doi: 10.1002/mnfr.201501008.
- Kiple, K.F., and K.C. Ornelas. 2000. Mango. Volume 2, p. 1809. In: (ed.). Cambridge world history of food. Cambridge University Press, Cambridge, UK
- Lane, J.H., and L. Eynon, 1923. Determination of reducing sugars by means of Fehling's solution with methylene blue as internal indicator. J. Soc. Chem. Ind. Trans. 42: 32–36
- Lauricella, M., S. Emanuele, G. Calvaruso, M. Giuliano, and A. D’Anneo. 2017. Multifaceted health benefits of Mangifera indica l. (mango): The inestimable value of orchards recently planted in Sicilian rural areas. Nutrients 9(5):525. doi: 10.3390/nu9050525.
- Manthey, J.A., and P. Perkins-Veazie. 2009. Influences of harvest date and location on the levels of β-carotene, ascorbic acid, total phenols, the in vitro antioxidant capacity, and phenolic profiles of five commercial varieties of mango (Mangifera indica l.). J. Agric. Food Chem. 57:10825–10830. doi: 10.1021/jf902606h.
- Masibo, M., and Q. He. 2008. Major mango polyphenols and their potential significance to human health. Compr. Rev. Food. Sci. Food Saf. 7:309–319. doi: 10.1111/j.1541-4337.2008.00047.x.
- Medlicott, A.P., and A. Thompson. 1985. Analysis of sugars and organic acids in ripening mango fruits (Mangifera indica l var keitt) by high performance liquid chromatography. J. Sci. Food Agric. 36:561–566. doi: 10.1002/jsfa.2740360707.
- Morales, M., S. Zapata, T.R. Jaimes, S. Rosales, A.F. Alzate, M.E. Maldonado, P. Zamorano, and B.A. Rojano. 2017. Mangiferin content, carotenoids, tannins and oxygen radical absorbance capacity (ORAC) values of six mango (Mangifera indica) cultivars from the Colombian Caribbean. J. Med. Plants Res. 11(7):144–152. doi: 10.5897/JMPR2017.6335.
- Morton, J. 1987. Mango, p. 221–239. In: Curtis F. Dowling, editor, Fruits of warm climates, Miami, Fla. Original from The University of Michigan, USA: Julia F. Morton.
- Mukherjee, S.K., and R.E. Litz. 2009. Introduction: Botany and importance, p.1–18. In: E. Litz (ed.). The mango: Botany, production and uses. Vol. 2, CAB International, UK. doi: 10.1079/9781845934897.0001.
- Palafox-Carlosa, H., E. Yahiab, M.A. Islas-Osunaa, P. Gutierrez-Martinezc, M. Robles-Sánchezd, and G.A. González-Aguilara. 2012. Effect of ripeness stage of mango fruit (Mangifera indica L., cv. Ataulfo) on physiological parameters and antioxidant activity. Sci. Hort. 135:7–13. doi: 10.1016/j.scienta.2011.11.027.
- Robichaud, J.L., and A.C. Noble. 1990. Astringency and bitterness of selected phenolics in wine. J. Sci. Food Agric. 53(3):343–353. doi: 10.1002/(ISSN)1097-0010.
- Silva, A., J. Nascimento, F. Lajolo, and B. Cordenunsi. 2008. Starch mobilization and sucrose accumulation in the pulp of Keitt mangoes during postharvest ripening. J. Food Biochem. 32:384–395. doi: 10.1111/j.1745-4514.2008.00175.x.
- Singleton, V.L., R. Orthofer, and R.M. Lamuela-Raventós. 1999. Analysis of total phenols and other oxidation substrates and antioxidants by means of Folin-Ciocalteu reagent. Meth. Enzymol. 299:152–178. doi: 10.1016/S0076-6879(99)99017-1.
- Wongmetha, O., L.-S. Ke, and Y.-S. Liang. 2015. The changes in physical, bio-chemical, physiological characteristics and enzyme activities of mango cv Jinhwang during fruit growth and development. Njas-Wagen J. Life Sc. 72–73:7–12. doi: 10.1016/j.njas.2014.10.001.
- Yashoda, H.M., T.N. Prabha, and R.N. Tharanathan. 2006. Mango ripening: Changes in cell wall constituents in relation to textural softening. J. Sci. Food Agric. 86:713–721. doi: 10.1002/jsfa.2404.
- Yeshitela, T., P.J. Robbertse, and P.J.C. Stassen. 2004. Effects of various inductive periods and chemicals on flowering and vegetative growth of ‘Tommy Atkins’ and ‘Keitt’ mango (Mangifera indica) cultivars. N. Z. J. Crop. Hortic. Sci. 32(2):209–215. doi: 10.1080/01140671.2004.9514298.
- Zakaria, A., A.Y. Md Shakaff, M.J. Masnan, F.S.A. Saad, A.H. Adom, M.N. Ahmad, M.N. Jaafar, A.H. Abdullah, and L.M. Kamarudin. 2012. Improved maturity and ripeness classifications of Magnifera indica cv Harumanis mangoes through sensor fusion of an electronic nose and acoustic sensor. Sensors. 12:6023–6048. doi: 10.3390/s120506023.