ABSTRACT
Several cell-intrinsic alterations have poor prognostic features in human breast cancer, as exemplified by the absence of MAP1LC3B/LC3B (microtubule-associated protein 1 light chain 3 β)-positive puncta in the cytoplasm (which indicates reduced autophagic flux) or the loss of nuclear HMGB1 expression by malignant cells. It is well established that breast cancer is under strong immunosurveillance, as reflected by the fact that scarce infiltration of the malignant lesion by CD8+ cytotoxic T lymphocytes or comparatively dense infiltration by immunosuppressive cell types (such as FOXP3+ regulatory T cells or CD68+ tumor-associated macrophages), resulting in low CD8+:FOXP3+ or CD8+:CD68+ ratios, has a negative prognostic impact. Here, we reveal the surprising finding that cell-intrinsic features may influence the composition of the immune infiltrate in human breast cancer. Thus, the absence of LC3B puncta is correlated with intratumoral (but not peritumoral) infiltration by fewer CD8+ cells and more FOXP3+ or CD68+ cells, resulting in a major drop in the CD8+:FOXP3+ or CD8+:CD68+ ratios. Moreover, absence of HMGB1 expression in nuclei correlated with a general drop in all immune effectors, in particular FOXP3+ and CD68+ cells, both within the tumor and close to it. Combined analysis of LC3B puncta and HMGB1 expression allowed for improved stratification of patients with respect to the characteristics of their immune infiltrate as well as overall and metastasis-free survival. It can be speculated that blocked autophagy in, or HMGB1 loss from, cancer cells may favor tumor progression due to their negative impact on anticancer immunosurveillance.
Introduction
The relationship between autophagy and cancer is complex and possibly context-dependent.Citation1 In the specific case of breast cancer, there is some evidence that inhibition of autophagy may favor oncogenesis and tumor progression. Thus, BECN1/Beclin-1, the gene that codes for the essential autophagy protein BECN1, frequently undergoes loss of heterozygosity in breast cancer cells,Citation2 and low BECN1 mRNA expression has a negative prognostic effect.Citation3 Moreover, in mice, heterozygosity of Becn1, which induces a partial autophagy defect,Citation4 accelerates breast carcinogenesis in 2 different models, namely in multiparous FVB/N mice, as well as in cooperation with the transgenic expression of the Wnt1 oncogene.Citation5
High expression of the 2 autophagy-relevant proteins ATG5 and RB1CC1/FIP200 has a positive impact on breast cancer patient survival.Citation6 Although the absolute expression level of MAP1LC3B/LC3B (microtubule-associated protein 1 light chain 3 β) has no major impact on patient survival,Citation7 the absence of LC3B puncta, which correlates with increased levels of the autophagy receptor SQSTM1/p62 and hence indicates a reduction of autophagic flux, has a negative prognostic impact, as we have reported on 2 cohorts comprising approximately 1700 breast cancer patients who underwent adjuvant chemotherapy.Citation8 Risk stratification can be further improved when we measure, in addition to LC3B puncta, the nuclear expression of HMGB1. Those patients who were characterized by low autophagy and low HMGB1 expression had a particularly poor overall and progression-free survival.Citation8
Autophagy is considered an oncosuppressive phenomenon because it can inhibit a series of cellular phenomena that contribute to oncogenesis,Citation1 namely the maintenance of a normal cellular metabolism,Citation9 antimicrobial effects including against oncogenic viruses,Citation10 maintenance of normal (as opposed to transformed) stem cell properties,Citation5 preservation of genomic stability,Citation11 antiinflammatory functions,Citation12 activation of oncogene-induced senescence or cell deathCitation13 and the degradation of potentially oncogenic proteins.Citation14,15 Beyond these cell-autonomous effects of autophagy, recent results also suggest an effect of autophagy on anticancer immunosurveillance.Citation16,17 Indeed, in mice, KRAS-induced lung cancer tends to develop earlier upon tumor-cell-specific conditional knockout of the essential autophagy gene Atg5, at least during the early phase of oncogenesis, and this effect is accompanied by an increased infiltration of the tumor by immunosuppressive regulatory T cells (Treg). Physical elimination or functional inhibition of Treg reversed this oncogenesis-accelerating consequence of Atg5 depletion,Citation17 establishing a cause-effect relationship between defective autophagy in malignant cells and failing immunosurveillance. Similarly, autophagy-deficient colorectal cancers and lung adenocarcinomas fail to elicit protective anticancer immune responses when they are used as vaccines subsequent to their in vitro stimulation with anthracyclines or oxaliplatine.Citation18 Autophagy-deficient carcinomas and melanomas developing in immunocompetent mice also fail to attract immune effects upon treatment by chemotherapy or radiotherapy, correlating with markedly reduced treatment responses.Citation16,18-20
Intrigued by this putative link between autophagy and immunosurveillance, which is suggested by experiments in mice, we decided to investigate the possible relationship between autophagy and the immune infiltrate in human breast cancer, a pathology that is under strong immunosurveillance. Indeed, the density of tumor-infiltrating lymphocytes (TIL), which can be easily determined by conventional hematoxylin-eosin staining has a strongly positive prognostic impact.Citation21-24 TIL density is also a favorable predictive marker, meaning that breast cancer that are heavily infiltrated by lymphocytes exhibit a longer progression-free and overall survival upon anthracycline- or taxane-based chemotherapies.Citation21-24 A more refined analysis, based on the immunohistochemical detection of cytotoxic T lymphocytes (CTL, which express CD8), which are considered as the anticancer effector cells and immunosuppressive elements such as Tregs (which express FOXP3) and tumor-associated macrophages (TAM, which express CD68), allowed to calculate ratios (namely, the CD8+:FOX3+ and the CD8+:CD68+ ratios) that have an even higher prognostic and predictive power than TIL density.Citation25-30 Beyond any doubt, local immune responses influence the fate of breast cancer patients.Citation31
Here, we provide evidence that cytoplasmic LC3B puncta and nuclear HMGB1 expression in breast cancer cells exhibit a strong correlation with local immune parameters, in a representative cohort of breast cancer patient samples. Our results plead in favor of a major influence of the intracellular milieu within cancer cells on the immunosurveillance system.
Results
Prognostic significance of immune-related parameters in breast cancer patients
Tissue microarrays comprising surgical specimens from 152 breast cancer patients (Table S1), the same as the derivation cohort in Ref. 8, were subjected to the immunohistochemical quantification of the immune infiltrate to determine the density of CD8+ cytotoxic T lymphocytes (CTL, ), FOXP3+ regulatory T cells (Treg, ), and CD68+ tumor-associated macrophages (TAM, ) both within the malignant lesion (“intra”) or close to it tumor (“peri”). The density of the infiltrate by these distinct subsets was correlated among each other in a positive fashion ( and S1), reflecting the fact that macrophage-rich breast cancers tend to contain high densities of the 2 lymphocyte subpopulations and vice versa.Citation23,32, 31 On this cohort, we have previously determined the presence of cytoplasmic LC3B puncta, which reflects autophagic flux (because they correlate with reduction in the expression level of the autophagic receptor SQSTM1), in malignant cells (), as well as the expression of HMGB1 ().Citation8 As reported for this cohort, as well as for a larger cohort,Citation8 patients bearing tumors that are both positive for LC3B puncta and HMGB1 expression have the most favorable prognosis, both at the level of overall survival (OS, ) and metastasis-free survival (MFS, ), while patients with cancer that lack both LC3B puncta and HMGB1 are characterized by a dismal prognosis. Moreover, in accord with the literature,Citation26,27 a high ratio of CD8+ over FOXP3+ (or CD3+ over CD68+) tumor infiltrating cells (labeled as “intra”) had a positive impact on OS (Fig. S2) and MFS (). In contrast, if the same ratios were calculated for the tumor-adjacent area (labeled as “peri”), they failed to correlate with patient survival (Figs. S2 and ). These correlations could be calculated for optimal cut-off values and were significant after adjustment for clinical parameters ( and S2). Altogether, the cohort examined here confirms the positive impact of immunosurveillance on BC patient survival.
Figure 1. Representative immunohistochemical staining patterns. Breast cancer specimen were stained for the detection of CD8+ cytotoxic T lymphocytes (A), FOXP3+ regulatory T cells (B), CD68+ tumor-associated macrophages (C) and representative samples exemplifying distinct levels of infiltration are shown (upper panel: high infiltration, lower panel: low infiltration). The density of the immune infiltrate in the intratumoral and peritumoral area was quantified, and the different values were correlated among each other (D). The color code in (D) indicates the Spearman correlation coefficients. In addition, tissue sections were stained for the detection of LC3B (E) and HMGB1 (F). In each case, 2 representative tumor samples with distinct staining patterns are shown (upper panel: positive tumor, lower panel: negative tumor). Scale bar is indicated for each staining.
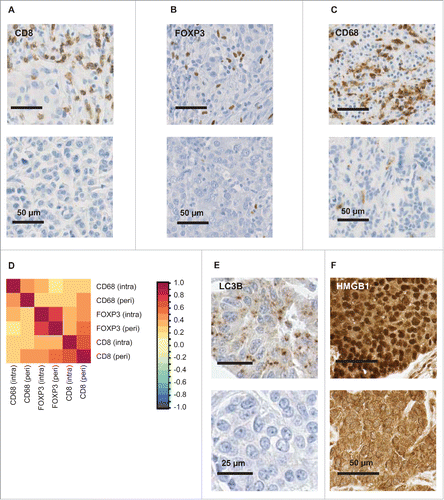
Figure 2. Stratification of patients based on cancer cell-intrinsic parameters. Patients were classified into 3 categories, namely (i) tumors with < median LC3B puncta (in < 10% of the malignant cells) and nuclear HMGB1 (HM) expression (in < 50% of the cancer cell), (ii) tumors that had either < median LC3B puncta or < median HMGB1 expression, and (iii) tumors with > median LC3B puncta and nuclear HMGB1 expression. Overall survival (A) and metastasis-free survival (B) are plotted and significance was calculated as indicated in Patients and Methods.
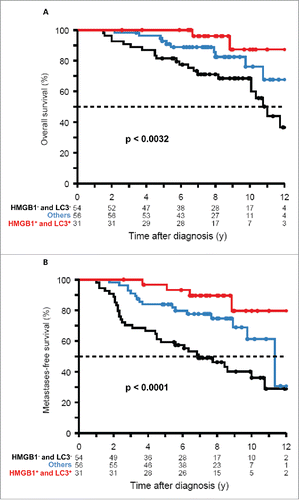
Figure 3. Stratification of patients based on immune parameters. The CD8+/FOXP3+ (A, B) and CD8+:CD68+ ratios (C, D) determined for the intratumoral (A, C) or peritumoral (B, D) immune infiltrates were subjected to calculations to determine optimal cut off values (CO, red lines), as defined by the zero-crossing of the martingale residual against the parameter. Moreover, the adjusted P values were plotted against all values. Finally, metastasis-free survival (MFS) was plotted for patients with values > median (blue) versus patients with values< median (black) or patients with values > CO (red) vs. patients with values < CO (black). Unadjusted and adjusted p-values are indicated.
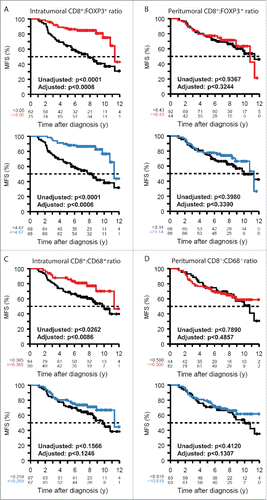
Correlation of LC3B puncta and the immune infiltrate in breast cancer
The percentage of malignant cells bearing LC3B puncta positively correlated with the frequency of intratumoral CD8+ T lymphocytes (), yet negatively correlated with that of FOXP3+ T cells () and CD68+ macrophages (). In contrast, LC3B puncta had no significant correlation with the density of the peritumoral immune infiltrate (, D, F), arguing in favor of local (rather than systemic) effects that determine the correlation between the cell-autonomous autophagic response and the immune contexture. Logically, the ratios of intratumoral (but not peritumoral) CD8+:FOXP3+ and CD8+:CD68+ cells exhibited a high (P < 0.0001) positive correlation with LC3B puncta in malignant cells (). Hence, those tumors that exhibit the highest level of autophagy are also those that manifest the most favorable immune effector:suppressor ratio.
Figure 4. Correlations between LC3B puncta and the density of the immune infiltrate. The percentage of cancer cells containing LC3B puncta was correlated with the density of the immune infiltrate measured for CD8+ (A, B) FOXP3+ (C, D) and CD68+ cells (E,F) within malignant lesions (intra, A, C, E) or in their periphery (peri, B, D, F). r = pseudo correlation as indicated in the Patients and Methods section.
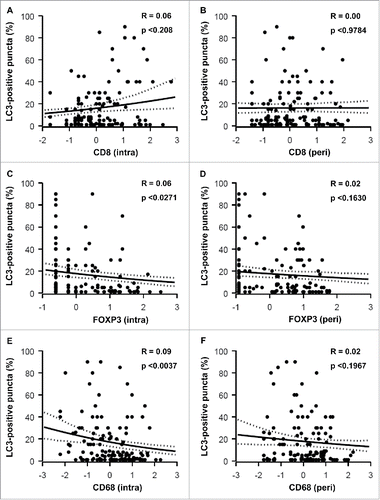
Correlation of HMGB1 expression and the immune infiltrate
The percentage of HMGB1+ nuclei within malignant cells did not significantly correlate with the frequency of CD8+ CTL within the tumor bed () or its vicinity (). However, HMGB1 expression did exhibit a significant negative correlation with the frequency of both intraepithelial () and peritumoral () infiltration by FOXP3+ Treg (with P-values of P < 0.0206 and P < 0.0057, respectively). This negative correlation was even more significant (P < 0.0001), when calculated between HMGB1 expression and the intratumoral () and peritumoral () infiltration by CD68+ TAM. It is important to emphasize that, in contrast to LC3B puncta, which only correlate with the intratumoral immune infiltrate (see above: ), HMGB1 expression correlates with (and presumably influences) the composition of both the local and the peritumoral immune infiltrate. HMGB1 expression failed to correlate with the CD8+:FOXP3+ and CD8+:CD68+ ratios, with the sole exception of the peritumoral CD8+:FOXP3+ ratio (), again underscoring the likely impact of HMGB1 on the recruitment of immune effectors into the vicinity (rather than into the core) of the malignant lesion.
Figure 5. Correlations between LC3B puncta and CD8+:FOXP3+ and CD8+:CD68+ ratios. CD8+:FOXP3+ (A, intra; B, peri) and CD8+:CD68+ (C, intra; D, peri) ratios were determined for each patient sample and plotted against the percentage of malignant cells bearing LC3B puncta. r = pseudo correlation as indicated in the Patients and Methods section.
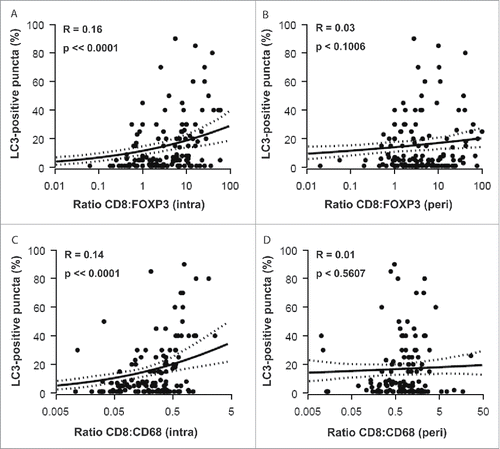
Figure 6. Correlations between HMGB1 expression and the density of the immune infiltrate. The percentage of cancer cells containing HMGB1-positive nuclei was correlated with the density of the immune infiltrate measured for CD8+ (A, intra; B, peri) FOXP3+ (C, intra; D, peri) and CD68+ cells (E, intra; F, peri). r = pseudo correlation as indicated in the Patients and Methods section.
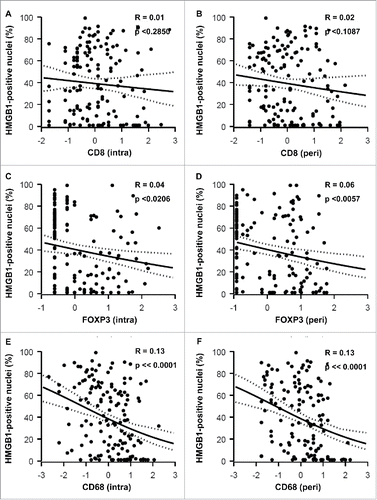
Figure 7. Correlations between HMGB1 expression and CD8+ : FOXP3+ and CD8+ : CD68+ ratios. CD8+: FOXP3+ (A, intra; B, peri) and CD8+: CD68+ (C, intra; D, peri) ratios were determined for each patient sample and plotted against the percentage of malignant cells containing HMGB1-positive nuclei. r = pseudo correlation as indicated in Patients and Methods section.
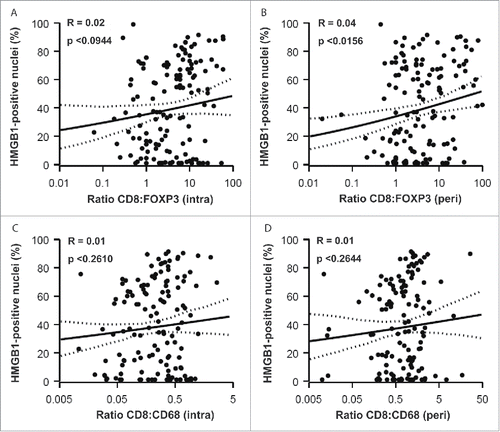
Combined impact of LC3B and HMGB1 on the immune infiltrate
In breast cancer cells, cytoplasmic LC3B puncta do not correlate with nuclear HMGB1 expression,Citation8 indicating that the 2 parameters can be investigated independently from each other with respect to their possible effects on the immune infiltrate. As a result, we classified patients (as in ) according to these 2 parameters into 3 subgroups, namely (i) those with dismal prognosis (and hence low LC3B puncta and HMGB1 expression < median), (ii) those with intermediate prognosis (and hence either low LC3B puncta or low HMGB1 expression), and (iii) those with good prognosis (and hence LC3B puncta and HMGB1 expression > median). Subsequent multiparameter correlation analyses revealed that good tumor cell-intrinsic prognostic features correlated with favorable intratumoral CD8+:FOXP3+ (, P < 0.0001) and CD8+:CD68+ (, P < 0.0004) ratios and, though to a lesser extent, with the peritumoral CD8+:FOXP3+ ratio (, P < 0.0114), but not (, P < 0.1372) with the peritumoral CD8+:CD68+ ratio. Altogether, these results underscore the correlation between cancer cell-intrinsic and microenvironmental prognostic features.
Figure 8. Combined impact of LC3B puncta and HMGB1 expression on CD8+: FOXP3+ and CD8+: CD68+ ratios. Intratumoral (A, C) and peritumoral (B, D) CD8+: FOXP3+ or CD8+: CD68+ ratios are represented for each patient category (with LC3B puncta and HMGB1 expression< median: HMGB1−LC3−; with either LC3B puncta or HMGB1 expression < median: (others); and with both LC3B puncta and HMGB1 expression > median: HMGB1+LC3+.
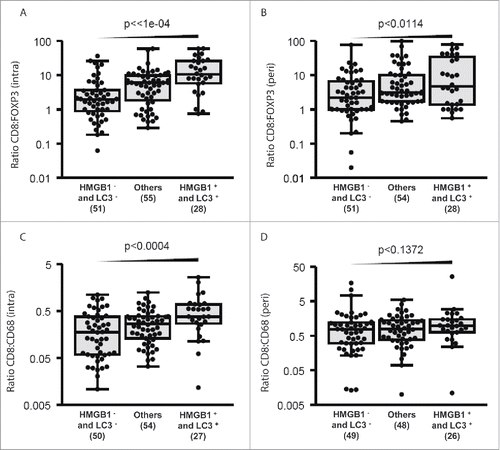
Discussion
In general terms, this work reveals a correlation between, on one hand, signs of active local immunosurveillance and, on the other hand, 2 characteristics of malignant cells that have favorable prognostic features (the presence of LC3B puncta and nuclear HMGB1 expression), favoring the hypothesis that there must be a relationship between cancer cell-specific stress pathways (that lead to autophagy and HMGB1 loss) and the immune microenvironment. Of note such relationships are subtle in the sense that LC3B puncta (but not nuclear HMGB1 expression) positively correlates with the intratumoral infiltration by CD8+ CTL, while both LC3B puncta and HMGB1 expression negatively correlate with the infiltration by immunosuppressive cell types, namely FOXP3+ Treg and CD68+ TAM. While LC3B puncta had a strictly local effect (and hence failed to affect peritumoral immune parameters), HMGB1 expression negative correlated with the density of the peritumoral FOXP3+ and CD68+ infiltrates. The existence of such subtle differences between cell-intrinsic/immune correlates appears particularly intriguing in view of the fact that, generally spoken, there was a positive correlation among the density of all immune cell types (Fig. S1), in line with retrospective multicenter studies, as well as microarray-based meta-analyses.Citation31-35 Thus, it appears that cell-intrinsic signs of stress can influence (or are influenced by) the complex properties of the immune infiltrate at the tumor site.
Correlative data obtained on patient samples without longitudinal analysis preclude the establishment of cause-effect relationships. Indeed, it is possible that intracellular stress is communicated to the microenvironment (for instance to attract immune effectors into the tumor bed),Citation36,37 but it is also possible that the immune response against neoplastic cells causes stress response or even the elimination of selective tumor cell populations (immunoselection).Citation38 Nonetheless, experimentation in mice indicate that autophagy can favor the release of the immunostimulatory chemotactic factor ATP by stressed cancer cells,Citation18,39 yet restrains the local production of adenosine, which would favor the recruitment of immunosuppressive Treg.Citation17 Thus, depletion of essential autophagy-relevant gene products (such as ATG5, ATG7, ATG10, ATG12 or BECN1) from transplantable murine cancer cells reduces their release of ATP in response to anthracycline chemotherapy in vitro Citation18,39 and abolishes their capacity to elicit protective anticancer immune responses in vivo, when injected into immunocompetent mice, in conditions in which autophagy-competent control cells do vaccinate against rechallenge with live cancer cells.Citation18 Similarly, autophagy-deficient tumors (due to transfection with shRNAs targeting Atg5 or Atg7) established in mice release less ATP than their autophagy-competent counterparts when treated with chemotherapy in vivo, fail to attract immune cells into the tumor bed, and do not reduce their growth in response to chemotherapyCitation16,18 or radiotherapy.Citation20 This deficient anticancer immune response could be restored by artificially increasing the extracellular ATP concentration, namely by injecting pharmacological inhibitors of extracellular ATPases, either together with the autophagy-deficient, anthracycline-treated suspension of dying cells (in vaccination experiments) or directly into the autophagy-deficient tumor concomitantly with systemic chemotherapy.Citation18 We also generated a mouse model of KRAS-induced lung carcinogenesis in which Atg5 could be inactivated within the transforming pneumocytes.Citation17 In this latter model, early oncogenesis is accelerated by Atg5 deletion, correlating with enhanced expression of ENTPD1 (ectonucleoside triphosphate diphosphohydrolase 1) (which catalyzes the first step of extracellular ATP degradation leading in fine to the generation of adenosine), as well as an increased local infiltration of the incipient neoplastic lesions by Tregs. In this model, inhibition of ENTPD1, neutralization of adenosinergic receptors or antibody-mediated depletion of Tregs abolishes the acceleration of oncogenesis induced by Atg5 deletion, underscoring the positive contribution of autophagy to immunosurveillance.Citation17,40
HMGB1 has also been involved in the stimulation of immune responses, correlating with the fact that extracellular HMGB1 can bind to TLR4 (and other pathogen recognition receptors such as AGER), thereby affecting the maturation and function of antigen-presenting cells including that of dendritic cells.Citation41,42 Cancer cells from which HMGB1 is depleted (by knockdown of the corresponding gene) or that lose HMGB1 expression spontaneously during tumor progression become unable to elicit anticancer immune responses in the 2 model system mentioned above, namely in vaccination assays (in which dying cells are injected subcutaneously into histocompatible, immunocompetent mice, followed by rechallenge with live cells one week later) and in chemotherapy experiments (in which anthracyclines are administered to mice bearing established tumors and immune-dependent tumor growth reduction is monitored).Citation41,42 This defective response can be overcome by injecting a synthetic TLR4 ligand together with dying cancer cells (in vaccination experiments) or into the tumor (in chemotherapy experiments).Citation42 Accordingly, tlr4−/− mice are unable to mount an immune response against dying cells, meaning that cancers established on such mice do not respond to chemotherapy or radiotherapy.Citation41 Similarly, patients with breast cancer bearing a loss-of-function mutation of TLR4 that reduces its affinity for HMGB1Citation41 are characterized by poor progression-free and overall survival after adjuvant chemotherapy, as compared to individuals bearing normal TLR4 alleles.Citation41,43 These observations lend support to the importance of the HMGB1-TLR4 interaction for anticancer immunosurveillance.
In conclusion, the results presented here are compatible with the hypothesis that the absence of autophagy and the missing expression of HMGB1 have a poor prognostic value because they negatively affect the capacity of the immune system to keep the neoplastic lesion in check.
Patients and methods
Patients
The patient cohort involved 152 patients treated at Georges François Leclerc Cancer Center (Dijon, France) for localized HER2 negative-BC between 1998 and 2007. After surgical removal of the primary tumor, patients were treated with 6 cycles of anthracyclines-based adjuvant chemotherapy (FEC100: epirubicin 100 mg/m2, cyclophosphamide 500 mg/m2, and 5-fluorouracil 500 mg/m2). After adjuvant chemotherapy, all patients with hormone receptor-positive disease received endocrine therapy for 5 y. Radiation therapy was given to all patients treated with conservative surgery and was performed after mastectomy if recommended according to the local risk of relapse. For each patient formalin-fixed paraffin-embedded (FFPE) tissue blocks of BC surgical specimen were retrieved from the department of pathology, and 4-µm-thick slides were stained for hematoxylin, CD8, CD68, FOXP3, HMGB1 and LC3B. The study was approved by the local ethical committee, and all patients gave written informed consent at the time of the diagnosis for the use of tumor samples for research purposes (national French authorization number: AC-2008-69).
Immunohistochemistry
HMGB1 staining: Formalin-fixed, paraffin-embedded cancer tissue sections were deparaffinized with 3 successive passages through xylene, and rehydrated through decreasing concentrations (100%, 95%, 80%, 70% and 50%) of ethanol. Antigen retrieval was carried out by heating slides for 20 min in pH 6.0 citrate buffer (10 mM sodium citrate, 0.05% Tween 20 [Sigma-Aldrich, P1379]) at 98°C. Endogenous peroxidase activity was inhibited with 3% hydrogen peroxidase (DAKO, S2001) for 10 min. Sections were then saturated 20 min with Protein Block Serum Free (DAKO, X0909). Without washing, the primary antibody, a polyclonal rabbit anti HMGB1 antibody (Thermo Fisher Scientific/Pierce, PA1-16926), was incubated overnight, followed by the secondary antibody (EnVision-Rabbit, DAKO K4011) for 30 min, and streptavidin-horse radish peroxidase (DAKO, P0397) was added for an additional 30 min. Peroxidase activity was revealed by means of daminobenzidine substrate (DAB; DAKO, K3468), and the sections were counterstained with Mayer hematoxylin (DAKO, S3309).Citation42
LC3B staining
We have previously described a validated immunohistochemical protocol for the detection of LC3B puncta in human FFPE cancer specimens.Citation44 Briefly, immunohistochemical staining of cancer tissue sections was performed using the Novolink Kit (Menarini Diagnostics, RE7140-K). Deparaffinized and heated tissue sections (as above) were allowed to cool down (45 min, room temperature) and mounted on Shandon Sequenza coverplates (Thermo Fisher Scientific, 72-199-50) in distilled water, and then washed twice for 5 min with 0.1% Tween 20 (v/v) in phosphate-buffered saline (PBS; Life Technologies, 10010–023). Thereafter, sections were incubated for 5 min with the Peroxidase Block reagent (Novolink kit, Menarini Diagnostics, RE7140-K), and subsequently washed twice for 5 min with 0.1% Tween 20 (v/v in PBS). Following a 5-min-long incubation at room temperature with the Protein Block reagent (Novolink kit; Menarini Diagnostics, RE7140-K), tissue sections were washed twice for 5 min with 0.1% Tween 20 (v/v in PBS), and then incubated overnight at 4°C with a primary antibody specific for LC3B (clone 5F10; Nanotools, 0231–100), dissolved in 1% bovine serum albumin (Sigma Aldrich, A2058) w/v in Tris-buffered saline (Sigma-Aldrich, T5912) at the final concentration of 25 µg/mL. This antibody recognizes both the soluble (LC3-I) and the lipidated membrane-bound form (LC3-II) of LC3B. After 2 washes in 0.1% Tween 20 (v/v in PBS), sections were incubated for 30 min with the Post Primary Block reagent (Novolink kit), washed again as before and incubated for 30 min with secondary antibodies coupled to horseradish peroxidase. Upon 2 additional washes, secondary antibodies were revealed with the liquid DAB Substrate Chromogen system (Novolink kit; 10 min). Finally, slides were washed in distilled water, and counterstained with hematoxylin.
CD8, Foxp3 and CD68 staining
Immunoperoxidase staining for CD8 (clone 1A5: Novocastra reagents, (NCL-L-CD8-295)) and CD68 (clone KP1; DAKO, (M 0814)) was performed using the Ventana Benchmark XT automated slide preparation system at the department of pathology from the Institut Gustave Roussy. Immunoperoxidase staining was performed on the FFPE tissues of all subjects. Tissue sections (4- to 5-µm thickness) were deparaffinized (EZ-Prep; Ventana Medical Systems, (950–102) at 75°C) followed by antigen-retrieval (Cell Conditioning 1; Ventana Medical Systems, (950–124) at 95 to 100°C). Antibodies were incubated at room temperature for 30 min, at 1:25 (CD8) and 1:150 (CD68) dilutions. Antibody staining was developed using the UltraView Universal DAB detection system (Ventana Medical Systems), and accompanied by hematoxylin counterstain. For regulatory T cells immunostaining, monoclonal antibody against the Treg cell marker FOXP3 (Abcam, ab450) was used at 4 µg/ml. Antigen retrieval was carried out by heating slides for 30 min at 98°C in 1 mmol/L EDTA, pH 9. Labeling was detected using the Leica, Novolink Polymer DS system (Leica Biosystems). The stained arrays were counterstained with hematoxylin.
Positive and negative staining controls were carried out with paraffin tonsil sections using CD8, CD68 and FOXP3 antibodies, and an isotype-matched negative control antibody.
Pathological assessment
For HMGB1 staining, the pattern of expression (nuclear or not) was evaluated in tumor cells: strong nuclear staining of at least 50% of the tumor cells was considered positive for HMGB1 tumor expression. The presence of LC3B puncta in tumor cells and the percentage of tumor cells with detectable LC3B puncta were assessed, independently of the intensity of diffuse cytoplasmic staining. Considering the possibility of tumor heterogeneity, LC3B pathological evaluations were done on the whole tumor area and at least 15 high-power fields (x20). HMGB1 and LC3B expressions were independently assessed by a trained histologist (SL) and a trained BC-pathologist (LA), both blinded for clinicopathological data. Discrepancies between the 2 observers were reviewed jointly to reach consensus. HMGB1 expression and LC3B expression were evaluated separately and correlated with CD8+, FOXP3+, and CD68+ cells infiltrates on surgical specimen, and long-term patient outcome.
Positive hormone receptor status was defined as 10% of tumor cells expressing either estrogen or progesterone receptor.
Quantification of immune cells
Levels of immune cells infiltration were evaluated by a trained histologist (SL). All samples were previously anonymized and blinded to the clinicopathologic data. On each slide, the number of stained cells was quantified in at least 5 high power fields (20X) both in tumor islets (“intratumoral”) and in adjacent stroma (“peritumoral”). For each staining, the total number of stained cells in each location was determined by the arithmetic mean.
Statistical analysis
Data analyses and representations were performed within the statistical environment R. Individual data points representing the measurement from one patient are systematically graphed alongside with the box and whiskers plot calculated from the corresponding distribution. Effects estimation on continuous variables were assessed by means of negative binomial regression for immunological parameters, linear modeling on the log2-preprocessed ratios between them and β regression for LC3, and HMGB1 responses. For discrete response, incidences were computed by Firth penalized-likelihood logistic or proportional-odds logistic regressions with the logistic link function. Overall survival (OS) and Metastasis Free Survival (MFS) were determined from the date of randomization were censored at the last follow-up date or 12 y (9 patients). Survival curves were estimated by the Kaplan-Meier product-limit method and their distributions compared by Cox proportional hazards regression. Multivariate adjustment was performed on pN (N0 vs. N1 vs. N2/3), pT (T1 vs. T2 vs. T3/4), tumor grade (I/II vs. III) and cancer subtype (triple negative vs. luminal). Unless stated, P values are 2-sided, reported together with the back-transformed 95% confidence intervals for the statistic of interest and considered significant when < 0.05.
Preliminary to any correlation studies, raw immunological parameters were transformed to z-scores on the basis they follow a negative binomial distribution. When regressing to HMGB1 and LC3, pseudo R correspond to the correlation between the linear predictor and logit-transformed response, elsewhere common Pearson (r in the figure) and Spearman (Rho in the figure) correlation coefficients apply.
To determine adequate cutoff values allowing to patient survival stratification, methods of the martingale residuals for the MFS Cox regression model, and of the log-rank statistics all possible cutpoints, were used.Citation45
Abbreviations
AGER | = | advanced glycosylation end product-specific receptor |
ATG7 | = | autophagy-related 7 |
ATG10 | = | autophagy-related 10 |
ATG12 | = | autophagy-related 12 |
ATG5, | = | autophagy-related 7 |
ATP | = | adenosine tri-phosphate |
BC, | = | breast cancer |
BECN1 | = | Beclin 1 autophagy related |
CD68, | = | cluster of differentiation 68 |
CD8, | = | cluster of differentiation 8 |
CTL, | = | cytotoxic T lymphocyte |
EDTA | = | ethylenediaminetetraacetic acid |
ENTPD1 | = | ectonucleoside triphosphate diphosphohydrolase 1 |
FFPE | = | formalin-fixed paraffin-embedded |
FOXP3 | = | forkhead box P3 |
HMGB1 | = | high mobility group box 1 |
KRAS | = | Kirsten rat sarcoma viral oncogene homolog |
MAP1LC3B/LC3B | = | microtubule-associated protein 1 light chain 3 β |
MFS | = | metastasis free survival |
mRNA | = | messenger ribonucleic acid |
OS, | = | overall survival |
PBS, | = | phosphate-buffered saline |
SQSTM1/p62 | = | sequestosome 1 |
TAM | = | tumor-associated macrophage |
TIL, | = | tumor-infiltrating lymphocyte |
TLR4 | = | toll like receptor 4 |
WNT1 | = | wingless-type MMTV integration site family member 1 |
Disclosure of potential conflicts of interest
None of the authors needed to declare a conflict of interest.
Supplemental_Material.zip
Download Zip (550.5 KB)Acknowledgments
The authors would like to thank Mrs S Piterboth, and Pr J-Y. Scoazec from the Department of Biology and Pathology of Gustave Roussy Institute (Villejuif) for their help in immunostaining.
Funding
GK and LZ are supported by the Ligue contre le Cancer (équipes labelisées); Agence Nationale de la Recherche (ANR); Association pour la recherche sur le cancer (ARC); Cancéropôle Ile-de-France; Institut National du Cancer (INCa); Fondation Bettencourt-Schueller; Fondation de France; Fondation pour la Recherche Médicale (FRM); the European Commission (ArtForce); the European Research Council (ERC for GK); the LabEx Immuno-Oncology; the SIRIC Stratified Oncology Cell DNA Repair and Tumor Immune Elimination (SOCRATE); the SIRIC Cancer Research and Personalized Medicine (CARPEM); and the Paris Alliance of Cancer Research Institutes (PACRI). LZ was supported by ISREC and Swiss Bridge Foundation.
References
- Galluzzi L, Pietrocola F, Bravo-San Pedro JM, Amaravadi RK, Baehrecke EH, Cecconi F, Codogno P, Debnath J, Gewirtz DA, Karantza V, et al. Autophagy in malignant transformation and cancer progression. EMBO J 2015; 34:856-80; PMID:25712477; http://dx.doi.org/10.15252/embj.201490784
- Liang XH, Jackson S, Seaman M, Brown K, Kempkes B, Hibshoosh H, Levine B. Induction of autophagy and inhibition of tumorigenesis by beclin 1. Nature 1999; 402:672-6; PMID:10604474; http://dx.doi.org/10.1038/45257
- Tang H, Sebti S, Titone R, Zhou Y, Isidoro C, Ross TS, Hibshoosh H, Xiao G, Packer M, Xie Y, et al. Decreased mRNA Expression in Human Breast Cancer is Associated with Estrogen Receptor-Negative Subtypes and Poor Prognosis. EBioMedicine 2015; 2:255-63; PMID:25825707; http://dx.doi.org/10.1016/j.ebiom.2015.01.008
- Qu X, Yu J, Bhagat G, Furuya N, Hibshoosh H, Troxel A, Rosen J, Eskelinen EL, Mizushima N, Ohsumi Y, et al. Promotion of tumorigenesis by heterozygous disruption of the beclin 1 autophagy gene. J Clin Invest 2003; 112:1809-20; PMID:14638851; http://dx.doi.org/10.1172/JCI20039
- Cicchini M, Chakrabarti R, Kongara S, Price S, Nahar R, Lozy F, Zhong H, Vazquez A, Kang Y, Karantza V. Autophagy regulator BECN1 suppresses mammary tumorigenesis driven by WNT1 activation and following parity. Autophagy 2014; 10:2036-52; PMID:25483966; http://dx.doi.org/10.4161/auto.34398
- Wang L, Yao L, Zheng YZ, Xu Q, Liu XP, Hu X, Wang P, Shao ZM. Expression of autophagy-related proteins ATG5 and FIP200 predicts favorable disease-free survival in patients with breast cancer. Biochem Biophys Res Commun 2015; 458:816-22; PMID:25689718; http://dx.doi.org/10.1016/j.bbrc.2015.02.037
- He Y, Zhao X, Subahan NR, Fan L, Gao J, Chen H. The prognostic value of autophagy-related markers beclin-1 and microtubule-associated protein light chain 3B in cancers: a systematic review and meta-analysis. Tumour Biol 2014; 35:7317-26; PMID:24838948; http://dx.doi.org/10.1007/s13277-014-2060-4
- Ladoire S, Penault-Llorca F, Senovilla L, Dalban C, Enot D, Locher C, Prada N, Poirier-Colame V, Chaba K, Arnould L, et al. Combined evaluation of LC3B puncta and HMGB1 expression predicts residual risk of relapse after adjuvant chemotherapy in breast cancer. Autophagy 2015; 11:1878-90; PMID:26506894; http://dx.doi.org/10.1080/15548627.2015.1082022
- Galluzzi L, Pietrocola F, Levine B, Kroemer G. Metabolic control of autophagy. Cell 2014; 159:1263-76; PMID:25480292; http://dx.doi.org/10.1016/j.cell.2014.11.006
- Levine B, Mizushima N, Virgin HW. Autophagy in immunity and inflammation. Nature 2011; 469:323-35; PMID:21248839; http://dx.doi.org/10.1038/nature09782
- Karantza-Wadsworth V, Patel S, Kravchuk O, Chen G, Mathew R, Jin S, White E. Autophagy mitigates metabolic stress and genome damage in mammary tumorigenesis. Genes Dev 2007; 21:1621-35; PMID:17606641; http://dx.doi.org/10.1101/gad.1565707
- Green DR, Galluzzi L, Kroemer G. Mitochondria and the autophagy-inflammation-cell death axis in organismal aging. Science 2011; 333:1109-12; PMID:21868666; http://dx.doi.org/10.1126/science.1201940
- Elgendy M, Sheridan C, Brumatti G, Martin SJ. Oncogenic Ras-induced expression of Noxa and Beclin-1 promotes autophagic cell death and limits clonogenic survival. Mol Cell 2011; 42:23-35; PMID:21353614; http://dx.doi.org/10.1016/j.molcel.2011.02.009
- Goussetis DJ, Gounaris E, Wu EJ, Vakana E, Sharma B, Bogyo M, Altman JK, Platanias LC. Autophagic degradation of the BCR-ABL oncoprotein and generation of antileukemic responses by arsenic trioxide. Blood 2012; 120:3555-62; PMID:22898604; http://dx.doi.org/10.1182/blood-2012-01-402578
- Vakifahmetoglu-Norberg H, Kim M, Xia HG, Iwanicki MP, Ofengeim D, Coloff JL, Pan L, Ince TA, Kroemer G, Brugge JS, et al. Chaperone-mediated autophagy degrades mutant p53. Genes Dev 2013; 27:1718-30; PMID:23913924; http://dx.doi.org/10.1101/gad.220897.113
- Ma Y, Adjemian S, Mattarollo SR, Yamazaki T, Aymeric L, Yang H, Portela Catani JP, Hannani D, Duret H, Steegh K, et al. Anticancer chemotherapy-induced intratumoral recruitment and differentiation of antigen-presenting cells. Immunity 2013; 38:729-41; PMID:23562161; http://dx.doi.org/10.1016/j.immuni.2013.03.003
- Rao S, Tortola L, Perlot T, Wirnsberger G, Novatchkova M, Nitsch R, Sykacek P, Frank L, Schramek D, Komnenovic V, et al. A dual role for autophagy in a murine model of lung cancer. Nat Commun 2014; 5:3056; PMID:24445999; http://dx.doi.org/10.1038/ncomms4056
- Michaud M, Martins I, Sukkurwala AQ, Adjemian S, Ma Y, Pellegatti P, Shen S, Kepp O, Scoazec M, Mignot G, et al. Autophagy-dependent anticancer immune responses induced by chemotherapeutic agents in mice. Science 2011; 334:1573-7; PMID:22174255; http://dx.doi.org/10.1126/science.1208347
- Michaud M, Xie X, Bravo-San Pedro JM, Zitvogel L, White E, Kroemer G. An autophagy-dependent anticancer immune response determines the efficacy of melanoma chemotherapy. Oncoimmunology 2014; 3:e944047; PMID:25610726; http://dx.doi.org/10.4161/21624011.2014.944047
- Ko A, Kanehisa A, Martins I, Senovilla L, Chargari C, Dugue D, Marino G, Kepp O, Michaud M, Perfettini JL, et al. Autophagy inhibition radiosensitizes in vitro, yet reduces radioresponses in vivo due to deficient immunogenic signalling. Cell Death Differ 2014; 21:92-9; PMID:24037090; http://dx.doi.org/10.1038/cdd.2013.124
- Denkert C, Loibl S, Noske A, Roller M, Muller BM, Komor M, Budczies J, Darb-Esfahani S, Kronenwett R, Hanusch C, et al. Tumor-associated lymphocytes as an independent predictor of response to neoadjuvant chemotherapy in breast cancer. J Clin Oncol 2010; 28:105-13; PMID:19917869; http://dx.doi.org/10.1200/JCO.2009.23.7370
- Loi S, Sirtaine N, Piette F, Salgado R, Viale G, Van Eenoo F, Rouas G, Francis P, Crown JP, Hitre E, et al. Prognostic and predictive value of tumor-infiltrating lymphocytes in a phase III randomized adjuvant breast cancer trial in node-positive breast cancer comparing the addition of docetaxel to doxorubicin with doxorubicin-based chemotherapy: BIG 02-98. J Clin Oncol 2013; 31:860-7; PMID:23341518; http://dx.doi.org/10.1200/JCO.2011.41.0902
- Stoll G, Enot D, Mlecnik B, Galon J, Zitvogel L, Kroemer G. Immune-related gene signatures predict the outcome of neoadjuvant chemotherapy. Oncoimmunology 2014; 3:e27884; PMID:24790795; http://dx.doi.org/10.4161/onci.27884
- Adams S, Gray RJ, Demaria S, Goldstein L, Perez EA, Shulman LN, Martino S, Wang M, Jones VE, Saphner TJ, et al. Prognostic value of tumor-infiltrating lymphocytes in triple-negative breast cancers from two phase III randomized adjuvant breast cancer trials: ECOG 2197 and ECOG 1199. J Clin Oncol 2014; 32:2959-66; PMID:25071121; http://dx.doi.org/10.1200/JCO.2013.55.0491
- Ladoire S, Arnould L, Apetoh L, Coudert B, Martin F, Chauffert B, Fumoleau P, Ghiringhelli F. Pathologic complete response to neoadjuvant chemotherapy of breast carcinoma is associated with the disappearance of tumor-infiltrating foxp3+ regulatory T cells. Clin Cancer Res 2008; 14:2413-20; PMID:18413832; http://dx.doi.org/10.1158/1078-0432.CCR-07-4491
- Ladoire S, Mignot G, Dabakuyo S, Arnould L, Apetoh L, Rebe C, Coudert B, Martin F, Bizollon MH, Vanoli A, et al. In situ immune response after neoadjuvant chemotherapy for breast cancer predicts survival. J Pathol 2011; 224:389-400; PMID:21437909; http://dx.doi.org/10.1002/path.2866
- DeNardo DG, Brennan DJ, Rexhepaj E, Ruffell B, Shiao SL, Madden SF, Gallagher WM, Wadhwani N, Keil SD, Junaid SA, et al. Leukocyte complexity predicts breast cancer survival and functionally regulates response to chemotherapy. Cancer Discov 2011; 1:54-67; PMID:22039576; http://dx.doi.org/10.1158/2159-8274.CD-10-0028
- Ruffell B, Au A, Rugo HS, Esserman LJ, Hwang ES, Coussens LM. Leukocyte composition of human breast cancer. Proc Natl Acad Sci U S A 2012; 109:2796-801; PMID:21825174; http://dx.doi.org/10.1073/pnas.1104303108
- Senovilla L, Vitale I, Martins I, Tailler M, Pailleret C, Michaud M, Galluzzi L, Adjemian S, Kepp O, Niso-Santano M, et al. An immunosurveillance mechanism controls cancer cell ploidy. Science 2012; 337:1678-84; PMID:23019653; http://dx.doi.org/10.1126/science.1224922
- Miyashita M, Sasano H, Tamaki K, Chan M, Hirakawa H, Suzuki A, Tada H, Watanabe G, Nemoto N, Nakagawa S, et al. Tumor-infiltrating CD8+ and FOXP3+ lymphocytes in triple-negative breast cancer: its correlation with pathological complete response to neoadjuvant chemotherapy. Breast Cancer Res Treat 2014; 148:525-34; PMID:25395319; http://dx.doi.org/10.1007/s10549-014-3197-y
- Kroemer G, Senovilla L, Galluzzi L, Andre F, Zitvogel L. Natural and therapy-induced immunosurveillance in breast cancer. Nat Med 2015; In Press; PMID:26444637
- Stoll G, Bindea G, Mlecnik B, Galon J, Zitvogel L, Kroemer G. Meta-analysis of organ-specific differences in the structure of the immune infiltrate in major malignancies. Oncotarget 2015; 6:11894-909; PMID:26059437; http://dx.doi.org/10.18632/oncotarget.4180
- Dieci MV, Criscitiello C, Goubar A, Viale G, Conte P, Guarneri V, Ficarra G, Mathieu MC, Delaloge S, Curigliano G, et al. Prognostic value of tumor-infiltrating lymphocytes on residual disease after primary chemotherapy for triple-negative breast cancer: a retrospective multicenter study. Ann Oncol 2015; 26:1518; PMID:26109735; http://dx.doi.org/10.1093/annonc/mdv239
- Dieci MV, Mathieu MC, Guarneri V, Conte P, Delaloge S, Andre F, Goubar A. Prognostic and predictive value of tumor-infiltrating lymphocytes in two phase III randomized adjuvant breast cancer trials. Ann Oncol 2015; 26:1698-704; PMID:25995301; http://dx.doi.org/10.1093/annonc/mdv239
- Stoll G, Zitvogel L, Kroemer G. Differences in the composition of the immune infiltrate in breast cancer, colorectal carcinoma, melanoma and non-small cell lung cancer: a microarray-based meta-analysis. Oncoimmunology 2015; In Press.
- Krysko DV, Garg AD, Kaczmarek A, Krysko O, Agostinis P, Vandenabeele P. Immunogenic cell death and DAMPs in cancer therapy. Nat Rev Cancer 2012; 12:860-75; PMID:23151605; http://dx.doi.org/10.1038/nrc3380
- Kroemer G, Galluzzi L, Kepp O, Zitvogel L. Immunogenic cell death in cancer therapy. Annu Rev Immunol 2013; 31:51-72; PMID:23157435; http://dx.doi.org/10.1146/annurev-immunol-032712-100008
- Schreiber RD, Old LJ, Smyth MJ. Cancer immunoediting: integrating immunity's roles in cancer suppression and promotion. Science 2011; 331:1565-70; PMID:21436444; http://dx.doi.org/10.1126/science.1203486
- Martins I, Wang Y, Michaud M, Ma Y, Sukkurwala AQ, Shen S, Kepp O, Metivier D, Galluzzi L, Perfettini JL, et al. Molecular mechanisms of ATP secretion during immunogenic cell death. Cell Death Differ 2014; 21:79-91; PMID:23852373; http://dx.doi.org/10.1038/cdd.2013.75
- Rao S, Yang H, Penninger JM, Kroemer G. Autophagy in non-small cell lung carcinogenesis: A positive regulator of antitumor immunosurveillance. Autophagy 2014; 10:529-31; PMID:24413089; http://dx.doi.org/10.4161/auto.27643
- Apetoh L, Ghiringhelli F, Tesniere A, Obeid M, Ortiz C, Criollo A, Mignot G, Maiuri MC, Ullrich E, Saulnier P, et al. Toll-like receptor 4-dependent contribution of the immune system to anticancer chemotherapy and radiotherapy. Nat Med 2007; 13:1050-9; PMID:17704786; http://dx.doi.org/10.1038/nm1622
- Yamazaki T, Hannani D, Poirier-Colame V, Ladoire S, Locher C, Sistigu A, Prada N, Adjemian S, Catani JP, Freudenberg M, et al. Defective immunogenic cell death of HMGB1-deficient tumors: compensatory therapy with TLR4 agonists. Cell Death Differ 2014; 21:69-78; PMID:23811849; http://dx.doi.org/10.1038/cdd.2013.72
- Vacchelli E, Ma Y, Baracco EE, Sistigu A, Enot DP, Pietrocola F, Yang H, Adjemian S, Chaba K, Semeraro M, et al. Chemotherapy-induced antitumor immunity requires formyl peptide receptor 1. Science 2015; 350:972-8; PMID:26516201; http://dx.doi.org/10.1126/science.aad0779
- Ladoire S, Chaba K, Martins I, Sukkurwala AQ, Adjemian S, Michaud M, Poirier-Colame V, Andreiuolo F, Galluzzi L, White E, et al. Immunohistochemical detection of cytoplasmic LC3 puncta in human cancer specimens. Autophagy 2012; 8:1175-84; PMID:22647537; http://dx.doi.org/10.4161/auto.20353
- Hothorn T, Lausen B. On the exact distribution of maximally selected rank statistics. Computational Statistics & Data Analysis 2003; 43:121-37; http://dx.doi.org/10.1016/S0167-9473(02)00225-6