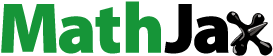
ABSTRACT
Green mold, caused by Penicillium digitatum, is a serious disease of citrus fruit during postharvest. The focus of this study was to evaluate possible biological alternatives to chemical fungicides for P. digitatum control. Four Pseudomonas isolates (Q110B, Q125B, Q112B, and Q004B) were isolated from rhizospheric soil and evaluated for their antagonistic activity against P. digitatum. The in vitro evaluations of these isolates by dual culture revealed an important zone of inhibition after 3 days of incubation at 25 ± 2°C. The Q004B isolate provided a high zone of inhibition in vitro at 45 mm diameter. Four Pseudomonas isolates tested showed antagonistic activity against P. digitatum on orange fruit after 7 days of inoculation under postharvest conditions. Inhibition percentage of P. digitatum on orange fruit ranged from 22 to 85% for Q004B and Q112B, respectively. Regarding the mechanism of action, the four tested isolates produced volatile compounds in vitro with inhibition percentage ranging from 35% to 79%, respectively for Q004B andQ125B. The Q004B isolate caused a high spore inhibition rate of 46%, while Q110B had no effect on spore germination. The four isolates of Pseudomonas produced siderophores in CAS medium. Additionally, Q125B, Q112B, and Q004B showed a positive chitinolytic activity, whereas Q110B provided negative results to the chitinase assay. These isolates represent potential candidates for biological control of citrus green mold and may be effectively used as a sustainable alternative measure to chemical fungicide control.
Introduction
Citrus is the most widely produced fruit crops on a worldwide basis (Zhong and Nicolosi, Citation2020). Fruits are traded as fresh commodities for direct consumption and are also utilized for juice extraction (Anticona et al. Citation2020). In Morocco, citrus fruit play a major role in economic development. The largest volume of citrus fruits grown and shipped from packing houses in the Souss-Massa region (Boubaker et al., Citation2009; Jaouad et al., Citation2020). However, citrus fruit in Morocco suffer significant loss from postharvest diseases and decay.
On a worldwide basis, more than 25% of harvested citrus fruits are lost during postharvest (Spadaro and Gullino, Citation2004a; Romanazzi et al., Citation2016). The losses are mainly due to postharvest decay caused by Penicillium digitatum (green mold) which is the primary postharvest pathogen of citrus (Droby et al., Citation2002); this pathogen causes significant losses worldwide (Zhu et al., Citation2006) and in Morocco (Boubaker et al., Citation2009). Infection occurs through injuries and wounds made during harvest and subsequent handling (Brown and Miller, Citation1999). Although synthetic fungicides still remain as the primary method to control postharvest diseases (Tripathi and Dubey, Citation2004), these chemical products constitute a toxicological risk, as the excessive use of some systemic fungicides has led to the development of P. digitatum resistance (Holmes and Eckert, Citation1999). Since many consumers desire commodities that are free of pesticide residues (Spadaro and Gullino, Citation2004b), there is a need for eco-friendly and effective alternatives for current disease management practices (Kanan and Al-Najar, Citation2008; Shrivastava and Kumar, Citation2018; Torres-León et al., Citation2018). Research on potential biological control methods as an alternative to chemicals has shown that antagonistic microorganisms (Leelasuphakul et al., Citation2008; Qessaoui et al., Citation2021; Taqarort et al., Citation2008) and plant extracts (Askarne et al., Citation2012; Chidi et al., Citation2020) are considered promising alternatives. Several microorganisms were reported as being effective in laboratory and pilot tests for controlling both blue and gray mold in postharvest fruit (Wallace et al., Citation2017). Previous research has indicated thatPseudomonas sp. is a suitable biological control agent for green and blue mold, caused by Penicillium sp. (Wallace et al., Citation2017; Wang et al., Citation2018). Currently, formulations of Pseudomonas for the control of diseases are becoming more commercially available (de Sousa Oliveira et al., Citation2020; Valverde et al., Citation2015). Therefore, the objective of this study was to evaluate the effect of four isolates of Pseudomonas collected from rhizospheric soils for P. digitatum control and to investigate their mode of action.
Materials and Methods
All experiments were conducted in a growth room at 25 ± 1°C, L16:D8 photoperiod, and 65 ± 5% relative humidity, at the plant protection laboratory, Integrated Crop Production Unit, National Institute of Agronomic Research (INRA), Agadir, Morocco.
Pseudomonas Isolates
The four Pseudomonas isolates evaluated (Q125B, Q004B, Q110B, Q112B) were isolated from the soil at the National Institute for Agricultural Research experimental farm near Belfaa, Agadir, in southwestern Morocco (30°02′42.2“N 9°33′13.4“W). The isolated bacteria were previously characterized, as new Pseudomonas sp.(Qessaoui et al., Citation2019) based on a partial rpoD gene sequence using the primers PsrpoD FNP1 (5′-TGAAGGCGARATCGAAATCGCCAA-3′) and PsrpoDnprpcr1 (5′-YGCMGWCAGCTTYTGCTGGCA-3′)(Qessaoui et al., Citation2019).The Pseudomonas isolates were stored in glycerol at −20°C at the Research Unit of Integrated Crop Production, National Institute for Agricultural Research, Agadir, Morocco.
Isolation of P. Digitatum
The isolate of P. digitatum was collected from infested citrus fruit. Potato Dextrose Agar (PDA, Difco) was used to select and purify P. digitatum. This isolate was identified and stored at 4°C. Periodic transfers (every six months) onto citrus fruit were used to maintain its aggressiveness.
In Vitro Screening of Antagonists for Antifungal Activity on P. Digitatum
The four selected isolates of Pseudomonas were tested using the dual culture method for their antagonistic effect on mycelial growth of P. digitatum. The Pseudomonas bacterial isolates were grown at 28◦C in nutrient broth for 24 h on a rotary shaker at 120rpm.An aliquot (10 µL) of 72 h old bacterial suspension (108cfu/mL) was placed in the center of a 9-cm diameter Petri dish containing PDA, and three plates were used for each isolate. After incubation for 24 h at 28 ± 2°C, a suspension of P. digitatum (105 conidia/mL) was sprayed over the PDA plates. The plates were incubated for an additional three days at 25 ± 2°C or until a dense growth of mycelium was present. The inhibition zone diameters were measured in mm. This procedure was repeated three times.
In Vivo Screening of Antagonists for Antifungal Activity against P. Digitatum on Orange Fruit
Preparation of Orange Fruits
‘Valencia-late’ orange fruit (Citrus sinensis L. Osbeck) were collected from unsprayed trees in a citrus orchard at the experimental farm of INRA, Belfaa, Agadir, Morocco. Only healthy, homogeneous size and commercially mature fruit (orange color) were used in this experiment. The fruits were surface-sterilized by soaking in aqueous sodium hypochlorite (2%) for 5 min (Hussien et al., Citation2018). Fruit were thoroughly rinsed and dried at laboratory ambient conditions, and were artificially wounded to a depth of 3 × 3 mm in diameter at four equidistant points around their equators using sterile needle (Askarne et al., Citation2012).
Inoculation of Fruits
Fresh cultures of P. digitatum and the Pseudomonas isolates were used for each experiment. To evaluate their antagonistic activity, the four Pseudomonas strains were grown for 48 h in King’s B medium and adjusted to 108 cfu/mL. Conidial suspensions of P. digitatum were adjusted to 105 conidia/ml. Twenty µL of a particular Pseudomonas isolate bacterial suspension was inoculated in the wells of wounded fruit. After drying for one hour under sterile conditions, the same wells were also inoculated with 20 µL of a P. digitatum conidial suspension. The fruit inoculated only with the pathogen was considered as a positive control; and, in the negative controls, fruits were only inoculated with distilled water. The fruit were then stored at 20°C for seven days in autoclaved transparent plastic bag. The P. digitatum mycelial growth inhibition percentage on treated fruits was calculated using the following formula:
Where R1 is the lesion diameter recorded in positive control fruits and R2 is the lesion diameter in treated fruits. Nine citrus fruits were used per treatment (Janisiewicz and Roitman, Citation1988).
Spore Germination Assay
Spore germination of P. digitatum was tested by mixing 40 µL of the spore suspension with an equal volume of filtrate of the supernatant of each isolate in the wells of depression slides. The slides were incubated 18 h in a sterile Petri dish containing filter paper moistened with distilled water. One hundred spores per well were examined using a microscope at x400 magnification for each Pseudomonas isolate treatment, with the spore germination rate determined. A spore was considered germinated if the germ tube length was at least as long as the conidia. As controls, 40 µL of the spore suspension were mixed with equal volume sterile distilled water. For each field, 100 conidia were counted, and the percentage of the inhibition of conidial germination was calculated as follows:
Where ICG is the inhibition of conidial germination (%), GC is the percentage of germination in the control and GD is the percentage of germination in the dual inoculation.
Effect of Volatile Compounds (Vocs) on P. Digitatum Growth
The effects of volatile organic compounds (VOCs) produced by Pseudomonas strains on the growth rates and activity of fungi were assessed using experimental systems that had only atmospheric connections (Fiddaman and Rossall, Citation1993; Rouissi et al., Citation2013). The Pseudomonas antagonism was evaluated on King’s medium B plates. After incubation for 48 h, the lid of each Petri dish was replaced by a plate containing 6 mm plug of the test fungus in PDA medium. The two plates were sealed with parafilm. Controls were also prepared without bacteria in the plate. Petri dishes were incubated at 25°C, with observations recorded after 5 days (Kumari and Khanna, Citation2014). The percentage of growth inhibition by VOCs was calculated using the formula:
Where r1 is the radial growth of the fungus in the control and r2 is the radial growth of the fungus in the treated plates.
To determine the production of Hydrogen cyanide (HCN), isolates were streaked onto KB agar plates supplemented with glycine (4.4 g l−1). Petri plates were inverted and a piece of filter paper impregnated with 0.5% picric acid and 2% sodium carbonate was placed on their lids. Petri plates were sealed with parafilm and incubated for 96 h at 28°C. HCN production was indicated by filtre color change (from orange to brown) (Bakker and Schippers Citation1987).
Siderophores Production
Production of siderophores was determined by the method of Schwyn and Neilands (Citation1987), using the CAS reagent (chrome azurol S; Fluka Chemika, Buchs, Switzerland). Isolates were grown on CAS agar plates supplemented with 2% glucose, 0.5% L-glutamic acid (neutralized), and 5 ppm biotin. The presence of orange haloes was recorded up to 7 days after incubation.
Chitinolytic Activity
For detection of chitinolytic activity on plates, cells were streaked on a mixture of SM and nutrient broth (3:1) supplemented with colloidal chitin (0.2%) and solidied with 1.5% agar (Chernin et al., Citation1995). After incubation at 30°C for 5 days, the plates were flooded with Congo red solutions (0.03%). The enzymatic activity was evidenced by appearance of clear zones around (Cattelan et al., Citation1999; Elshafie et al., Citation2012).
Statistical Analysis
The percentage of mycelial growth inhibition of P. digitatum was estimated for each Pseudomonas isolate. All data and rates were subjected to the analysis of variance (ANOVA) using Statistica software (Version 6). Data for mycelial growth inhibition percentage was presented as means ± standard deviation. Any difference mentioned was significant at p < .01 using the Newman–Keuls multiple range test.
Results
In Vitro Screening of Antagonists for Antifungal Activity against P. Digitatum
The four Pseudomonas isolates each provided inhibitory activity against P. digitatum, and was expressed as a clear halo around each colony. The inhibition zone diameter ranged from16 to 45 mm after the incubation period (). At P < 1% level, the 3 isolates Q110B, Q125B and Q004B showed the equal inhibitory activity, with 39.17, 30.00 and 45.00 mm, respectively. However, the Pseudomonas isolate Q112B caused the lowest inhibitory effect with 16.25 mm.
Table 1. Effect of four Pseudomonas isolates on P. digitatum cultures in vitro and in vivo (on Orange fruit) for 7 days.
In Vivo Screening of Fluorescent Pseudomonas Antagonists of P. Digitatum on Orange Fruit
The effects of four Pseudomonas (Q004B, Q110B, Q125B and Q112B) isolates for the control of P. digitatum on orange fruits are presented in . There were significant differences in the disease incidence or lesion diameter between the four isolates tested. The inhibition percentage ranged from 22 to 85% (). The two strains Q125B and Q112B provided the most important inhibition significantly with 56.8 and 85%, respectively, as showed by the lowest disease incidence and lesion diameter. Therefore two strains Q004B and Q110B caused the least inhibition, 22 and 30,9%, respectively.
Spore Germination Assay
Results indicate that among the four tested isolates, only Q112B, Q004B and Q125B significantly inhibited germination of P. digitatum spores 18 h after incubation in laboratory condition (). The inhibition rate of spore germination ranged from 27% to 57.80% for Q112B and Q125B respectively. However, the Pseudomonas isolate Q110B did not inhibited spore germination of P. digitatum.
Table 2. Penicillium digitatum spore germination and mycelium inhibition by four Pseudomonas isolates and their metabolites.
Production of Volatile Antifungal Compounds (Vocs)
The results of VOCs indicated that the four Pseudomonas isolates produced volatile products effective against P. digitatum, which was shown as inhibition of P. digitatum mycelium growth (). Moreover, at P < 1% level of significance, the mycelium inhibition rates caused by VOCs were stistically equal even thes effects were ranged from 35 to 79% for the four isolates, with Q004B and Q125B, providing the least and most inhibition, respectively. On the other hand, the tests of HCN production showed that the four isolates Q004B, Q110, Q112B and Q125B produce significantly HCN which could be one of the mechanism of actions of inhibition of P. digitatum.
Siderophore Production
The results of siderophores production indicated that all four Pseudomonas isolates tested were able to produce siderophores. They exhibited an orange halo after 5 days of incubation at 30°C on CAS agar plate and, therefore, were considered positive for siderophores production (). Among the strains, intensity of orange halo showed wide variations.
Chitinase Activity
The results of chitinase production indicated that among four Pseudomonas isolates tested, three isolates Q125B, Q112B, and Q004B were able to hydrolyze the chitin when grown on solid agar medium supplemented with colloidal chitin (0.2%) (). Clearing zones were observed around the colonies, which suggested that endochitinase activity was excreted from the cells. However, the isolate Q110B did not provided any chitinase activity.
Discussion
All four Pseudomonas isolates evaluated in this study (Q125B, Q112B, Q004B and Q110B) have potential as biocontrol agents against P. digitatum on citrus. Other research has reported that several species within the genus Pseudomonas are effective biocontrol agents against this pathogen (Gao et al., Citation2018; Kaur et al., Citation2007; Qessaoui et al., Citation2020). These Pseudomonas isolates (Q125B, Q112B, Q004B and Q110B) were all collected and isolated from tomato roots in greenhouse, and exhibit significant antifungal activity against P. digitatum in vitro (PDA medium) and in vivo (orange fruit under postharvest conditions). The cell-free supernatant of these isolates inhibited conidia germination. Huang et al. (Citation1995) reported that the isolates of P. cepacia (ID 2131) inhibited germination of P. digitatum and showed strong ability to protect wounded orange fruit from P. digitatum infection. Additionally, Errampalli and Brubacher (Citation2006) reported that P. syringae was effective on blue mold of apples caused by P. expansum. This inhibition can be explained by their ability to produce secreted bioactive components. Some beneficial rhizobacteria can reduce pathogenic infections directly by space competition, nutritional competition (niche exclusions), and/or secretion of antibiotics (Lugtenberg and Kamilova, Citation2009; Mitter et al., Citation2013), such as the lipopeptide, 2,4-diacetylphloroglucinol and phenazine-1-carboxylic acid (PCA) (Jaaffar et al., Citation2017; Paulin et al., Citation2017). In our study, Pseudomonas isolates (Q125B, Q112B, Q004B and Q110B) were able to produce HCN, chitinase and siderophore. Volatile antifungal compounds, such as HCN, produced by selected isolates played crucial roles in inhibiting P. digitatum (Pena et al., Citation2019). Our results are similar to those found in previous studies which revealed that Erwinia herbicola had a direct inhibition of spore germination of both fungi Botrytis cinerea and Penicillium expansum in liquid culture (Bryk et al., Citation1998). Moreover, Kumari and Khanna (Citation2014) found that the plant growth promoting rhizobacteria isolate (15B) significantly inhibited (64.2%) Fusarium wilt (F. oxysporum sp.ciceri) growth in chick pea (Cicer arietinum) by producing volatile compounds. Similarly, our study indicated that the four isolates of Pseudomonas evaluated produced significant amount of sidrophores and lytic enzymes. These findings agree with many studies reporting the ability of enzyme producing bacteria to destroy spores of phytopathogenic fungi (El-Tarabily, Citation2006). Additionally, Kong et al. (Citation2020) reported that the ST-TJ4 strain of Pseudomonas sp. uses siderophores, cellulase, and protease for antagonistic activity on 11 different pathogenic fungi, and this isolate has genes involved in the synthesis of phenazine, phenazine-1-carboxylic acid, pyrrolnitrin, and hydrogen cyanide. Furthermore, Pseudomonas rhizobacteria constitute a suitable potential biopesticides alternative for chemical fungicides in managing fungal diseases (Moin et al., Citation2020; Noreen et al., Citation2015). Thus, the rhizospheric soil constitutes an unexplored reservoir of biocontrol agents. Our investigation indicated that the Pseudomonas isolates evaluated exhibited strong inhibitory properties to efficaciously control P. digitatum mycelium growth and spore germination. This inhibition may be caused by volatile compound production, such as HCN or by diffusible substances released (chinitase, cellulase and others) or by both of these mechanisms at the same time. Finally, since these four Pseudomonas isolates could effectively be used to decrease the use of synthetic fungicide chemical sprays, their implementation opens new opportunities as potential bio-fungicides, which would be an environmental-friendly and sustainable alternative to current practices.
Siderophore is one of the main mechanisms of bacteria involved in the biological control of plant diseases. Both plant pathogenic fungi and bacteria are reported to be inhibited by siderophore-producing biocontrol agents (López-Reyes et al., Citation2017). These siderophores are produced in iron-limited conditions to sequester the less-available iron from the environment and thereby deprive the pathogen from iron, which ultimately leads to inhibition (Bhatia and Singh, Citation2021; Dhuldhaj and Pandya, Citation2021; Yu et al., Citation2011).
Disclosure statement
No potential conflict of interest was reported by the author(s).
Additional information
Funding
References
- Anticona M., J. Blesa, A. Frigola, and M. J. Esteve. 2020. High biological value compounds extraction from citruswaste with non-conventional methods. Foods. 9(6):811. doi:10.3390/foods9060811
- Askarne, L., I. Talibi, H. Boubaker, E.H. Boudyach, F. Msanda, B. Saadi, M.A. Serghini, and A. Ait Ben Aoumar. 2012. In vitro and in vivo antifungal activity of several Moroccan plants against Penicillium italicum, the causal agent of citrus blue mold. Crop Prot. 40:53–58. doi: 10.1016/j.cropro.2012.04.023.
- Bakker A. W., and B. Schippers.1987. Microbial cyanide production in the rhizosphere in relation to potato yield reduction and Pseudomonas SPP-mediated plant growth-stimulation. Soil Biol. Biochem. 19(4): 451–57. doi:10.1016/0038-0717(87)90037-X
- Bhatia, S., and S. Singh. 2021. Inhibition of siderophores in blocking fungal infection. Fungal Siderophores: From Mineral--Microbe Interactions to Anti-Pathogenicity, p. 13–31. In: Springer, Cham. doi: 10.1007/978-3-030-53077-8_2
- Boubaker, H., B. Saadi, E.H. Boudyach, and A.A. Benaoumar. 2009. Sensitivity of Penicillium digitatum and P. italicum to Imazalil and Thiabendazole in Morocco. Plant Pathol. J. 8(4):152–158. doi: 10.3923/ppj.2009.152.158.
- Brown, G., and W.R. Miller. 1999. Maintaining fruit healthy after harvest. APS Press St. Paul, MN, 175–188.
- Bryk, H., P. Sobiczewski, and B. Dyki. 1998. Antagonistic effect of Erwinia herbicola on in vitro spore germination and germ tube elongation of Botrytis cinerea and Penicillium expansum. BioControl. 43:97–106. doi: 10.1023/A:1009914612914.
- Cattelan, A.J., P.G. Hartel, and J.J. Fuhrmann. 1999. Screening for plant growth-promoting rhizobacteria to promote early soybean growth. Soil Sci. Soc. Am. J. 63:1670–1680. doi: 10.2136/sssaj1999.6361670x.
- Chernin, L., Z. Ismailov, S. Haran, and I. Chet. 1995. Chitinolytic enterobacter agglomerans antagonistic to fungal plant pathogens. Appl. Environ. Microbiol. 61:1720–1726. doi: 10.1128/aem.61.5.1720-1726.1995.
- Chidi, F., A. Bouhoudan, and M. Khaddor. 2020. Antifungal effect of the tea tree essential oil (Melaleuca alternifolia) against Penicillium griseofulvum and Penicillium verrucosum. J. King Saud Univ. Sci. 32:2041–2045. doi: 10.1016/j.jksus.2020.02.012.
- de Sousa Oliveira, M.I., A.A. Chaibub, T.P. Sousa, M.V.C.B. Cortes, A.C.A. de Souza, E.C. Da Conceição, and M.C.C. de Filippi. 2020. Formulations of Pseudomonas fluorescens and Burkholderia pyrrocinia control rice blast of upland rice cultivated under no-tillage system. Biol. Control. 144:104153. doi: 10.1016/j.biocontrol.2019.104153.
- Dhuldhaj, U., and U. Pandya. 2021. Diversity, function, and application of fungal iron chelators (Siderophores) for integrated disease management. In: G. Seneviratne, and J.S. Zavahir (eds.). Role of microbial communities for sustainability. Microorganisms for sustainability. p. 259–288. In Springer, Singapore . doi: 10.1007/978-981-15-9912-5_10
- Droby, S., V. Vinokur, B. Weiss, L. Cohen, A. Daus, E.E. Goldschmidt, and R. Porat. 2002. Induction of resistance to Penicillium digitatum in grapefruit by the yeast biocontrol agent Candida oleophila. Phytopathology. 92:393–399. doi: 10.1094/PHYTO.2002.92.4.393.
- El-Tarabily, K.A. 2006. Rhizosphere-competent isolates of streptomycete and non-streptomycete actinomycetes capable of producing cell-wall-degrading enzymes to control Pythium aphanidermatum damping-off disease of cucumber. Can. J. Bot. 84:211–222. doi: 10.1139/b05-153.
- Elshafie, H., I. Camele, R. Racioppi, L. Scrano, N. Iacobellis, S. Bufo, H.S. Elshafie, I. Camele, R. Racioppi, L. Scrano, et al. 2012. In vitro antifungal activity of Burkholderia gladioli pv. agaricicola against some phytopathogenic fungi. Int. J. Mol. Sci. 13:16291–16302. doi: 10.3390/ijms131216291.
- Errampalli, D., and N.R. Brubacher. 2006. Biological and integrated control of postharvest blue mold (Penicillium expansum) of apples by Pseudomonas syringae and cyprodinil. Biol. Control. 36:49–56. doi: 10.1016/J.BIOCONTROL.2005.07.011.
- Fiddaman, P.J., and S. Rossall. 1993. The production of antifungal volatiles by Bacillus subtilis. J. Appl. Bacteriol. 74:119–126. doi: 10.1111/j.1365-2672.1993.tb03004.x.
- Gao, P., J. Qin, D. Li, S. Zhou, and Y.-W. He. 2018. Inhibitory effect and possible mechanism of a Pseudomonas strain QBA5 against gray mold on tomato leaves and fruits caused by Botrytis cinerea. PLoS One. 13:e0190932. doi: 10.1371/journal.pone.0190932.
- Holmes, G.J., and J.W. Eckert. 1999. Sensitivity of Penicillium digitatum and P. italicum to postharvest Citrus Fungicides in California. Phytopathology. 89:716–721. doi: 10.1094/PHYTO.1999.89.9.716.
- Huang, Y., B.J. Deverall, and S.C. Morris. 1995. Postharvest control of green mould on oranges by a strain of Pseudomonas glathei and enhancement of its biocontrol by heat treatment. Postharvest Biol. Technol. 5:129–137. doi: 10.1016/0925-5214(94)00016-L.
- Hussien, A., Y. Ahmed, A.-H. Al-Essawy, and K. Youssef. 2018. Evaluation of different salt-amended electrolysed water to control postharvest moulds of citrus. Trop. Plant Pathol. 43:10–20. doi: 10.1007/s40858-017-0179-8.
- Jaaffar, A.K.M., J.A. Parejko, T.C. Paulitz, D.M. Weller, and L.S. Thomashow. 2017. Sensitivity of Rhizoctonia Isolates to Phenazine-1-Carboxylic acid and biological control by Phenazine-producing Pseudomonas spp. Phytopathology. 107:692–703. doi: 10.1094/PHYTO-07-16-0257-R.
- Janisiewicz, W.J., and J. Roitman. 1988. Biological control of blue mold and gray mould on apple and pear with Pseudomonas cepacia. Phytopathology 78(12):1697–1700. doi: 10.1094/Phyto-78-1697.
- Jaouad, M., A. Moinina, S. Ezrari, and R. Lahlali. 2020. Key pests and diseases of citrus trees with emphasis on root rot diseases: An overview. J. Agri. Sci. 1(3):149–160.
- Kanan, G.J., and R.A. Al-Najar. 2008. In vitro antifungal activities of various plant crude extracts and fractions against Citrus post-harvest disease agent Penicillium digitatum. Jordan J. Biol. Sci. 1:89–99.
- Kaur, R., R.S. Singh, and C. Alabouvette. 2007. Antagonistic activity of selected isolates of fluorescent Pseudomonas against Fusarium oxysporum f. sp. ciceri. Asian J. Plant Sci. 6:446–454. doi: 10.3923/ajps.2007.446.454.
- Kong, W.-L., P.-S. Li, X.-Q. Wu, T.-Y. Wu, and X.-R. Sun. 2020. Forest tree associated bacterial diffusible and volatile organic compounds against various phytopathogenic fungi. Microorganisms. 8:590. doi: 10.3390/microorganisms8040590.
- Kumari, S., and V. Khanna. 2014. Effect of antagonistic Rhizobacteria coinoculated with Mesorhizobium ciceris on control of Fusarium wilt in chickpea (Cicer arietinum L.). African J. Microbiol. Res. 8:1255–1265. doi: 10.5897/ajmr2013.6481.
- Leelasuphakul, W., P. Hemmanee, and S. Chuenchitt. 2008. Growth inhibitory properties of Bacillus subtilis strains and their metabolites against the green mold pathogen (Penicillium digitatum Sacc.) of citrus fruit. Postharvest Biol. Technol. 48:113–121. doi: 10.1016/j.postharvbio.2007.09.024.
- López-Reyes, L., M.G. Carcaño-Montiel, T.L. Lilia, G. Medina-de La Rosa, and T.H.R. Armando. 2017. Antifungal and growth-promoting activity of Azospirillum brasilense in Zea mays L. ssp. mexicana. Arch. Phytopathol. Pflanzenschutz 50(13–14):727–743. doi: 10.1080/03235408.2017.1372247.
- Lugtenberg, B., and F. Kamilova. 2009. Plant-growth-promoting rhizobacteria. Annu. Rev. Microbiol. 63:541–556. doi: 10.1146/annurev.micro.62.081307.162918.
- Mitter, B., A. Petric, P. SG Chain, F. Trognitz, J. Nowak, S. Compant, and A. Sessitsch. 2013. Genome analysis, ecology, and plant growth promotion of the endophyte Burkholderia phytofirmans strain PsJN, p. 865–874. In: Molecular microbial ecology of the rhizosphere. F. J. de Bruijn (Ed.),John Wiley & Sons, Inc, Hoboken, NJ, USA. doi: 10.1002/9781118297674.ch81.
- Moin, S., S.A. Ali, K.A. Hasan, A. Tariq, V. Sultana, J. Ara, and S. Ehteshamul-Haque. 2020. Managing the root rot disease of sunflower with endophytic fluorescent Pseudomonas associated with healthy plants. Crop Prot. 130:105066. doi: 10.1016/j.cropro.2019.105066.
- Noreen, R., S.A. Ali, K.A. Hasan, V. Sultana, J. Ara, and S. Ehteshamul-Haque. 2015. Evaluation of biocontrol potential of fluorescent Pseudomonas associated with root nodules of mungbean. Crop Prot. 75:18–24. doi: 10.1016/j.cropro.2015.04.018.
- Paulin, M.M., A. Novinscak, C. Lanteigne, V.J. Gadkar, and M. Filion. 2017. Interaction between 2,4-diacetylphloroglucinol- and hydrogen cyanide-producing Pseudomonas brassicacearum LBUM300 and Clavibacter michiganensis subsp. michiganensis in the tomato rhizosphere. Appl. Environ. Microbiol. 83:e00073–17. doi: 10.1128/AEM.00073-17.
- Pena, L.C., G.H. Jungklaus, D.C. Savi, L. Ferreira-Maba, A. Servienski, B.H.L.N.S. Maia, V. Annies, L.V. Galli-Terasawa, C. Glienke, and V. Kava. 2019. Muscodor brasiliensis sp. nov. produces volatile organic compounds with activity against Penicillium digitatum. Microbiol. Res. 221:28–35. doi: 10.1016/j.micres.2019.01.002.
- Qessaoui, R., A. Amarraque, H. Lahmyed, A. Ajerrar, E.H. Mayad, B. Chebli, A.S. Walters, R. Bouharroud, and K. Del-Claro. 2020. Inoculation of tomato plants with rhizobacteria suppresses development of whitefly Bemisia tabaci (GENNADIUS) (HEMIPTERA: ALEYRODIDAE): Agro-ecological application. PLoS One. 15:e0231496. doi: 10.1371/journal.pone.0231496.
- Qessaoui, R., H. Lahmyed, A. Ajerrar, J. Furze, T. Paulitz, M. Alouani, B. Chebli, E.H. Mayad, and B. R. 2021. Rhizospheric solutions: Pseudomonas isolates counter Botrytis cinerea on tomato. AFRIMED AJ –Al Awamia 131:50–72. http://196.200.148.132/index.php/AFRIMED/article/view/81/46
- Qessaoui, R., R. Bouharroud, J.N. Furze, M. El Aalaoui, H. Akroud, A. Amarraque, J. Vaerenbergh, R. Van Tahzima, E.H. Mayad, and B. Chebli. 2019. Applications of new rhizobacteria Pseudomonas isolates in agroecology via fundamental processes complementing plant growth. Sci. Rep. 9:12832. doi: 10.1038/s41598-019-49216-8.
- Romanazzi, G., S.M. Sanzani, Y. Bi, S. Tian, P.G. Martíneze, and N. Alkan. 2016. Induced resistance to control postharvest decay of fruit and vegetables. Postharvest Biol. Technol. 122:82–94. doi: 10.1016/j.postharvbio.2016.08.003.
- Rouissi, W., L. Ugolini, C. Martini, L. Lazzeri, and M. Mari. 2013. Control of postharvest fungal pathogens by antifungal compounds from Penicillium expansum. J. Food Prot. 76:1879–1886. doi: 10.4315/0362-028X.JFP-13-072.
- Schwyn, B., and J.B. Neilands. 1987. Universal chemical assay for the detection and determination of siderophores. Anal. Biochem. 160:47–56. doi: 10.1016/0003-2697(87)90612-9.
- Shrivastava, P., and R. Kumar. 2018. Actinobacteria: Eco-friendly candidates for control of plant diseases in a sustainable manner. New Futur. Dev. Microb. Biotechnol. Bioeng. 79–91. doi: 10.1016/B978-0-444-63994-3.00005-9.
- Spadaro, D., and M.L. Gullino. 2004. State of the art and future prospects of the biological control of postharvest fruit diseases. Int. J. Food Microbiol. 91:185–194. doi: 10.1016/S0168-1605(03)00380-5.
- Taqarort, N., A. Echairi, R. Chaussod, R. Nouaim, H. Boubaker, A.A. Benaoumar, and E. Boudyach. 2008. Screening and identification of epiphytic yeasts with potential for biological control of green mold of citrus fruits. World J. Microbiol. Biotechnol. 24:3031–3038. doi: 10.1007/s11274-008-9849-5.
- Torres-León, C., R. Parra-Saldívar, R. De La Cruz-quiroz, M. Tranier, S. Saldaña-Mendoza, S. Roussos, A.C. Flores-Gallegos, C.N. Aguilar, G. Martinez-Medina, K. Nathiely Ramírez-Guzmán, et al. 2018. Biocontrol agents as strategy of agro-ecosystem management to restitution of productive soils for food production, p. 185–213. In: Phytobiont and ecosystem restitution V. Kumar et al. (eds.),Springer, Singapore. doi: 10.1007/978-981-13-1187-1_10.
- Tripathi, P., and N. Dubey. 2004. Exploitation of natural products as an alternative strategy to control postharvest fungal rotting of fruit and vegetables. Postharvest Biol. Technol. 32:235–245. doi: 10.1016/J.POSTHARVBIO.2003.11.005.
- Valverde, C., G.G. Anta, and G. Ferraris. 2015. Pseudomonas and azospirillum, p. 389–409. Handbook for Azospirillum: Technical issues and protocols. In Cassán FD, Okon Y, Creus CM(eds), Springer International Publishing, Cham. Springer International Publishing. doi: 10.1007/978-3-319-06542-7_21.
- Wallace, R.L., D.L. Hirkala, and L.M. Nelson. 2017. Postharvest biological control of blue mold of apple by Pseudomonas fluorescens during commercial storage and potential modes of action. Postharvest Biol. Technol. 133:1–11. doi: 10.1016/j.postharvbio.2017.07.003.
- Wang, Z., M. Jiang, K. Chen, K. Wang, M. Du, Z. Zalán, F. Hegyi, and J. Kan. 2018. Biocontrol of Penicillium digitatum on postharvest citrus fruits by Pseudomonas fluorescens. J. Food Qual. 2018:1–10. doi: 10.1155/2018/2910481.
- Yu, X., C. Ai, L. Xin, and G. Zhou. 2011. The siderophore-producing bacterium, Bacillus subtilis CAS15, has a biocontrol effect on Fusarium wilt and promotes the growth of pepper. Eur. J. Soil Biol. 47(2):138–145. doi: 10.1016/J.EJSOBI.2010.11.001.
- Zhong, G., and E. Nicolosi. 2020. Citrus origin, diffusion, and economic importance. Springer, Cham, 5–21.
- Zhu, J.-W., Q. Xie, and H. Li. 2006. Occurrence of imazalil-resistant biotype of Penicillium digitatum in China and the resistant molecular mechanism. J. Zhejiang Univ. A. 7:362–365. doi: 10.1631/jzus.2006.as0362.