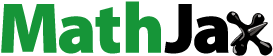
ABSTRACT
This study investigated the rehydration, water activity, pH, Brix, color, bulk density, tapped density, flowability, cohesiveness, wettability, solubility values, and microstructures of freeze-dried European cranberrybush powders. Additionally, experimental freeze-drying data were coupled to nine thin-layer models, and the logarithmic model was chosen to estimate the moisture ratio of European cranberrybush. The pH and Brix values were measured between 3.0–3.2 and 40.33–52.00 for different powder sizes, respectively. A significant difference was observed for the water activity values of different powders that were classified into different powder sizes (P < .05). Moreover, it was identified that all powders exhibited “low” cohesiveness and “fair” flowability. However, the results showed that the highest bulk density (0.440 g/ml), tapped density (0.563 g/ml), wettability (4386 s) and solubility (264 s) were obtained in the samples with a “>500 µm” powder size. According to the all scanning electron microscopy images, skeletal-like structures formed on the surfaces.
Introduction
European cranberrybush (Viburnum opulus L.) is an organic fruit that has increased its demand rapidly in recent decades (Yaldız et al., Citation2019). However, postharvest issues and astringent-bitter-sour tastes with typical smells are obstacles to its further consumption. Due to preservation methodologies, the fruits are used in many new and different food formulations such as granola bar, bread, cookie, pudding and cake (Turkpatent, Citation2020). The most commonly used method, sun drying, is a natural, old and low-cost method. Although it uses the sun as the heat source and therefore produces no CO2, there is no possibility of drying by using solar heat everywhere and at any time. In addition, the disadvantages of this process are inadequate drying, spoiling due to wind, rain, dust and moisture, the encroachment of insects, rodents and birds and fungal growth (Purohit et al., Citation2006; Sharma et al., Citation2009). Therefore, artificial drying systems are preferred for several reasons (Erbay and Küçüköner, Citation2008)
When comparing drying methods, freeze-drying, also called lyophilization, is the best preservation method with the application of primary drying/ice sublimation and drying/desorption of unfrozen water (Ma et al., Citation2020). Additionally, sublimation processing under vacuum at low temperatures minimizes enzymatic and nonenzymatic browning reactions, biological activities, texture, aroma and nutritional values of foods (Dirim et al., Citation2015). Thus, the obtained product has a porous internal structure, and the initial shape of the product does not deteriorate because the shrinkage is negligible. Therefore, freeze-drying has been known as the best drying method to keep the original properties of the raw materials (Domin et al., Citation2020). With the increase in consumer awareness about quality products, the freeze-drying method is more widely used in food processing (Dirim et al., Citation2015).
On the other hand, drying processes may provoke changes in fruit powder quality (Michalska et al., Citation2016). Mixtures of hot and cold drinks, salad dressings, soup, cakes, cookies and fruit juices that use powdered products are affected as a consequence. In recent years, the application of powder production has gained a considerable deal of attention, and various drying studies have reported the successful use of freeze-drying for fruits. Si et al. (Citation2016) examined the physical properties, antioxidant activity and bioactive compounds of raspberry powders obtained by various drying techniques (freeze-drying, infrared radiation, infrared radiation-microwave vacuum-drying, hot air-drying and hot air-explosion puffing). They determined that freeze-dried raspberry powders had higher values of soluble solids (63.46%), water solubility (45.26%), hygroscopicity (18.06%), anthocyanin retention and color parameters (60.70%) than those of raspberry powders produced with the other drying methods. Similarly, Forero et al. (Citation2016) explored lulo fruit powder that was freeze-dried or hot air-, spray- and ultrasound-assisted hot air-dried. The highest insoluble dietary fiber percentage (87.9%) was measured in the freeze-dried powders. In brief, the quality standards such as a particular moisture content, morphology, particle size and particle size distribution should meet by end powder product (Ratti, Citation2013).
The scope of the present research was to assess the drying kinetics of European cranberrybush with freeze-drying using various thin-layer drying models. This research also intended to produce freeze-dried European cranberrybush in different powder sizes as a functional ingredient in food products evaluating physicochemical and morphological properties.
Materials and Methods
Drying Procedure and Drying Equipment
For the experiments, European cranberrybush samples were collected from the fields of Corekdere Village, Kayseri, Turkey. Fruits were kept in plastic drums under refrigeration conditions (4 ± 0.5°C). The initial moisture content on a dry basis was measured as 5.32 ± 0.04 (g water g dry matter−1) with oven drying (Elektromag, M3025P, Turkey) at 105°C for 24 h (Taşkın et al., Citation2018). To grind samples into a puree, a home-type blender (800, Tefal, France) was used. The freeze-drying process was performed in a lab-type freeze-dryer (Alpha 1–2 LD Plus, Osterode am Harz, Germany) at a constant pressure of 52 Pa and a − 50°C processing temperature in the drying chamber. To evaluate any moisture loss during the drying process, the sample was weighed hourly with a digital balance with an accuracy of ±0.01 g (WH −5002, Weightlab Ins., Turkey). A quantity of 250 g of freeze-dried European cranberrybush puree was milled (Hc-500, Lavion, PRC) at constant speed before sieving. Powder sizes of “>500, 425–500, 200–425, 100–200 and 63–100 µm” were obtained with stacking and shaking of laboratory sieve shakers (As200, Retsch, Germany) (Caparino et al., Citation2012; Ermiş et al., Citation2018). All experiments were conducted in triplicate.
Mathematical Modeling
The moisture content data collected from the freeze-drying experiment were utilized to calculate the moisture ratio (MR) and then implemented in thin-layer models of “Henderson and Pabis,” “Newton,” “Page,” “Logarithmic,” “Two Term,” “Two Term Exponential,” “Wang and Singh,” “Diffusion Approach” and “Midilli et al.” to determine the most appropriate model (Taskin, Citation2020). The MR of the samples was determined according to EquationEq. (1(1)
(1) ), and the moisture ratio of the equation was simplified as EquationEq. (2)
(2)
(2) :
where Mt is the moisture content (g water g dry matter−1) at a particular time, Me is the equilibrium moisture content, and Mo is the initial moisture content (Xiao et al., Citation2010).
Rehydration, Water Activity, pH and Brix Analysis
The rehydration degree determination was prepared using one gram of powder added to 20 mL of distilled water that was kept at 25°C for 1 h. The mixture was centrifuged (Universal 320, Hettich, Germany) at 2,000 × g for 15 min. The precipitate weight was utilized as the rehydrated powder weight. The rehydration degree (Rd) was computed with EquationEq. (3(3)
(3) ) (Kim et al., Citation2012):
The water activity, pH and Brix values were measured with a water activity meter (Labtouch-AW, Novasina, Switzerland), pH meter (Starter 300, Ohaus, USA) and digital refractometer (RA600, KEM, Japan), respectively (Dirim and Çaliskan, Citation2012). For pH and Brix measurements, two g of dried European cranberrybush samples were homogenized (WiseTis/HG-15D, Daihan, Korea) in 10 mL distilled water for 3 min, and then the homogenates were filtered under vacuum. After this step, obtained juice samples were used for pH and Brix analysis.
Color Measurement
Color analysis was performed with a color analyzer (MSEZ-4500 L, Hunterlab, USA). The coordinates of the lightness (L*), redness (a*) and yellowness (b*) were obtained after instrument calibration. Chroma (C), hue angle (α) and total color difference (ΔE) values were estimated from the color coordinates by applying EquationEqs. (4)(4)
(4) , (Equation5
(5)
(5) ) and (Equation6
(6)
(6) ), respectively. Here, the subscript “o” denotes the color of the fresh sample (Taşkın et al., Citation2018).
Flow Property Determination
The bulk density (ρbulk) was determined by gently placing the powders into a 50 ml graduated cylinder. The ratio of mass to volume read from the cylinder was expressed as the bulk density. The same cylinder was tapped 120 times to measure the tapped density (ρtapped). The Carr index (CI) and Hausner ratio (HR) were used to consider the flowability and cohesiveness values of the powders, respectively. Both CI and HR were calculated from the tapped and bulk densities of the powder using EquationEqs. (7)(7)
(7) and (Equation8
(8)
(8) ) (Caliskan and Dirim, Citation2016):
The wettability was obtained by placing 10 g powder on 100 mL distilled water at 25°C in a 250 mL beaker. The time was saved until the powder placed around the beaker completely wetted. On the other hand, the solubility was determined by adding 2 g of the powder to 50 mL of distilled water at 30°C in a glass beaker using a magnetic stirrer. The time to dissolve the whole powder was recorded (Gong et al., Citation2007; Goula and Adamopoulos, Citation2008).
Scanning Electron Microscopy Imaging
The tissue characteristics of the freeze-dried powders were evaluated with scanning electron microscopy (SEM) (EVO 40, Carl Zeiss, Oberkochen, Germany). The powder surface was covered with a thin layer of gold-palladium under high vacuum conditions (20 kV) (SCD-005, Baltec, Wetzlar, Germany). The SEM images were taken at a magnification of 1000× (Lee et al., Citation2012).
Statistical Analysis
The software package MATLAB (MathWorks Inc., Natick, MA) was used in the modeling studies. In addition, the statistical parameters were explored by MINITAB (Version 14, Texas University, Austin, TX, USA), the significance of mean differences was tested, and the LSD (least significant difference) test was implemented with a 5% significance level. The R2 (coefficient of determination), RMSE (root-mean-square error) and (reduced chi-squared) were used to verify the best equation for fitting variation in the drying curve of the dried sample. The statistical values were calculated with EquationEqs. (9)
(9)
(9) and (Equation10
(10)
(10) ):
where MRexp,i and MRpre,i are the experimental and predicted moisture ratios, respectively, N is the number of experimental data points, and n is the number of parameters in the model (Özbek and Dadalı, Citation2007).
Results and Discussion
Drying Kinetics and Modeling of the Drying Curve
Variations in the moisture ratio versus the drying time of European cranberrybush powders and the change in the experimental and predicted moisture ratio with the most appropriate models in terms of drying time under freeze-drying conditions are presented in . The total time to reach the final moisture content was 720 min in the freeze-drying process. This was in line with the results obtained by Izli et al. (Citation2017) that freeze-drying is a time-consuming process compared to other drying techniques. On the other hand, experimental data were coupled to the models of “Henderson and Pabis,” “Newton,” “Page,” “Logarithmic,” “Two Term,” “Two Term Exponential,” “Wang and Singh,” “Diffusion Approach” and “Midilli et al..” The statistical analysis for the evaluation of the fitting quality (R2, RMSE and χ2) and the model constants values of the nine models, the values of R2, RMSE and χ2 ranged from 0.7999 to 0.9989, 0.0097 to 0.1298 and 0.8137 × 10−4 to 170.396 × 10−4, respectively. However, the freeze-drying curves of European cranberrybush powder were best-described by a logarithmic model, which exhibited the lowest RMSE (0.0097) and χ2 (0.8137 × 10−4) and highest R2 (0.9989) values. As seen from , plots of experimental values and predicted values applying the most suitable model proved satisfactory for describing the freeze-drying characteristics of European cranberrybush powders. Similarly, Çalışkan and Dirim (Citation2015) chose the logarithmic model for freeze-drying of persimmon puree, which was fitted with great accuracy compared to that of ten well-known empirical thin-layer drying models. Additionally, button mushroom slices were freeze-dried, and the best fit was also based on the statistical tests provided by a logarithmic model (Pei et al., Citation2014).
Rehydration, Water Activity, pH and Brix Properties
shows the rehydration, water activity, pH and Brix values of fresh and freeze-dried European cranberrybush powders of different sizes. One of the major quality characteristics of dried products is their ability to be rapidly and completely rehydrated. The results indicated that the rehydration degree increased with increasing powder size. The values increased from 136.563 ± 0.499 (the lowest) for the powder size of “63–100 µm” to 200.288 ± 0.968 (the highest) for the powder size of “>500 µm.” Due to particle size of the sample, the higher water uptake was likely because of the filling of free capillaries and intercellular spaces with water. This result was in line with that of Kim et al. (Citation2012), who determined a higher rehydration degree for larger-sized powders of freeze-dried jujube fruit. From the water activity values, the fresh sample (0.944 ± 0.004) contained a significantly higher water activity than that of any sized powder and thus limited shelf life. This might due to absorb more free water (Oberoi and Sogi, Citation2015) and the values of water activity from 0.20 to 0.40 ensure the stability of the product against browning, and hydrolitical reactions, lipid oxidation, auto-oxidation, and enzymatic activity (Marques et al., Citation2007). In the study of Dirim and Çaliskan (Citation2012) on the production of pumpkin powder by freeze-drying, water activity values in similar range as about 0.2 after 9 hours of freeze-drying process. As a result of the pH values in our study, all powder sizes were higher compared to the value of the fresh sample (2.9 ± 0.1). In addition, acidity increased with decreasing powder size, probably because there were not permit of a significant amount of this component in the particle surface (Antigo et al., Citation2018). On the other hand, Brix values reached a final value between 40.33 ± 1.04 and 52.00 ± 0.50. The difference of Brix values between powders could be associated with a large contact surface and the cell-wall materials (Nabil et al., Citation2020). Also, previous research on European cranberrybush showed similar fresh sample values of 2.8 (pH) and 10.35 (Brix) (Yilmaztekin and Sislioglu, Citation2015) and the small differences may be attributed to the growth conditions, harvesting times, soil properties, and geographical variations.
Table 1. Physicochemical properties of fresh and freeze-dried European cranberrybush powders
Color Evaluation
The L*, a*, b*, C, α and ΔE (color parameters) of fresh and freeze-dried European cranberrybush powder of different sizes are presented in . The L*, a* and b* values of European cranberrybush were 42.38 ± 0.16, 44.92 ± 0.32 and 23.15 ± 0.18, respectively. In all the treatments, the L* and b* values increased for all powder sizes, whereas the a* value did not significantly changed between the fresh and “>500 µm” samples (P < .05). The freeze drying has a marked effect in the brightness of the dried samples: it originated by sublimation of small ice crystals formed by freezing drying, scatter more light than fresh ones (Ceballos et al., Citation2012). The highest C and α variations were calculated for the samples with sizes of “425–500 µm” and “>500 µm,” while the lowest occurred in the samples with the size of “63–100 µm” and the “fresh” sample, respectively. The ΔE values showed a significant difference with increasing powder size from “63–100 µm” to “>500 µm” (P < .05). Based on the results of this study, the color changes rate trend of samples provides an end-product of highly acceptable color. Additionally, the particle size within range of “>500, 425–500, 200–425, 100–200 and 63–100 µm” affected the color parameters of European cranberrybush powder to a relatively small extent as expected (Chen et al., Citation1999). These results were in agreement with earlier values given in the literature. Caparino et al. (Citation2012) investigated the impact of freeze-drying and drum, spray and refractance window drying techniques on the color of mango powders of different particle sizes. Comparing the “>500 µm” and “350–500 µm” in terms of the L*, b*, C and α parameters, the highest values were found in the freeze-dried samples.
Table 2. Color variations of fresh and freeze-dried European cranberrybush powders
Flow Characterization
exhibits the flow properties of bulk density, tapped density, flowability, cohesiveness, wettability and solubility of freeze-dried European cranberrybush powders of different sizes. The highest bulk density, tapped density, wettability and solubility were observed for the “>500 µm” samples, while those of the “63–100 µm” samples were the lowest (P < .05). The flowability and cohesiveness ranged between 22.019 ± 1.322 and 31.370 ± 2.318 and 0.686 ± 0.023 and 0.780 ± 0.013, respectively. According to the classification of flowability and cohesiveness presented by Jinapong et al. (Citation2008), all European cranberrybush powder sizes were found to exhibit “low” cohesiveness and “fair” flowability. Flow characterization changes in powder products have been reported by researchers as follows: Michalska et al. (Citation2016) assessed the effects of different drying technologies on plum powder properties. The lowest true and bulk densities were determined in freeze-dried powders, whereas porosity and solubility were highest. In parallel, Çalıskan et al. (Citation2015) examined freeze-dried kiwi powder and reported bulk density, tapped density, solubility, wettability, flowability and cohesiveness values of 16 ± 0.01 g ml−1, 0.26 ± 0.01 g ml−1, 26 ± 3 s, 78.5 ± 2 s, 38 ± 3 and 1.6 ± 0.08, respectively.
Table 3. Flow properties of freeze-dried European cranberrybush powders
Microstructure
The scanning electron microscopy images taken from the freeze-dried European cranberrybush powders of “>500 µm,” “425–500 µm,” “200–425 µm,” “100–200 µm” and “63–100 µm” sizes are presented in , respectively. The microstructures were investigated by 1000× magnification. Although the tissues were quite similar for every freeze-dried powder size, they showed large porosities and skeletal-like structures. These considerations were in accordance with the microstructure of freeze-dried mango powders, as explained by Caparino et al. (Citation2012). In addition, Lee et al. (Citation2012) found that the porosities of hot air-dried Citrus ‘Hallabong’ powders were relatively small due to shrinkage and surface hardening, while freeze-dried Citrus ‘Hallabong’ powders were relatively large. Consequently, microstructural properties of the foods were clearly observed in good correlation with food texture quality which has significant effects on the gas and heat transport in the food matrix and its nutritional, chemical, and microbiological stability (Do Trong et al., Citation2014).
Conclusion
In this study, European cranberrybush was freeze-dried and separated with different sieve sizes. The powders were compared in terms of physicochemical, flow and morphological properties. According to statistical parameters, the results of the moisture ratio values were best fit with a logarithmic model. By increasing the powder sizes, the rehydration and total color difference values increased. However, there was no significant difference between their scanning electron microscopy images. Moreover, the lowest bulk density (0.230 ± 0.001 g/ml), tapped density (0.295 ± 0.005 g/ml), flowability (22.019 ± 1.322%), wettability (534 ± 5 s) and solubility (170 ± 2 s) were detected in the “63–100 µm” powder samples. Therefore, the developed freeze-dried European cranberrybush powder can easily be used in many new and different food formulations or can be precursor products in different industries with preserved quality parameters.
Recommendations
European Cranberrybush (Viburnum opulus L.) uses as folk medicine by European, Asian and Native American people for the preventive effect on many diseases. However, there is a very limited study to develop new products from the fruit. Freeze-dried European Cranberrybush powder is an alternative to consume after the harvest season with preserved quality parameters. In addition, various food sectors can be used as sub-products with the results of the determination of physicochemical and morphological properties.
Acknowledgments
This research was financed by TUBITAK, National Support Programmes (Project No. 118O504).
Disclosure Statement
The authors have declared no conflict of interest for this article.
Additional information
Funding
References
- Antigo, J.L.D., R.D.C. Bergamasco, and G.S. Madrona. 2018. Effect of pH on the stability of red beet extract (Beta vulgaris L.) microcapsules produced by spray drying or freeze drying. Food Sci. Technol (Campinas). 38(1):72–77. doi: https://doi.org/10.1590/1678-457x.34316.
- Caliskan, G., and S.N. Dirim. 2016. The effect of different drying processes and the amounts of maltodextrin addition on the powder properties of sumac extract powders. Powder Technology. 287:308–314. doi: https://doi.org/10.1016/j.powtec.2015.10.019.
- Çalışkan, G., and S.N. Dirim. 2015. Freeze drying kinetics of persimmon puree. Gıda 40(1):9–14.
- Çalıskan, G., K. Ergün, and S.N. Dirim. 2015. Freeze drying of kiwi (Actinidia deliciosa) puree and the powder properties. Ital. J. Food Sci 27(3):385–396.
- Caparino, O.A., J. Tang, C.I. Nindo, S.S. Sablani, J.R. Powers, and J.K. Fellman. 2012. Effect of drying methods on the physical properties and microstructures of mango (Philippine ‘Carabao’ var.) powder. J. Food Eng. 111(1):135–148. doi: https://doi.org/10.1016/j.jfoodeng.2012.01.010.
- Ceballos, A.M., G.I. Giraldo, and C.E. Orrego. 2012. Effect of freezing rate on quality parameters of freeze dried soursop fruit pulp. J. Food Eng. 111(2):360–365. doi: https://doi.org/10.1016/j.jfoodeng.2012.02.010.
- Chen, Q., H.K. Koh, and J.B. Park. 1999. Color evaluation of red pepper powder. Transactions of the ASAE 42(3):749–752. doi: https://doi.org/10.13031/2013.13237.
- Dirim, S.N., and G. Çaliskan. 2012. Determination of the effect of freeze drying process on the production of pumpkin (Cucurbita Moschata) puree powder and the powder properties. Gıda 37(4):203–210.
- Dirim, S.N., G. Çalışkan, and K. Ergün. 2015. Dondurularak kurutulmuş bazı meyve tozlarının toz ürün özelliklerinin belirlenmesi. Gıda 40(2):85–92.
- Do Trong, N.N., A. Rizzolo, E. Herremans, M. Vanoli, G. Cortellino, C. Erkinbaev, M. Tsuta, L. Spinelli, D. Contibi, A. Torricelli, et al. 2014. Reprint of” optical properties–microstructure–texture relationships of dried apple slices: Spatially resolved diffuse reflectance spectroscopy as a novel technique for analysis and process control”. Innov. Food Sci. Emerg. Technol. 24:145–153. doi: https://doi.org/10.1016/j.ifset.2014.07.007.
- Domin, M., D. Dziki, S. Kłapsia, A. Blicharz-Kania, B. Biernacka, and A. Krzykowski. 2020. Influence of the freeze-drying conditions on the physicochemical properties and grinding characteristics of kiwi. Int. J. Food Eng. 16(1–2):1–8. doi: https://doi.org/10.1515/ijfe-2018-0315.
- Erbay, B., and E. Küçüköner. 2008. Gıda endüstrisinde kullanılan farklı kurutma sistemleri. Türkiye 10. Gıda Kongresi.
- Ermiş, E., R. Güneş, and İ. Zent. 2018. Bazı model Toz Gıdaların Akışkanlığına ve Sıkıştırılabilirliğine Partikül Boyutunun Etkisinin PFT Toz Akışı Test Cihazı Kullanılarak Belirlenmesi. Turkish JAF Sci. Tech. 6(1):55–60. doi: https://doi.org/10.24925/turjaf.v6i1.55-60.1622.
- Forero, D.P., J.G. Carriazo, and C. Osorio. 2016. Effect of different drying methods on morphological, thermal, and biofunctional properties of lulo (Solanum quitoense Lam.) fruit powders. Drying Technol. 34(9):1085–1094. doi: https://doi.org/10.1080/07373937.2015.1094667.
- Gong, Z., M. Zhang, A.S. Mujumdar, and J. Sun. 2007. Spray drying and agglomeration of instant bayberry powder. Drying Technol. 26(1):116–121. doi: https://doi.org/10.1080/07373930701781751.
- Goula, A.M., and K.G. Adamopoulos. 2008. Effect of maltodextrin addition during spray drying of tomato pulp in dehumidified air: II. Powder properties. Drying Technol. 26(6):726–737. doi: https://doi.org/10.1080/07373930802046377.
- Izli, N., G. Izli, and O. Taskin. 2017. Drying kinetics, colour, total phenolic content and antioxidant capacity properties of kiwi dried by different methods. J. Food Meas. Charact. 11(1):64–74. doi: https://doi.org/10.1007/s11694-016-9372-6.
- Jinapong, N., M. Suphantharika, and P. Jamnong. 2008. Production of instant soymilk powders by ultrafiltration, spray drying and fluidized bed agglomeration. J. Food Eng. 84(2):194–205. doi: https://doi.org/10.1016/j.jfoodeng.2007.04.032.
- Kim, S.H., Y.J. Choi, H. Lee, S.-H. Lee, J.-B. Ahn, B.-S. Noh, and S.C. Min. 2012. Physicochemical properties of jujube powder from air, vacuum, and freeze drying and their correlations. J. Korean Soc. Appl. Bi. 55(2):271–279. doi: https://doi.org/10.1007/s13765-012-1039-3.
- Lee, C.W., H.J. Oh, S.H. Han, and S.B. Lim. 2012. Effects of hot air and freeze drying methods on physicochemical properties of citrus ‘hallabong’powders”. Food Sci. Biotechnol. 21(6):1633–1639. doi: https://doi.org/10.1007/s10068-012-0217-8.
- Ma, Y., J. Gao, W. Jia, Y. Liu, L. Zhang, Q. Yang, J. Guo, J. Zhao, B. Yan, and Y. Wang. 2020. A comparison of spray-drying and freeze-drying for the production of stable silybin nanosuspensions. J. Nanosci. Nanotechnol. 20(6):3598–3603. doi: https://doi.org/10.1166/jnn.2020.17407.
- Marques, L.G., M.C. Ferreira, and J.T. Freire. 2007. Freeze-drying of acerola (Malpighia glabra L.). Chem. Eng. Process. 46(5):451–457. doi: https://doi.org/10.1016/j.cep.2006.04.011.
- Michalska, A., A. Wojdyło, K. Lech, G.P. Łysiak, and A. Figiel. 2016. Physicochemical properties of whole fruit plum powders obtained using different drying technologies. Food Chem. 207:223–232. doi: https://doi.org/10.1016/j.foodchem.2016.03.075.
- Nabil, B., R. Ouaabou, M. Ouhammou, L. Saadouni, and M. Mahrouz. 2020. Impact of particle size on functional, physicochemical properties and antioxidant activity of cladode powder (Opuntia ficus-indica). J. Food Sci. Technol. 57(3):943–954. doi: https://doi.org/10.1007/s13197-019-04127-4.
- Oberoi, D.P.S., and D.S. Sogi. 2015. Effect of drying methods and maltodextrin concentration on pigment content of watermelon juice powder. J. Food Eng. 165:172–178. doi: https://doi.org/10.1016/j.jfoodeng.2015.06.024.
- Özbek, B., and G. Dadalı. 2007. Thin-layer drying characteristics and modelling of mint leaves undergoing microwave treatment. J. Food Eng. 83(4):541–549. doi: https://doi.org/10.1016/j.jfoodeng.2007.04.004.
- Pei, F., Y. Shi, A.M. Mariga, W.J. Yang, X.Z. Tang, L.Y. Zhao, Q.H. Hu, and Q.-H. Hu. 2014. Comparison of freeze-drying and freeze-drying combined with microwave vacuum drying methods on drying kinetics and rehydration characteristics of button mushroom (Agaricus bisporus) slices. Food Bioprocess Technol. 7(6):1629–1639. doi: https://doi.org/10.1007/s11947-013-1199-0.
- Purohit, P., A. Kumar, and T.C. Kandpal. 2006. Solar drying vs. open sun drying: A framework for financial evaluation. Sol. Energy. 80(12):1568–1579. doi: https://doi.org/10.1016/j.solener.2005.12.009.
- Ratti, C. 2013. Freeze drying for food powder production, p. 57–84. In: B. Bhandari, N. Bansal, M. Zhang, P. Schuck (eds.). Handbook of food powders. Cambridge: Woodhead Publishing.
- Sharma, A., C.R. Chen, and N.V. Lan. 2009. Solar-energy drying systems: A review. Renewable Sustainable Energy Rev. 13(6–7):1185–1210. doi: https://doi.org/10.1016/j.rser.2008.08.015.
- Si, X., Q. Chen, J. Bi, X. Wu, J. Yi, L. Zhou, and Z. Li. 2016. Comparison of different drying methods on the physical properties, bioactive compounds and antioxidant activity of raspberry powders. J. Sci. Food Agric. 96(6):2055–2062. doi: https://doi.org/10.1002/jsfa.7317.
- Taskin, O. 2020. Evaluation of freeze drying for whole, half cut and puree black chokeberry (Aronia melanocarpa L.). Heat and Mass Transfer 56(8):2503–2513. doi: https://doi.org/10.1007/s00231-020-02867-0.
- Taşkın, O., G. İzli, and N. İzli. 2018. Convective drying kinetics and quality parameters of european cranberrybush. Tarim Bilim. Derg. 24(3):349–358. doi: https://doi.org/10.15832/ankutbd.456654.
- Turkpatent. 2020. Turkish patent and trademark office. 06 Oct. 2020. <https://portal.turkpatent.gov.tr/anonim/arastirma/patent/detayli>
- Xiao, H.-W., C.-L. Pang, L.-H. Wang, J.-W. Bai, W.-X. Yang, and Z.-J. Gao. 2010. Drying kinetics and quality of Monukka Seedless grapes dried in an air-impingement jet dryer. Biosyst. Eng. 105(2):233–240. doi: https://doi.org/10.1016/j.biosystemseng.2009.11.001.
- Yaldız, G., M. Çamlıca, and F. Özen. 2019. Organik gübrelemenin tıbbi bitkilerin verim ve kalite özelliklerine etkileri. UAZİMDER 1(3):37–48.
- Yilmaztekin, M., and K. Sislioglu. 2015. Changes in volatile compounds and some physicochemical properties of european cranberrybush (Viburnum opulus L.) during ripening through traditional fermentation. J. Food Sci 80(4):687–694. doi: https://doi.org/10.1111/1750-3841.12836.