ABSTRACT
Leishmania is an obligate intracellular parasite that replicates inside phagolysosomes or parasitophorous vacuoles (PV) of the monocyte/macrophage lineage. It reprograms macrophages and produces a metabolic state conducive to successful infection and multiplication. MicroRNAs (miRNAs), a class of small 22 to 24 nucleotide noncoding regulatory RNAs alter the gene expression and consequently proteome output by targeting mRNAs, may play a regulatory role in modulating host cell functions. In the present study, we demonstrate the novel regulatory role of host microRNA, MIR30A-3p in modulation of host cell macroautophagy/autophagy after infection with L. donovani. Our in vitro studies showed that MIR30A-3p expression was significantly enhanced after L. donovani infection in a time-dependent manner. Transient transfection with a MIR30A-3p inhibitor followed by L. donovani infection promoted the autophagic response and decreased the intracellular parasite burden in both THP-1 cells and human monocyte-derived macrophages (HsMDM). BECN1/Beclin 1, the mammalian ortholog of yeast Vps30/Atg6, is a key autophagy-promoting protein that plays a key role in the regulation of cell death and survival. We report BECN1-dependent modulation of host cell autophagy in response to L. donovani infection. Pretreatment of L. donovani-infected macrophages with the MIR30A-3p mimic decreased and with antagomir increased the expression of BECN1 protein. We demonstrate that BECN1 is a potential target of MIR30A-3p and this miRNA negatively regulates BECN1 expression. Our present study reveals for the first time a novel role of MIR30A-3p in regulating autophagy-mediated L. donovani elimination by targeting BECN1. The present study has significant impact for the treatment of visceral leishmaniasis.
Introduction
Leishmania is an obligate intracellular parasite and is known to employ a range of subversion mechanisms inside macrophages to subvert and/or suppress leishmanicidal activities of macrophages. These subversion strategies include repression of macrophage-microbicidal functions, such as inhibition of nitric oxide (NO) production, rewiring of host cell signaling pathways, and inhibition of cytokine-inducible macrophage function and modulation of metabolic pathways.Citation1-3
Host cells are also known to initiate autophagy as a central innate defense mechanism.Citation4,5 Autophagy is an evolutionarily conserved cellular adaptive response against intra- or extracellular stress and signals such as starvation-induced nutrient deprivation, ER-stress and pathogenic insult.Citation6 Autophagy restricts bacterial and viral pathogens such as Group A Streptococcus,Citation7 Salmonella,Citation8 mycobacteria,Citation9 Listeria,Citation10 RickettsiaCitation11 and viruses including herpes simplex virusCitation12 and human cytomegalovirus.Citation13 Conversely, intracellular pathogens such as Toxoplasma exploit the autophagy apparatus for their survival and nutrition acquisition.Citation14 Earlier reports suggest acquisition of host cell macromolecules by L. mexicana involves an autophagy-like mechanism.Citation15 Induction of host cell autophagy mediated by either starvation or cytokines alters the intracellular burden of Leishmania depending upon the strain of mice used in the study.Citation16,17 Depending on the cellular context, autophagy can either increase nutrient supply to starved cells via recycling of products or can act like a central antimicrobial defense complementing the innate immunity by restricting the growth and proliferation of invading pathogens.Citation18,19
The formation of autophagosomes is mediated by a series of autophagy-related gene products such as multiple autophagy-related (ATG) proteins, several of which are associated into protein complexes controlling different stages of autophagy, including initiation and elongation of the phagophore, the precursor to the autophagosome.Citation18 ATG7 (autophagy-related 7) is an E1-activating enzyme and has a role in membrane elongation, whereas MAP1LC3B (microtubule-associated protein 1 light chain 3β) is located on both sides of the autophagosome and that part of the population localized to the concave surface is degraded during autolysosomal maturation.Citation5 BECN1/Vps30/Atg6 is a BH3-only domain protein and a key autophagy promoter protein, needed for localization of several autophagic proteins to a phagophore assembly site (PAS).Citation20,21
Recent evidence suggests that several microRNAs (miRNAs) may participate in modulating autophagy by directly targeting autophagy-related genes, such as BECN1 and ATG4.Citation22,23 MicroRNAs, a class of small noncoding RNAs, are key regulators of gene expression at the post-transcriptional level. They target mRNA leading to translational repression or degradation.Citation24 MicroRNA-mediated gene regulation is a fundamental layer of the genetic program involved in the development of diseases and inflammation.Citation25 Modulation of host miRNAs is yet another feature involved during infection with intracellular pathogens. Profiling of host miRNAs during the course of infection with bacterial, viral or parasitic pathogens have indicated their role in innate defenses as well as pathogen-mediated exploitation of these noncoding RNAs in distortion of innate immunity and regulation of cell survival mechanisms.Citation26-29
Human macrophages infected with L. major showed a significant change in the expression pattern of a large number of miRNAs, suggesting their potential role in modulating the gene expression profile of the infected cells. Several miRNAs that were modulated in human macrophages after infection with L. major or L. donovani have been reported to be induced during inflammation and activation of toll-like receptors mediated by either pathogenic challenge or by bacterial lipopolysaccharides.Citation30,31
In the present study, we identified key miRNAs that were differentially modulated after L. donovani infection. First we report key information regarding the molecular mechanism involved in the autophagy response in L. donovani-infected host cells. Subsequently, we discuss our investigation of the potential role of the MIR30 family member, MIR30A-3p in modulating autophagy and L. donovani infection. We, for the first time, demonstrate the regulatory role of MIR30A-3p in autophagy-mediated L. donovani elimination by targeting BECN1. Our findings demonstrate understanding of the host defense mechanisms after L. donovani infection.
Results
Profiling of miRNA expression in THP-1 cells infected with L. donovani
In order to investigate the modulation pattern of host miRNAs after Leishmania infection, THP-1 cells were infected with stationary phase promastigotes of L. donovani for 45 min, 6 h and 24 h. RNA samples were extracted from Leishmania-infected THP-1 cells postinfection. RNA derived from uninfected THP-1 cells (0 h) served as a negative control. The percentage of infected cells and parasite load at all time points was examined via microscopy, and infection was consistent across various time points. The infection rate was ∼45% to 60%, ∼60% to 70%, and ∼65% to 80% at 45 min, 6 h and 24 h, respectively, and number of parasites per infected cell were ∼4.5, ∼6.0 and ∼7.5 parasites/macrophage at 45 min, 6 h and 24 h, respectively.
Using human miRNA 8 × 15 k arrays, a distinct miRNA expression profile was observed across different time points after infection with reference to 0 h in THP-1 cells after Leishmania infection. We found several key members of microRNA families that were differentially modulated across different time points in THP-1 cell post-Leishmania infection. These families included: let-7, MIR30, MIR17, MIR15 and the MIR9 family of miRNAs, clearly suggesting the specific role of these miRNAs during Leishmania infection and proliferation in a time-dependent manner. shows the heat map of 38 infection-specific differentially expressed miRNA grouped in a response-specific manner.
Figure 1. Heat map and hierarchical clustering of infection-specific miRNAs. Heat map and hierarchical clustering of 38 infection-specific differentially expressed key miRNAs in THP-1 cells in response to Leishmania infection at 45 min, 6 h and 24 h as compared to uninfected control. The heat-map shows several members of key families of miRNAs (let-7, MIR30, MIR17, MIR15 and MIR9) differentially modulated across different time points. The miRNA expression values are presented using a red (upregulated), black (median value) and green (downregulated) color scheme.
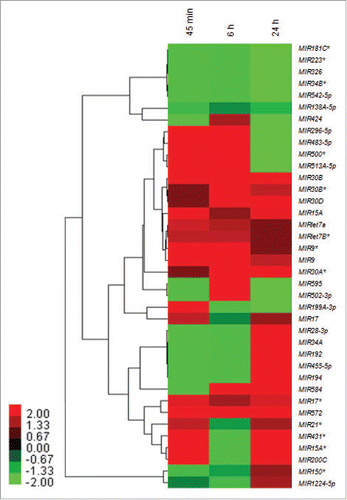
Expression of the MIR30 family of miRNAs is significantly upregulated in vitro after L. donovani infection
The MIR30 subfamily of miRNAs includes several paralogs such as MIR30A, B, D and E located at different genomic positions.Citation23 A considerable representation of several members of this family that were consistently upregulated across different time points was observed in our microarray-based expression analysis. To validate the changes in the expression level of key members of the MIR30 family of miRNAs, we examined L. donovani-induced induction in THP-1 and HsMDMs cells. A qRT-PCR based approach was adopted to validate the expression profile of key members of MIR30 family members. shows a time-dependent increase in the expression of MIR30B, MIR30D and MIR30A-3p in both THP-1 cells and HsMDMs after infection with L. donovani. Maximum expression of MIR30B, MIR30D and MIR30A-3p was observed at 6 h in THP-1 cells (MIR30B, ∼6.0 fold; P < 0.05; MIR30D, ∼4.5-fold; P < 0.05 and MIR30A-3p, ∼15.8-fold; P < 0.01) post-Leishmania infection followed by a significant decline in the expression at 36 and 48 h (). The expression pattern of MIR30B, MIR30D and MIR30A-3p in HsMDMs infected with Leishmania showed an increase in expression of MIR30B (∼3.7-fold; P < 0.01), MIR30D (∼2.1-fold; P < 0.05) and MIR30A-3p (∼9.9-fold; P < 0.01) at 6 h followed by a time-dependent decrease at 36 and 48 h (). MIR30A-3p showed increased abundance in both THP-1 cells (∼11.7-fold; P < 0.05) and HsMDMs (∼7.83 fold; P < 0.05) at 24 h after Leishmania infection (), indicating a possible regulatory role played by this noncoding RNA in human macrophages infected with L. donovani. While the real-time qRT-PCR studies validated the findings of the miRNA microarray experiments, they also suggest a critical association of MIR30 family members in the reprogramming of macrophage function during the course of infection.
Figure 2. Induction of the Leishmania-specific MIR30 family of miRNAs. The MIR30 family of miRNAs is induced in macrophages infected with L. donovani. Expression levels of different miRNAs were examined in THP-1 cells infected with L. donovani with a multiplicity of infection (MOI) of 10 at different time-points (A) MIR30B, (B) MIR30D and (C) MIR30A-3p. The expression levels were also examined in human peripheral blood monocyte-derived macrophages (HsMDMs) infected with L. donovani with an MOI of 10 at different time-points (D) MIR30B, (E) MIR30D and (F) MIR30A-3p. Expression of let-7a (G) and let-7b* (H) in THP-1 cells infected with L. donovani. The graphical data points represent mean of 3 replicate experiments ± standard error mean (SEM). (Mann-Whitney test; **, P < 0.001; *, P < 0.05). U44 Small RNA (RNU44A) was used as an internal control. Data analysis was performed using 2-ΔΔCt method.
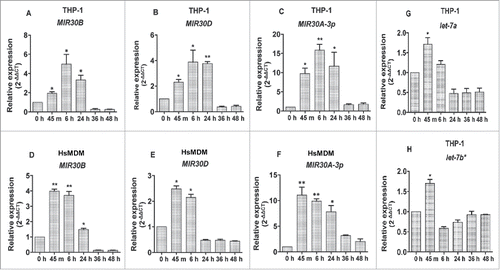
A considerable representation of several members of the let-7 family of miRNAs was observed in the miRNA microarray expression profile in an infection-specific manner across different time-points (). Host miRNA profiling in human macrophages and dendritic cells infected with either L. donovani or L. major have earlier suggested a role of let-7 family members during the course of infection. While the let-7 family of miRNAs (let-7a and 7b) was reported to be upregulated in macrophages infected with L. donovani, these miRNAs were found repressed in response to L. major infection.Citation31 Our miRNA expression profile suggested an early induction of let-7a and let-7b* in THP-1 cells infected with L. donovani (). A very similar expression pattern was observed for let-7a and let-7b* in the qRT-PCR based validation studies, where they were upregulated at 45 min (let-7a, ∼1.71fold; P < 0.05 and let-7b*, ∼1.7fold; P < 0.05) after Leishmania infection followed by a gradual decline in expression level till 48 h post-Leishmania infection ().
We then conducted miRWalk (www.ma.uniheidelberg.de/apps/zmf/mirwalk/)-based target identification for MIR30 family members. This led to identification of several gene targets such as BECN1, ATG3 and ATG9 that play a critical role in the induction of autophagy (). Earlier reports indicate induction of autophagy in human and murine macrophages infected with different Leishmania species.Citation17,32,33 The regulatory role of MIR30 family members in downregulation of several genes (e.g., BECN1) involved in autophagy process in cancer cells and infection models has been reported earlier.Citation34-36
Table 1. Pattern of the let-7 and MIR30 families of microRNAs that were differentially expressed in THP-1 cells after L. donovani infection and the key experimentally validated gene targets as identified by the miRNA target-prediction tool, miRWalk.
Effect of MIR30A-3p on autophagic activity in THP-1 cells infected with L. donovani
To further investigate the regulatory role of MIR30 family members in modulation of autophagy in Leishmania-infected macrophages, we first examined the status of autophagy in THP-1 cells infected with L. donovani. This experiment was critical to further investigate the regulatory role of MIR30 family members in modulation of autophagy in Leishmania-infected macrophages.
Autophagy is characterized by the redistribution of MAP1LC3B (microtubule-associated protein 1 light chain 3β) proteins into cytoplasmic puncta, coinciding with LC3 lipidation (to generate LC3-II). Immunostaining for LC3-II is one of the ways to ascertain the status of autophagosome formation in cells undergoing stress and conditions known to induce autophagy.Citation37 The status of autophagy was investigated in L. donovani-infected THP-1 cells using confocal microscopy and LC3-II specific immunostaining across different time points postinfection.
Immunostaining of THP-1 cells infected with L. donovani for 24, 36 and 48 h using anti-LC3B antibody suggested an initial increase in the expression of LC3-II at 24 and 36 h followed by a decline at 48 h (). The maximum autophagy response was observed at 36 h after Leishmania infection. Rapamycin, an antibiotic that induces autophagy in a BECN1-dependent manner was selected as a positive control.Citation6 Treatment of THP-1 cells with rapamycin (100 nM) for 24 h resulted in increased autophagy as measured by the number of LC3-II-positive puncta in the treated cells (). Western blot analysis of LC3 processing (conversion of LC3-I to LC3-II) from lysates derived from Leishmania-infected THP-1 cells further confirmed the dynamic pattern of autophagy in host cells infected with Leishmania (). The increased level of LC3-II was observed at 12, 24 and 36 h in Leishmania-infected THP-1 cells followed by a decline at 48 h (). Maximum conversion of endogenous LC3-I to LC3-II was observed at 24 and 36 h. The difference between levels of LC3-II at 24 and 36 h was not statistically significant (). THP-1 cells treated with rapamycin (100 nM for 24 h) were taken as positive controls to confirm autophagic activation (). Suppression of autophagosome formation appears to be a delayed response in infected macrophages.
Figure 3. Induction of autophagy in THP-1 cells in response to Leishmania infection. (A) THP-1 cells were infected with promastigotes of L. donovani for 24, 36 and 48 h. Cells were subsequently fixed and immunostaining was carried out using anti-LC3B antibody (green) to detect the autophagosomes. DRAQ5 staining (red) was performed to detect cell nuclei and infected Leishmania parasites. Uninfected THP-1 cells were used as control cells to compare the status of autophagy in Leishmania-infected cells. Rapamycin (100 nM) was used as an inducer of autophagy in THP-1 cells. Merged images show colocalization of parasites and LC3-II foci (yellow). The zoomed confocal photomicrographs show localization of LC3-II puncta around Leishmania parasites in macrophages infected with parasites. Confocal microscopy was performed to acquire images. Scale bars: 10 μm for 63x images. Experiments were repeated 3 times, the images shown are representative of a single experiment. (B) Bar diagram showing number of LC3-II puncta per macrophage. To access the number of puncta per cell, approximately 100 cells were counted for the number of puncta for each condition. (Student t test; ***, P < 0.001; ** , P < 0.01; * , P < 0.05). (C) THP-1 cells were infected with L. donovani for 12, 24, 36 and 48 h. Protein levels of LC3 were detected by western blot in the cell lysates using anti-LC3B antibody. Cell lysates derived from rapamycin (100 nM) treated cells were used as positive control. Densitometric analysis shows the fold change in expression of LC3-II (autophagy marker) in THP-1 cells infected with L. donovani. TUBB was used as the loading control. The data are representative of 3 independent experiments. (Unpaired t test with the Welch correction; *, P < 0.05). Rapa, rapamycin.
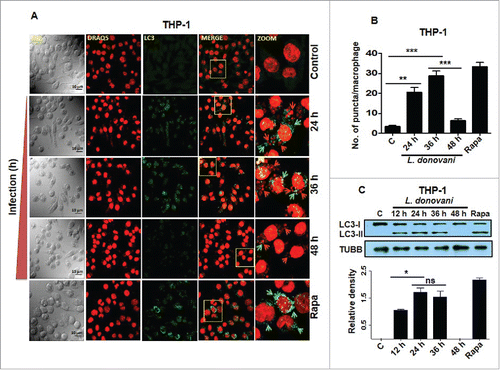
Next, we tested the effect of MIR30A-3p on the autophagic response to L. donovani infection. We evaluated the autophagic activity in THP-1 cells and HsMDM using western blots to test the processing of LC3 (conversion from LC3-I to LC3-II). Western blot results showed that transient transfection with the MIR30A-3p mimic did not result in decrease in activation of autophagy in both L. donovani-infected THP-1 cells and HsMDM (lane 4) when compared to the infected cells (lane 2) as suggested by no significant change in the amount of LC3-II (). A further reduction in LC3-II expression through the MIR30A-3p mimic should ideally result in additional inhibition, but conceivably the inhibitory effect of infection alone was already so much that additional presence of the MIR30A-3p mimic does not result in significantly visible additional inhibition. Transfection with a MIR30A-3p inhibitor showed an increased amount of LC3-II in THP-1 cells and HsMDMs after Leishmania infection (P < 0.01) () (lane 6) in comparison to the L. donovani-infected cells (lane 2).
Figure 4. Effect of MIR30A-3p expression on autophagy in Leishmania-infected THP-1 cells and HsMDMs. (A) THP-1 cells and HsMDMs were transfected with the MIR30A-3p mimic (lane 3) and the MIR30A-3p inhibitor (lane 5) for 36 h. Cells transfected with the MIR30A-3p mimic (lane 4) and the MIR30A-3p inhibitor (lane 6) were further infected with L. donovani for 24 h. Cell lysates from uninfected control cells (lane 1) and macrophages infected with L. donovani (lane 2) were used as controls to compare the LC3-II expression, as a marker of autophagy. Protein levels of LC3 were detected by western blot in the cell lysates using anti-LC3B antibody. (B) Densitometric analysis shows the fold change in expression of LC3-II (autophagy marker) in both THP-1 and HsMDM infected with L. donovani. TUBB was used as the loading control. Western blot images are representative of 3 independent experiments. (Unpaired t test with the Welch correction; ***, P < 0.0001; **, P < 0.001; *, P < 0.05); LD: Leishmania donovani; Infect., Infection; HsMDM, human monocyte-derived macrophages.
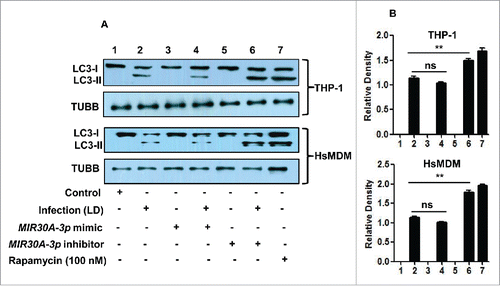
MIR30A-3p influences intracellular burden of THP-1 cells infected with Leishmania
Further investigations were carried out to determine the biological outcome of MIR30A-3p induction in THP-1 cells and HsMDMs on the burden of intra-macrophagial amastigotes. We analyzed parasite burden in THP-1 cells and HsMDMs either infected with Leishmania alone or previously transfected with the MIR30A-3p mimic or the MIR30A-3p inhibitor followed by Leishmania infection. The intracellular parasite burden was determined at 48 h post-Leishmania infection. Transfection with the MIR30A-3p mimic followed by Leishmania infection resulted in slight but significantly higher parasite burden in THP-1 cells (P = 0.0329) () when compared to the untransfected cells infected with Leishmania. However, transfection of HsMDMs with the MIR30A-3p mimic followed by Leishmania infection showed an essentially similar parasite burden as untransfected cells infected with Leishmania (). It is possible that infection with L. donovani alone has already reached maximum levels and the additional presence of the MIR30A-3p mimic does not result in significantly visible additional increase of MIR30A-3p. The MIR30A-3p inhibitor-transfected cells had a relatively lower number of parasites per macrophages in both THP-1 cells and HsMDMs ().
Figure 5. Expression of MIR30A-3p influences intracellular burden of Leishmania parasites in THP-1 cells. (A) THP-1 differentiated macrophages were transfected with the MIR30A-3p mimic or the MIR30A-3p inhibitor for 48 h followed by infection with promastigotes of L. donovani (MOI of 10). Cells were fixed and subsequently stained with fluorescent nucleic acid probe, DRAQ5. Confocal microscopy was performed to assess the parasite burden. The image is a representative field among multiple random fields analyzed for scoring average parasite number per macrophage from a minimum of 100 infected cells counted from at least 10 fields. Arrows indicate infected parasites, scale bars: 10 μm for 63x images. Bar diagram showing average number of parasites per THP-1 cells (B). To assess the parasite burden, at least 100 macrophages were counted for the number of parasites in each condition of manipulation. The statistical significance was determined by using the Student t test (***, P < 0.001; **, P < 0.01; *, P < 0.05). LD, Leishmania donovani.
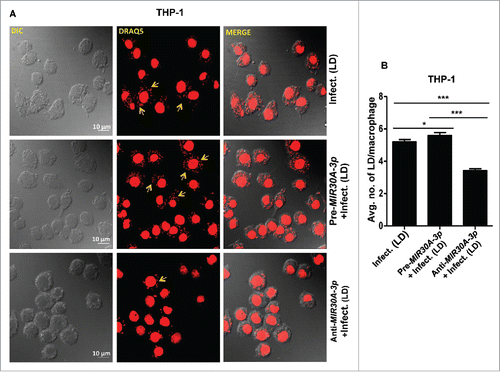
Figure 6. Expression of MIR30A-3p influences intracellular burden of Leishmania parasites in HsMDMs. (A) HsMDMs were transfected with the MIR30A-3p mimic or the MIR30A-3p inhibitor for 48 h followed by infection with promastigotes of L. donovani (MOI of 10). Cells were fixed and subsequently stained with the fluorescent nucleic acid probe, DRAQ5. Confocal microscopy was performed to assess the parasite burden. The image is a representative field among multiple random fields analyzed for scoring average parasite number per macrophage from a minimum of 100 infected cells counted from at least 10 fields. Arrows indicate infected parasites, scale bars: 10 μm for 63x images. Bar diagram showing average number of parasites per cell (B). To assess the parasite burden, at least 100 macrophages were counted for the number of parasites in each condition of manipulation. The statistical significance was determined by using the Student t test (***, P < 0.001; **, P < 0.01; *, P < 0.05). DIC, differential interference contrast; HsMDM, human monocyte-derived macrophages; LD, Leishmania donovani.
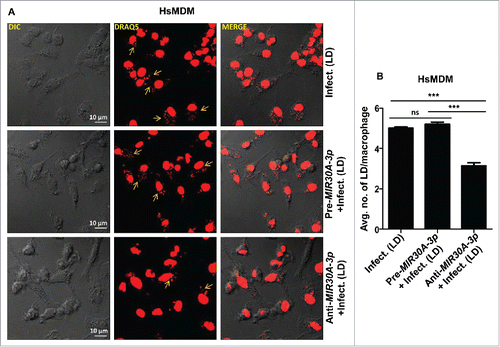
MIR30A-3p negatively regulates BECN1 expression in Leishmania-infected THP-1 cells and HsMDMs
The formation of autophagosomes is mediated by a series of autophagy-related gene products. These proteins function at different stages of autophagy.Citation18 Autophagy-related genes, such as BECN1 and ATG3, are critical to nucleation and elongation of phagophores.Citation38 In order to demonstrate the molecular mechanism involved in the autophagic response in L. donovani-infected macrophages, we determined the expression pattern of BECN1 and ATG3 in infected macrophages. The kinetics of expression of these 2 key genes was followed at the level of mRNA as well as protein in both THP-1 and HsMDM cells infected with Leishmania.
We determined the modulation of expression pattern of BECN1 and ATG3 in THP-1 and HsMDM cells infected with L. donovani across different time points. A qRT-PCR based approach was employed to assess the level of BECN1 and ATG3 in Leishmania-infected macrophages. The pattern of expression of BECN1 and ATG3 was followed till 48 h in both THP-1 cells and HsMDMs infected with L. donovani. Induction of both BECN1 and ATG3 was observed at 45 min (BECN1, ∼29.7-fold, P < 0.001; ATG3, ∼32.2-fold, P < 0.001) and 6 h (BECN1, ∼43.4-fold, P < 0.001; ATG3, ∼40.6-fold, P < 0.001) in Leishmania-infected THP-1 cells followed by decline till 48 h (). These results were further validated in primary human macrophages (HsMDMs). A similar expression profile of BECN1 and ATG3 was observed (). These findings suggest a dynamic modulation of BECN1 and ATG3 in macrophages after Leishmania infection, suggesting an early induction of gene expression followed by a progressive and strong repression at 24, 36 and 48 h after Leishmania infection. Our results indicate downregulation of BECN1 and ATG3 in Leishmania-infected macrophages is a delayed response.
Figure 7. Expression pattern of BECN1 and ATG3 in macrophages infected with L. donovani across different time points. Bar diagrams showing fold expression of (A) BECN1 and (B) ATG3 in Leishmania-infected THP-1 cells and HsMDMs (C and D) as compared to uninfected control. The expression pattern was accessed by quantitative real-time qRT-PCR. The qRT-PCR data shown represents mean values obtained from 3 independent experiments. U6 small nuclear 1 RNA (RNU6-1) was used as the endogenous control. Data analysis was performed using 2-ΔΔCt method. (Mann-Whitney test; ***, P < 0.001; **, P < 0.01; *, P < 0.05). Western blot showing time-dependent expression of BECN1 and ATG3 in THP-1 (E) cells and HsMDMs (F) infected with Leishmania donovani. TUBB was used as the loading control. Western blot images are representative of 3 independent experiments.
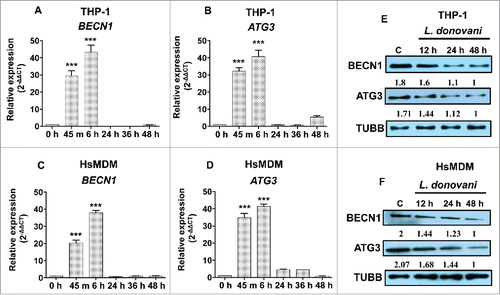
Immunoblotting experiments were also performed using cell lysates derived from both THP-1 cells and HsMDMs infected with L. donovani for 12, 24 and 48 h. Western blot analysis using cell lysates derived from THP-1 cells infected with parasites suggested lower levels of BECN1 and ATG3 at 48 h postinfection (). These results were further confirmed in HsMDMs infected with L. donovani for different time periods (). A time-dependent decrease in the level of BECN1 and ATG3 was observed in HsMDMs post-Leishmania infection.
To investigate the expression pattern of BECN1, THP-1 cells were infected with L. donovani for 24, 36 and 48 h followed by immunostaining using anti-BECN1 antibody. Confocal microscopy suggested a time-dependent increase in the expression pattern of BECN1 in Leishmania-infected THP-1 cells, with maximum expression at 36 h postinfection, followed by a progressive decline at 48 h (). Together, these studies suggest a potential regulatory mechanism possibly operating at the post-transcriptional level in Leishmania-infected macrophages leading to significant reduction in the level of gene-transcripts of BECN1 and ATG3 in infected macrophages. The immunostaining and western blot studies using both THP-1 cells and primary macrophages (HsMDMs) infected with L. donovani suggest downregulation of BECN1 and ATG3 as a delayed response against Leishmania infection. A perfect temporal correlation between BECN1 expression and modulation of autophagy in THP-1 cells infected with Leishmania suggests BECN1-dependent modulation of host cell autophagy in response to L. donovani infection.
Figure 8. BECN1 expression pattern in macrophages infected with Leishmania. Confocal photomicrographs showing BECN1 expression and infected parasites in THP-1 cells infected with L. donovani. THP-1 cells were infected with promastigotes of Leishmania and were allowed to proliferate for 24, 36 and 48 h postinfection. Uninfected cells were used as controls to compare the level of BECN1 across different times. Cells were subsequently fixed and immunostaining was carried out using anti-BECN1 antibody (green). A fluorescent nucleic acid probe, DRAQ5 (red), was used to stain cell nuclei of macrophages and parasites. Scale bars: 10 μm for 63x images. Confocal microscopy was performed to acquire images. Experiments were repeated 3 times; the images shown are representatives of a single experiment.
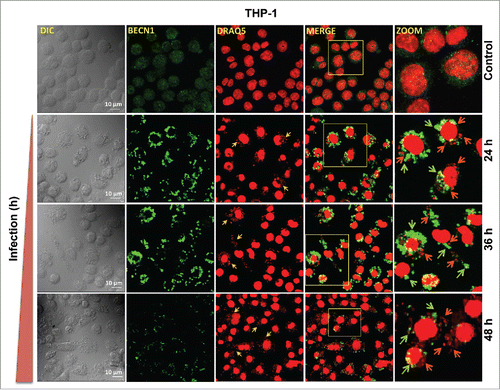
To determine the functional consequences of modulation of BECN1 expression by MIR30A-3p, we then went ahead to establish the relationship between induction of MIR30A-3p and downregulation of BECN1 and autophagy in both THP-1 and HsMDMs cells post- L. donovani infection.
Several independent reports have suggested the role of MIR30A as a potential regulator of BECN1 expression at the level of both gene transcript as well as protein in cancer cells and macrophages challenged with intracellular pathogens.Citation34,36 Earlier reports also indicate the regulatory role of MIR30A in autophagy modulation, as overexpression of MIR30A resulted into BECN1-mediated repression of autophagy.Citation34 To obtain experimental evidence to support BECN1 as a target for MIR30A-3p, we further examined the effect of MIR30A-3p in regulating BECN1 and ATG3 levels in macrophages. Both THP-1 cells and HsMDMs were transfected with the MIR30A-3p mimic and the expression of BECN1 and ATG3 was observed at the level of mRNA and proteins using qRT-PCR and western blot analyses.
As shown in (), transfection of the MIR30A-3p mimic into macrophages led to an approximately 80% reduction in the level of BECN1 in both THP-1 cells () and HsMDMs (). There was more than a 50% reduction at the mRNA level of ATG3 in both THP-1 cells () and HsMDMs (). These results clearly demonstrate that both BECN1 and ATG3 are specific targets of MIR30A-3p in both THP-1 cells and primary human macrophages (HsMDMs).
Figure 9. MIR30A-3p specifically targets BECN1 and ATG3. Downregulation of BECN1 and ATG3 in THP-1 cells (A and B) and HsMDM (D and E) transfected with the MIR30A-3p mimic. Macrophages were transfected with the MIR30A-3p mimic (25 nM), RNA was isolated (48 h post-transfection) and quantitative real-time RT-PCR was performed to access the expression status of BECN1 and ATG3. The control mimic for MIR30A-3p was used as a negative control. qRT-PCR data shown here represent mean values obtained from 3 independent experiments. RNU6-1 was used as the endogenous control. Data analysis was performed using 2-ΔΔCt method. (Mann-Whitney test; *, P < 0.05). Downregulation of BECN1 and ATG3 in THP-1 cells (C) and HsMDM (F) transfected with the MIR30A-3p mimic. Macrophages were transfected with the MIR30A-3p mimic (25 nM) and the corresponding control mimic for 48 h. Cell lysates from control THP-1 cells and cells transfected with the miR-mimic for MIR30A-3p, control mimic and cells treated with transfection reagent were probed for BECN1 and ATG3 expression using corresponding primary antibodies. TUBB was used the loading control. The data are representative of 3 independent experiments. HsMDM, human monocyte-derived macrophages. Cell cont, cell control; Transf. cont, transfection control.
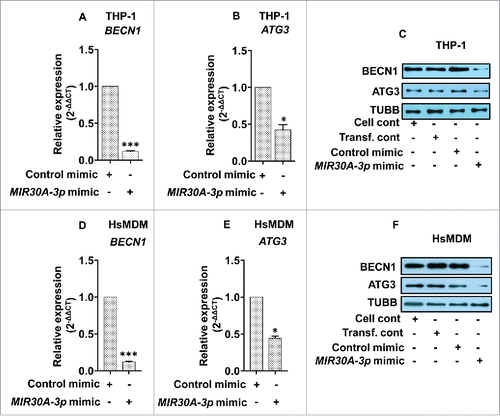
Additionally, immunoblotting experiments were carried out to see the effect of the MIR30A-3p mimic on the protein expression of BECN1 and ATG3 using cell lysates derived from THP-1 cells and HsMDMs previously transfected with either the MIR30A-3p mimic or its negative control mimic. A significant repression of BECN1 and ATG3 was observed in both THP-1 cells () as well as HsMDMs (). These experiments clearly demonstrate a suppressive role of this miRNA on expression of autophagy-related BECN1 and ATG3.
BECN1 repression mediated by MIR30A-3p is a Leishmania-specific response
To further validate the role of MIR30A-3p in regulating the expression of BECN1, both THP-1 cells and HsMDMs were transfected with either the MIR30A-3p mimic or the MIR30A-3p inhibitor followed by infection with L. donovani. Uninfected cells transfected with either the MIR30A-3p mimic or the MIR30A-3p inhibitor were used as corresponding controls. Since the previous results suggested a significant reduction in the level of BECN1 in macrophages post-Leishmania infection, the same window time-point (48 h) was selected to ascertain the level of BECN1 after transfecting the cells with either the miR-mimic or the inhibitor of MIR30A-3p followed by Leishmania infection.
Western blot analyses using cell lysates derived from THP-1 cells () and HsMDMs () were performed to confirm the Leishmania-infection-specific regulation of expression of BECN1. Cell lysates derived from uninfected (lane 1), Leishmania-infected (lane 2), MIR30A-3p mimic-transfected (lane 3), MIR30A-3p inhibitor-transfected (lane 4), MIR30A-3p mimic-transfected followed by Leishmania infection (lane 5) and MIR30A-3p inhibitor-transfected followed by Leishmania infection (lane 6) was used and immunoblotting was performed using anti-BECN1 antibody. A reduced level of BECN1 in miR-mimic-transfected THP-1 cells or HsMDMs (lane 3) () was observed when compared to the levels in uninfected THP-1 cells or HsMDMs. Cells transfected with the MIR30A-3p mimic when infected with L. donovani resulted in decreased expression of BECN1 (lane 5) () when compared to the MIR30A-3p mimic-transfected cells, suggesting infection specific upregulation of MIR30A-3p and subsequent targeting of BECN1 (). MIR30A-3p inhibitor-transfected cells showed an enhanced level of BECN1 (lane 4) () that was comparable to that of the uninfected control (). However, MIR30A-3p inhibitor-transfected cells when infected with Leishmania showed an increased expression of BECN1 (lane 6) when compared to pre-miR-transfected cells infected with Leishmania (lane 5) in both THP-1 cells and HsMDMs (). Densitometry analysis of western blots clearly suggests significant downregulation of BECN1 expression in macrophages transfected with the MIR30A-3p mimic in both THP-1 cells () and HsMDMs (). The combined additive effect of the MIR30A-3p mimic together with Leishmania infection resulted in further repression of BECN1 in macrophages ().
Figure 10. Leishmania-specific downregulation of BECN1 is mediated by MIR30A-3p. Effect of MIR30A-3p on BECN1 expression in THP-1 cells (A) and human peripheral blood monocyte derived macrophages (B) infected with L. donovani. Cells were transfected with the MIR30A-3p mimic (lane 3) and the MIR30A-3p inhibitor (lane 4) for 48 h. Cells transfected with the MIR30A-3p mimic (lane 5) and the MIR30A-3p inhibitor (lane 6) for 48 h were further infected with L. donovani for 36 h. Cell lysates from uninfected cells (lane 1) and cells infected with L. donovani for 36 h (lane 2) were used as controls to compare the expression status of BECN1. Protein levels of BECN1 were detected by western blot in the cell lysates using anti-BECN1 antibody. TUBB was used as the loading control. The data are representative of 3 independent experiments. Bar diagram showing fold-changes in protein expression using densitometric analysis of the corresponding western blot of THP-1 cells (C) and HsMDM (D). (Unpaired t test with the Welch correction; ***, P < 0.001; **, P < 0.01; *, P < 0.05). cont., control; LD, L. donovani; HsMDM, human monocyte-derived macrophages.
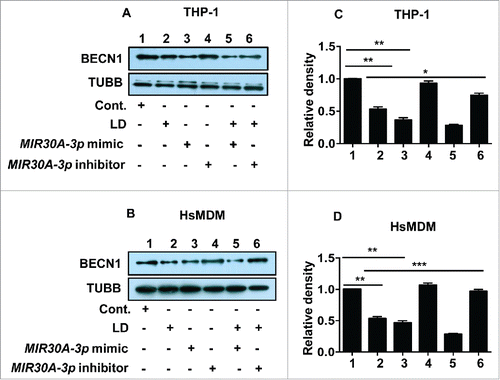
Discussion
Induction of autophagy in Leishmania-infected macrophages has been shown to play a key role in providing essential nutrients to the multiplying parasites inside PV and/or also selectively targeting parasites to be neutralized by the innate defense response. However, the molecular mechanism involved in the autophagy response in macrophages infected with Leishmania remains largely unclear. Understanding the regulation of infection-induced autophagy by noncoding RNAs, and in particular by miRNAs, is still not established. The physiological importance of the host miRNA-autophagy interconnection is only beginning to be decoded during the course of bacterial infection.Citation36,39,40 However, it represents a new understanding of the post-transcriptional regulatory control of autophagy by miRNAs. The exact mechanistic details of host microRNAs in regulating the autophagy process during the course of Leishmania infection remains unknown. The present study firstly, provides key information regarding the molecular mechanism involved in the autophagy response in L. donovani-infected host cells. Secondly, this study also reveals a novel role of MIR30A-3p as a key post-transcriptional regulator that targets BECN1, the key molecule of autophagic process in Leishmania-infected macrophages.
Autophagy-related (ATG) genes are the chief autophagy regulators controlling different stages of the autophagy process, including initiation and elongation steps during autophagosome formation.Citation38 In the past several years, accumulating evidence suggests post-transcriptional controls mediated by noncoding miRNAs significantly influence the process of autophagy in cancer, and most recently during infection with viruses and intracellular pathogens.Citation22,39-41 The miRNA-based modulation of autophagy process is achieved at the level of cell cycle control, genotoxic stress and hypoxia among others.Citation42 BECN1 is a central molecule that lies at the crossroads of the process of autophagy and apoptosis depending on its differential interaction with key proteins that regulate the process of cell survival inside the cell.Citation21 BECN1, the mammalian ortholog of yeast Vps30/Atg6, is needed for localization of several autophagic proteins to a phagophore assembly site.Citation21,38 The expression level of BECN1 during several pathophysiological processes is a direct consequence of transcriptional as well as post-transcriptional regulation. BECN1 expression is governed by transcription factors (E2F and NFKB) as well as the MIR30 family of miRNAs.Citation34,43 MIR30A also plays a regulatory role in the autophagic response by targeting BECN1 expression in cancer cells and macrophages infected with Mycobacterium tuberculosis.Citation34,36
Host microRNA profiling of human monocyte-derived dendritic cells and macrophages infected with different species of Leishmania followed by target analyses have yielded enormous information leading to identification of several key miRNAs that target MAP kinases and signaling cascades modulated during the course of Leishmania infection.Citation30,31 A recent report suggests induction of MIR210 in a HIF1A-dependent manner in human macrophages infected with L. major.Citation30 Another report identifies a significant reduction in the level of MIR122 expression that is correlated with the altered expression of lipid metabolic genes in the liver of a murine model of visceral leishmaniasis.Citation44 These findings, strongly suggest involvement of microRNAs as key regulators of disease phenotype in Leishmania-infected host cells.
The present study identifies the regulatory role of the MIR30 family member MIR30A-3p as the key modulator of host cell autophagy after infection with L. donovani. We first examined the molecular mechanism involved in the autophagy response in L. donovani-infected host cells. A perfect temporal correlation between BECN1 expression and modulation of autophagy in THP-1 cells infected with L. donovani suggests an early induction of BECN1 and autophagosome formation and downregulation of BECN1 and suppression of autophagy is a delayed response.
In vitro experiments showed that MIR30A-3p expression was significantly enhanced after L. donovani infection in a time-dependent manner. Transient transfection with the MIR30A-3p inhibitor followed by L. donovani infection promoted the autophagic response and decreased the intracellular parasite burden in both THP-1 cells and HsMDMs.
Previous studies have shown that MIR30A could negatively regulate BECN1 gene expression, resulting in decreased autophagic activity. An early induction of the MIR30 family of miRNAs () in Leishmania-infected macrophages suggested possible involvement of these miRNAs in repression of host autophagy. To investigate the biological outcome of infection-specific MIR30A-3p induction, we monitored the expression level of its cellular target (BECN1) and finally autophagy in infected macrophages. It appears that the basal level of BECN1 protein already present in the cells was sufficient to initiate autophagosomal nucleation and support autophagy induction during infection. Both MIR30A-3p and BECN1 transcription were induced immediately after infection (during a window of 45 min to 6 h) (). However, as time progressed following infection, MIR30A-3p-dependent degradation of BECN1 transcripts () prevented any de novo synthesis of BECN1 protein explaining the gradual decrease in the levels of BECN1 with time due to steady protein turnover (). Furthermore, the loss of translatable BECN1 transcripts, due to the action of MIR30A-3p might not result in an immediate penury of functional BECN1 protein owing to its fairly stable nature. The existing pool of BECN1 protein therefore may be sufficient for induction and sustenance of autophagy at 24 and 36 h. This MIR30A-3p-dependent regulation of BECN1 was further reflected on autophagy in macrophages infected with Leishmania ().
Our results clearly demonstrated that BECN1 is a potential target of MIR30A-3p and this miRNA negatively regulates BECN1 expression resulting in decreased autophagic activity. Treatment of macrophages with the MIR30A-3p mimic decreased, and with an antagomiR increased, the expression of the BECN1 protein. Our results further confirm that BECN1 is a putative target of MIR30A-3p in both THP-1 cells and HsMDMs infected with L. donovani. This regulation appears to be a delayed response during the course of infection ().
Several reports indicate that host autophagy could be a pathogen-friendly response and at times the induction of autophagy may be pathogen-mediated. Pathogen-induced autophagy in host cells could be an important determinant of nutrient supply to the intracellular pathogen whose survival is greatly dependent on host resources. Autophagy can also act like a central antimicrobial defense complementing the innate immunity by restricting the growth and proliferation of invading pathogens.Citation18,19 Several landmark studies establish host-cell autophagy as an important innate immune response against intracellular bacterial pathogens (such as M. tuberculosis and Shigella spp.) and extracellular bacterial pathogens that invade the cytosol (such as group A Streptococcus). These observations are context dependent, where different model systems have been used for different intra-macrophagial pathogens.
Acquisition of host cell macromolecules by L. mexicana involves an autophagy-like mechanism.Citation15 Cytokines have been reported to alter the intracellular burden of Leishmania depending upon the strain of mice used in the study.Citation17 Autophagy may favor an inflammatory or immune-silencing response depending on which antigens are presented. As a result T-cell polarization to Th1 and Th2 may change. This polarization plays an important role in Leishmania disease outcome.Citation45 A recent study demonstrates apoptotic-like parasites activate the macrophages' autophagy machinery, thereby dampening T-cell responses and enhancing parasite survival.Citation45 In the present study we found upregulation of host cell autophagy in response to Leishmania infection that appears to be a host protective response at 24 and 36 h. MIR30A-3p-dependent downregulation of autophagy at 48 h, ensured survival of intracellular Leishmania. Furthermore, anti-miR-mediated intervention resulted in significantly reduced parasite burden possibly by restoring the host-cell autophagic machinery.
The miRNA based modulation of the autophagy process is achieved at the level of cell-cycle control, genotoxic stress and hypoxia among others.Citation46-48 A newer paradigm in cancer treatment involves anti-autophagy cancer therapy that carries the potential to exploit the strong relationship between microRNAs active during oncogenesis and their potential link with autophagy regulation.Citation42,48 Human MIR101, MIR30A, and MIR221 are some of the key miRNAs that have proved their therapeutic potential against cancer.Citation35,46,47 These therapeutic approaches involve usage of miR-mimics or anti-miRs as potential therapeutic molecules for anticancer drug development.Citation49
The role of miRNAs in controlling intracellular pathogens such as Mycobacterium and Salmonella is well documented and several microRNAs including MIR30A and MIR155 play key roles in regulating autophagy process by targeting several key molecules regulating the process of autophagy during the course of infection in human macrophages.Citation36,39 As the information accumulates, there is an urgent need to look into the regulatory mechanisms controlling the host cell autophagy during pathogenic challenge. This information further carries the merit to identify potential antipathogenic therapeutics and decipher the basic information related to the infection process.
Materials and methods
Leishmania strain and culture conditions
Leishmania donovani strain AG83 (MHOM/IN/1983/AG83) was maintained in BALB/c mice by repeated passage. BALB/c mice for maintenance of the parasite were obtained from the National Institute of Nutrition (Hyderabad, India) and were maintained in the institute facility. Amastigotes were isolated from the spleen of infected BALB/c mice and were transformed into promastigotes, prior to infection as reported earlier.Citation50 Modified M199 media (Sigma-Aldrich, M5017) supplemented with 10% heat-inactivated fetal bovine serum (Gibco®/BRL, Life Technologies, 10082147) and 0.13 mg/ml penicillin and streptomycin were used to culture Leishmania promastigotes at 26°C.
Cell line, macrophage differentiation and infection
THP-1 cells, an acute monocytic leukemia-derived human cell line (ATCC, TIB-202TM) were maintained in RPMI-1640 (Sigma-Aldrich, R8005) medium supplemented with 10% heat inactivated fetal bovine serum and 1% streptomycin/penicillin at 37°C and 5% CO2. Cells were treated with 50 ng/ml phorbol 12-myristate 13-acetate (Sigma-Aldrich, P1585) for 48 h to induce differentiation into macrophage-like cells before infection.
Primary human peripheral blood mononuclear cells were isolated using a Ficoll Paque density gradient (Sigma-Aldrich, GE-17-5442-02) on whole blood collected from healthy donors. Human monocyte-derived macrophages (HsMDMs) were isolated as described previously.Citation50 Briefly, 2 × 106 monocytes were incubated in the presence of recombinant human macrophage colony-stimulating factor (M-CSF) (300 ng/ml) (Sigma-Aldrich, G5618) in RPMI medium with 10% fetal calf serum. Nonadherent cells were periodically removed followed by addition of fresh media containing recombinant human CSF1/M-CSF (300 ng/ml; Sigma-Aldrich, G5618) and 10% human serum (Sigma-Aldrich, H3667) every 12 h. Cells were allowed to differentiate into HsMDMs for 96 h.
For RNA and protein isolation, both macrophage-like THP-1 cells and HsMDMs were seeded at a density of 0.5 × 106 cells per ml. For microscopy studies, 0.2 × 106 cells were seeded on sterile glass cover slips placed in individual wells in 6-well plate. To carry out in vitro infection assays, late stationary phase promastigotes were used at a ratio of 10 parasites per macrophage inside culture wells. Leishmania-infected macrophages were incubated for different time periods to allow the establishment of infection and proliferation of intra-macrophagial parasites.
miRNA microarray profiling
RNA extraction was carried out using TRI reagent (Sigma-Aldrich, T9424) as per the manufacturer's instruction. RNA labeling and hybridization of miRNA microarray was carried out using an miRNA Complete Labeling and Hyb kit (Agilent Technologies, 5190-0456). The hybridization experiments were carried out at 55°C for 20 h using human miRNA 8×15k arrays (Agilent Technologies, AMADID: 21827). The microarray scanning was performed on a G2505C scanner (Agilent Technologies, G2565CA). The microarray hybridization experiments were carried out at Genotypic Technology Pvt. Ltd, Bangalore, India. Image analysis was done using Agilent Feature Extraction software Version 10.7 (Agilent Technologies). Feature extracted data were analyzed using Agilent GeneSpring GX version 12 software (Agilent Technologies). Normalization of the data was done in GeneSpring GX using the 90th percentile shift and normalization to specific samples.Citation27 Significant differentially expressed miRNA up- and downregulated within the group of samples were identified. Differentially expressed miRNAs were selected to have a 1.5 and above fold expression difference between THP-1 cells infected with L. donovani in comparison to uninfected cells. An unsupervised hierarchical clustering of infection specific differentially expressed miRNAs was carried out by performing a Pearson Uncentered algorithm with an average linkage rule to identify miRNAs patterns that were specific to different time points of infection. For miRNA target identification differentially modulated miRNAs were queried in miRWalk comprehensive database (www.ma.uniheidelberg.de/apps/zmf/mirwalk/) (last updated on March 29, 2011) to generate information on experimentally validated gene targets.Citation51
miRNA transfection
Macrophage-like THP-1 cells and HsMDMs in exponential phase of growth were placed in 60 mm plates at a density of 1 × 106 and were cultured overnight and then transfected with a mimic or antagomiR of MIR30A-3p or a nontargeting control RNA (25 nM). Synthetic pre-miRNA (Ambion, AM17100), anti-miR (Ambion, AM17000), negative control pre-miRNA (Ambion, AM17110) and negative control anti-miR Inhibitor (Ambion, AM17010) for MIR30A-3p were purchased from Ambion, USA. The oligos were diluted in nuclease-free water supplied with the kit as suggested by the manufacturer. N-TER™ Nanoparticle siRNA Transfection System (Sigma-Aldrich, N2913) was used to transfect both macrophage-like THP-1 cells and HsMDMs as reported earlier.Citation52
Determination of transfection efficiency
Cells were transfected with a control fluorophore conjugated siRNA using N-TER peptide based transfection kit (Sigma-Aldrich, N2913). After 6 h of incubation, cells were thoroughly washed with phosphate-buffered saline (PBS; Gibco, 10010072) and observed under a fluorescence microscope (Eclipse TE2000, Nikon, Japan). Transfection efficiency was calculated in terms of percentage of cells expressing fluorescence, and was calculated by observing a minimum of 20 fields under 40x. Transfection experiments with a minimum of 60% efficiency were considered for further processing.
TaqMan miRNA assay for miRNA array validation
Validation of miRNA expression was carried out using quantitative real-time RT-PCR (qRT-PCR) analysis by TaqMan miRNA assays (Life Technologies, 4427975). Briefly, miRNAs were converted to cDNA using TaqMan® miRNA Reverse Transcription kit (Life Technologies, 4366596). This was followed by quantitative real-time RT-PCR using the miRNA specific primers in a total reaction volume of 10 μL of reverse transcription product, 5 μL of 10x TaqMan Universal PCR master mix (Life Technologies, 4364341), 0.5 μL nuclease-free water, and 0.5 μL of the 20x primer probe mix (TaqMan Small RNA Assay) provided with TaqMan MicroRNA Assay Kit. SNORD44 (small nucleolar RNA, C/D box 44; Life Technologies, 4427975) amplification was used as the internal control in the assay. The thermal profile for the PCR was 95°C for 10 min followed by 40 cycles at 95°C for 15 sec and final extension of 60°C for 60 sec. ABI Prism 7000 sequence detection system instrument (Applied Biosystems, Thermo Fisher Scientific, MA, USA) was used to perform the experiment. The experiments were performed in triplicate and the results were expressed using 2-ΔΔCt method.Citation53
qRT-PCR for gene expression analysis
mRNA quantification, amplification and quantification of cDNA was performed using SYBR Green-based qRT-PCR as discussed previously.Citation50 Briefly, cDNA was prepared using first-strand cDNA synthesis kit (Thermo Fisher Scientific, k1612). Real-time PCR (RT-PCR) with gene-specific primers was performed on the resulting cDNA using Fast SYBR® green double-stranded DNA binding dye (Applied Biosystems, 4385612) and ABI Prism 7000 sequence detection system instrument (Applied Biosystems). The details of the primers (NCBI gene identifiers, sequences and product sizes) are given in the Table S1. The thermal profile for the real-time PCR was amplification at 50°C for 2 min, followed by 40 cycles at 95°C for 30 s, 62°C for 1 min, and 72°C for 20 s. To determine the primer efficiency, a 10-fold dilution series experiment was carried out with the cDNA to obtain the average slope of each primer pair. Melting curve analysis confirmed generation of a specific PCR product. Amplification of RNU6-1 (RNA, U6 small nuclear 1) was used as an internal control. The experiments were performed in triplicate and the results were expressed using the 2-ΔΔCt method.Citation53
Confocal microscopy
Briefly, cells were washed repeatedly using ice-cold PBS and were subsequently fixed using 4% paraformaldehyde (PFA) for 15 min, followed by cell permeabilization using 0.1% Triton X-100 (Sigma-Aldrich, T8787) for 10 min at room temperature (RT). Cell blocking was performed using 1% BSA (Sigma-Aldrich, A2153) for 30 min. Cells were washed 3 times with 1× PBS and subsequently incubated overnight with primary antibody against BECN1 (1:1000; BD Biosciences, 612113) at 4°C. Cells were washed and incubated with Alexa Fluor 488-conjugated secondary antibody (Invitrogen, A11001) (Excitation/Emission wavelength: 488/525 nm) at RT for 45 min. DNA staining (for both macrophage and parasite nuclei) was performed using DNA fluorescent probe DRAQ5 (5 μM) (Excitation/Emission wavelength: 633/681 nm) (Thermo Fisher Scienific, 62251) for 5 min. Images were captured at 63× magnification Leica TCS SP5 II (Leica Microsystems, Wetzlar, Germany) confocal microscope. Image analysis was carried out using LAS AF integrated Leica software.
For quantification of LC3-II puncta, cells were fixed using 4% PFA and were subsequently incubated overnight with the primary antibody (LC3B/MAP1LC3B ; 1:1000) (Novus Biologicals, NB100-2220) at 4°C. Cells were washed and were incubated with Alexa Fluor 488-conjugated secondary antibody (Invitrogen, A11008) at RT for 45 min. DNA staining (for both macrophage and parasite nuclei) was performed using DNA fluorescent probe DRAQ5 (5 μM) (Thermo Fisher Scientific, 62251) for 5 min. Images were captured at 63x magnification using a Leica TCS SP5 II (Leica Microsystems, Wetzlar, Germany) confocal microscope and were processed for algorithmic quantification of LC3-II puncta using custom-written ImageJ macro-containing plug-ins as described previously.Citation52 At least 100 cells per condition were counted.
Determination of intracellular parasite burden
We used an easy to perform and highly sensitive DRAQ5 staining to locate the macrophage nuclei as well as parasitic DNA to determine number of infected parasites as mentioned elsewhere.Citation54 Briefly, cells were fixed using 4% PFA, washed and were subsequently incubated with DNA fluorescent probe DRAQ5 (5 μM) (Thermo Fisher Scientific, 62251) for 5 min at RT. Cells were washed with 1 × PBS to remove excess dye and images were acquired at 63x using a Leica TCS SP5 II (Leica Microsystems, Wetzlar, Germany) confocal microscope. At least 100 cells were counted manually for each condition to determine the average number of parasites per macrophage (Fig. S1 A and B).
As an additional confirmation for the presence of parasites in macrophages, real-time PCR was done as reported earlier.Citation55 The amplification target was on the kinetoplast minicircle DNA of the parasite. The details of the primers (NCBI gene identifiers, sequences and product sizes) are given in Table S1. Briefly, 100 ng of total DNA isolated from uninfected and infected macrophages was used as template in real-time minicircle PCR with 4 replicates to determine the mean Ct value. The Ct value for the uninfected macrophage sample was found to be the highest, indicating the absence of any parasite DNA in macrophages. Conversely, the Ct value for the infected macrophages decreased indicating, amplification of parasite specific kinetoplast minicircle DNA (Fig. S1C and D).
Western blotting
Cells were lysed using cell lysis buffer (0.125 mol/L Tris, pH 6.8, 4% SDS [Sigma-Aldrich, L3771], 20% glycerol (Sigma-Aldrich, G5516), 10% 2-mercaptoethanol [Sigma-Aldrich, M625]. CBX protein assay kit (G-biosciences, 786-12X) was used to determine the protein concentration per the manufacturer's protocol. Cell lysate (30 to 60 µg) was electrophoresed in 12% SDS polyacrylamide gels and transferred onto nitrocellulose membranes at RT. Membrane blocking was performed in 5% fat free milk (Santa Cruz Biotechnology, sc-2325) in 0.05% Tween 20 (Sigma-Aldrich, P9416) in 1x PBS. The membranes were incubated with primary antibodies for BECN1 (1:1000; BD Biosceinces, 612113), ATG3 (1:1000; Cell Signaling Technology, 3415P), LC3B/MAP1LC3B (1:1000; Novus Biologicals, NB100-2220) and TUBB/β tubulin (1:4000; Thermo, RB-9249-P1) in PBS-Tween 20 having 1% BSA and incubated overnight at 4°C. The blots were subsequently incubated with the secondary antibody conjugated to horseradish peroxidase (Abcam, ab6721)at 1:3000 dilutions in 0.05% PBS-Tween 20 for 1 h at RT. Protein bands were observed on X-ray film using femto LUCENT™ PLUS-HRP Chemiluminiscent reagent kit (G-biosciences, 786-003).
Statistical analyses
Fold-expression (qRT-PCR and densitometric analyses), quantification of LC3-II puncta and intracellular parasite burden were represented as mean value ± standard error mean (SEM). Data distribution was analyzed for normality using D'Agostino and Pearson omnibus normality test. Differences between individual test groups were analyzed by applying an unpaired Student t test. In cases where a test for normality was not feasible, the data were either normalized for variances, and further analyzed by applying an unpaired t test with the Welch correction, or the Mann-Whitney test was performed to compensate for non-Gaussian distribution. All statistics was performed using GraphPad Prism Version 5.01. P values < 0.05 were considered statistically significant.
Ethics statement
Experimental protocols involving HsMDMs were reviewed and experiments were performed following approval by the Institutional Human Ethical Committee of the National Institute of Immunology (Project no. IHEC #l84/14). The protocol for maintaining BALB/c mice used in the present study, were approved by the Institutional Animal Ethics Committee (IAEC) of Jawaharlal Nehru University (JNU) (IAEC Code Number: 24/2011).
Abbreviations
HsMDMs | = | human monocyte-derived macrophages |
LD | = | Leishmania donovani |
MAP1LC3B/LC3B | = | microtubule-associated protein 1 light chain 3 β |
miRNAs | = | microRNAs |
MOI | = | multiplicity of infection |
PBS | = | phosphate-buffered saline |
PFA | = | paraformaldehyde |
PV | = | parasitophorous vacuole |
qRT-PCR | = | quantitative reverse transcriptase-polymerase chain reaction |
Rapa | = | rapamycin |
SDS-PAGE | = | sodium dodecyl sulfate-polyacrylamide gel electrophoresis |
VL | = | visceral leishmaniasis |
Disclosure of potential conflicts of interest
No potential conflicts of interest were disclosed.
Supplementary files
Download Zip (182 KB)Acknowledgments
We acknowledge the technical support provided by Mr. Madavan Vasudevan, Bionivid Technology Private Limited, Bangalore, India during microarray data analyses.
Funding
Rentala Madhubala is a JC Bose National Fellow. The funding was from the Department of Science and Technology, Ministry of Science and Technology (DST), India (D.O. No. SB/SO/HS/009/2013) to RMB. Alok Singh is supported by a fellowship from Council of Scientific and Industrial Research, India. DST-PURSE grant supported the publication charges.
References
- Olivier M, Gregory DJ, Forget G. Subversion mechanisms by which Leishmania parasites can escape the host immune response: a signaling point of view. Clin Microbiol Rev 2005; 18:293-305; PMID:15831826; http://dx.doi.org/10.1128/CMR.18.2.293-305.2005
- Shadab M, Ali N. Evasion of Host Defence by Leishmania donovani: Subversion of Signaling Pathways. Mol Biol Int 2011; 2011:343961; PMID:22091401; http://dx.doi.org/10.4061/2011/343961
- Naderer T, McConville MJ. The Leishmania-macrophage interaction: a metabolic perspective. Cell Microbiol 2008; 10:301-8; PMID:18070117; http://dx.doi.org/10.1111/j.1462-5822.2007.01096.x
- Deretic V, Saitoh T, Akira S. Autophagy in infection, inflammation and immunity. Nat Rev Immunol 2013; 13:722-37; PMID:24064518; http://dx.doi.org/10.1038/nri3532
- Levine B, Mizushima N, Virgin HW. Autophagy in immunity and inflammation. Nature 2011; 469:323-35; PMID:21248839; http://dx.doi.org/10.1038/nature09782
- Ravikumar B, Sarkar S, Davies JE, Futter M, Garcia-Arencibia M, Green-Thompson ZW, Jimenez-Sanchez M, Korolchuk VI, Lichtenberg M, Luo S, et al. Regulation of mammalian autophagy in physiology and pathophysiology. Physiol Rev 2010; 90:1383-435; PMID:20959619; http://dx.doi.org/10.1152/physrev.00030.2009
- Nakagawa I, Amano A, Mizushima N, Yamamoto A, Yamaguchi H, Kamimoto T, Nara A, Funao J, Nakata M, Tsuda K, et al. Autophagy defends cells against invading group A Streptococcus. Science 2004; 306:1037-40; PMID:15528445; http://dx.doi.org/10.1126/science.1103966
- Birmingham CL, Smith AC, Bakowski MA, Yoshimori T, Brumell JH. Autophagy controls Salmonella infection in response to damage to the Salmonella-containing vacuole. J Biol Chem 2006; 281:11374-83; PMID:16495224; http://dx.doi.org/10.1074/jbc.M509157200
- Gutierrez MG, Master SS, Singh SB, Taylor GA, Colombo MI, Deretic V. Autophagy is a defense mechanism inhibiting BCG and Mycobacterium tuberculosis survival in infected macrophages. Cell 2004; 119:753-66; PMID:15607973; http://dx.doi.org/10.1016/j.cell.2004.11.038
- Py BF, Lipinski MM, Yuan J. Autophagy limits Listeria monocytogenes intracellular growth in the early phase of primary infection. Autophagy 2007; 3:117-25; PMID:17204850; http://dx.doi.org/10.4161/auto.3618
- Rikihisa Y. Glycogen autophagosomes in polymorphonuclear leukocytes induced by rickettsiae. Anat Rec 1984; 208:319-27; PMID:6721227; http://dx.doi.org/10.1002/ar.1092080302
- Tallóczy Z, Virgin HW, Levine B. PKR-dependent autophagic degradation of herpes simplex virus type 1. Autophagy 2006; 2:24-9; PMID:Can't; http://dx.doi.org/10.4161/auto.2176
- Smith JD, de Harven E. Herpes simplex virus and human cytomegalovirus replication in WI-38 cells. III. Cytochemical localization of lysosomal enzymes in infected cells. J Virol 1978; 26:102-9; PMID:206717
- Wang Y, Weiss LM, Orlofsky A. Host cell autophagy is induced by Toxoplasma gondii and contributes to parasite growth. J Biol Chem 2009; 284:1694-701; PMID:19028680; http://dx.doi.org/10.1074/jbc.M807890200
- Schaible UE, Schlesinger PH, Steinberg TH, Mangel WF, Kobayashi T, Russell DG. Parasitophorous vacuoles of Leishmania mexicana acquire macromolecules from the host cell cytosol via two independent routes. J Cell Sci 1999; 112(Pt 5):681-93; PMID:9973603
- Jaramillo M, Gomez MA, Larsson O, Shio MT, Topisirovic I, Contreras I, Luxenburg R, Rosenfeld A, Colina R, McMaster RW, et al. Leishmania repression of host translation through mTOR cleavage is required for parasite survival and infection. Cell Host Microbe 2011; 9:331-41; PMID:21501832; http://dx.doi.org/10.1016/j.chom.2011.03.008
- Pinheiro RO, Nunes MP, Pinheiro CS, D'Avila H, Bozza PT, Takiya CM, Côrte-Real S, Freire-de-Lima CG, DosReis GA. Induction of autophagy correlates with increased parasite load of Leishmania amazonensis in BALB/c but not C57BL/6 macrophages. Microbes Infect Inst Pasteur 2009; 11:181-90; PMID:19070676; http://dx.doi.org/10.1016/j.micinf.2008.11.006
- Kroemer G, Mariño G, Levine B. Autophagy and the integrated stress response. Mol Cell 2010; 40:280-93; PMID:20965422; http://dx.doi.org/10.1016/j.molcel.2010.09.023
- Orvedahl A, Levine B. Eating the enemy within: autophagy in infectious diseases. Cell Death Differ 2009; 16:57-69; PMID:18772897; http://dx.doi.org/10.1038/cdd.2008.130
- Sinha S, Levine B. The autophagy effector Beclin 1: a novel BH3-only protein. Oncogene 2008; 27(Suppl 1):S137-48; PMID:19641499; http://dx.doi.org/10.1038/onc.2009.51
- Kang R, Zeh HJ, Lotze MT, Tang D. The Beclin 1 network regulates autophagy and apoptosis. Cell Death Differ 2011; 18:571-80; PMID:21311563; http://dx.doi.org/10.1038/cdd.2010.191
- Su Z, Yang Z, Xu Y, Chen Y, Yu Q. MicroRNAs in apoptosis, autophagy and necroptosis. Oncotarget 2015; 6:8474-90; PMID:25893379; http://dx.doi.org/10.18632/oncotarget.3523
- Xu J, Wang Y, Tan X, Jing H. MicroRNAs in autophagy and their emerging roles in crosstalk with apoptosis. Autophagy 2012; 8:873-82; PMID:22441107; http://dx.doi.org/10.4161/auto.19629
- He L, Hannon GJ. MicroRNAs: small RNAs with a big role in gene regulation. Nat Rev Genet 2004; 5:522-31; PMID:15211354; http://dx.doi.org/10.1038/nrg1379
- O'Connell RM, Rao DS, Baltimore D. microRNA regulation of inflammatory responses. Annu Rev Immunol 2012; 30:295-312; PMID:Can't; http://dx.doi.org/10.1146/annurev-immunol-020711-075013
- Schulte LN, Eulalio A, Mollenkopf H-J, Reinhardt R, Vogel J. Analysis of the host microRNA response to Salmonella uncovers the control of major cytokines by the let-7 family. EMBO J 2011; 30:1977-89; PMID:21468030; http://dx.doi.org/10.1038/emboj.2011.94
- Shwetha S, Gouthamchandra K, Chandra M, Ravishankar B, Khaja MN, Das S. Circulating miRNA profile in HCV infected serum: novel insight into pathogenesis. Sci Rep 2013; 3:1555; PMID:23549102; http://dx.doi.org/10.1038/srep01555
- Chen X-M, Splinter PL, O'Hara SP, LaRusso NF. A cellular micro-RNA, let-7i, regulates Toll-like receptor 4 expression and contributes to cholangiocyte immune responses against Cryptosporidium parvum infection. J Biol Chem 2007; 282:28929-38; PMID:17660297; http://dx.doi.org/10.1074/jbc.M702633200
- Cai Y, Chen H, Mo X, Tang Y, Xu X, Zhang A, Lun Z, Lu F, Wang Y, Shen J. Toxoplasma gondii inhibits apoptosis via a novel STAT3-miR-17-92-Bim pathway in macrophages. Cell Signal 2014; 26:1204-12; PMID:24583285; http://dx.doi.org/10.1016/j.cellsig.2014.02.013
- Lemaire J, Mkannez G, Guerfali FZ, Gustin C, Attia H, Sghaier RM, Sysco-Consortium, Dellagi K, Laouini D, Renard P. MicroRNA expression profile in human macrophages in response to Leishmania major infection. PLoS Negl Trop Dis 2013; 7:e2478; PMID:24098824; http://dx.doi.org/10.1371/journal.pntd.0002478
- Geraci NS, Tan JC, McDowell MA. Characterization of microRNA expression profiles in Leishmania-infected human phagocytes. Parasite Immunol 2015; 37:43-51; PMID:25376316; http://dx.doi.org/10.1111/pim.12156
- Mitroulis I, Kourtzelis I, Papadopoulos VP, Mimidis K, Speletas M, Ritis K. In vivo induction of the autophagic machinery in human bone marrow cells during Leishmania donovani complex infection. Parasitol Int 2009; 58:475-7; PMID:19591960; http://dx.doi.org/10.1016/j.parint.2009.07.002
- Cyrino LT, Araújo AP, Joazeiro PP, Vicente CP, Giorgio S. In vivo and in vitro Leishmania amazonensis infection induces autophagy in macrophages. Tissue Cell 2012; 44:401-8; PMID:22939777; http://dx.doi.org/10.1016/j.tice.2012.08.003
- Zhu H, Wu H, Liu X, Li B, Chen Y, Ren X, Liu C-G, Yang J-M. Regulation of autophagy by a beclin 1-targeted microRNA, miR-30a, in cancer cells. Autophagy 2009; 5:816-23; PMID:19535919; http://dx.doi.org/10.4161/auto.9064
- Zou Z, Wu L, Ding H, Wang Y, Zhang Y, Chen X, Chen X, Zhang C-Y, Zhang Q, Zen K. MicroRNA-30a sensitizes tumor cells to cis-platinum via suppressing beclin 1-mediated autophagy. J Biol Chem 2012; 287:4148-56; PMID:22157765; http://dx.doi.org/10.1074/jbc.M111.307405
- Chen Z, Wang T, Liu Z, Zhang G, Wang J, Feng S, Liang J. Inhibition of Autophagy by MiR-30A Induced by Mycobacteria tuberculosis as a Possible Mechanism of Immune Escape in Human Macrophages. Jpn J Infect Dis 2015; 68:420-4; PMID:25866116; http://dx.doi.org/10.7883/yoken.JJID.2014.466
- Mizushima N, Yoshimori T, Levine B. Methods in mammalian autophagy research. Cell 2010; 140:313-26; PMID:20144757; http://dx.doi.org/10.1016/j.cell.2010.01.028
- Xie Z, Klionsky DJ. Autophagosome formation: core machinery and adaptations. Nat Cell Biol 2007; 9:1102-9; PMID:17909521; http://dx.doi.org/10.1038/ncb1007-1102
- Wang J, Yang K, Zhou L, Minhaowu null, Wu Y, Zhu M, Lai X, Chen T, Feng L, Li M, et al. MicroRNA-155 promotes autophagy to eliminate intracellular mycobacteria by targeting Rheb. PLoS Pathog 2013; 9:e1003697; PMID:24130493; http://dx.doi.org/10.1371/journal.ppat.1003697
- Kim JK, Yuk J-M, Kim SY, Kim TS, Jin HS, Yang C-S, Jo E-K. MicroRNA-125a inhibits autophagy activation and antimicrobial responses during mycobacterial infection. J Immunol Baltim Md 1950 2015; 194:5355-65; PMID:25917095
- Cramer EM, Shao Y, Wang Y, Yuan Y. miR-190 is upregulated in Epstein-Barr Virus type I latency and modulates cellular mRNAs involved in cell survival and viral reactivation. Virology 2014; 464-465:184-95; PMID:25086243
- Zhai H, Fesler A, Ju J. MicroRNA: a third dimension in autophagy. Cell Cycle Georget Tex 2013; 12:246-50; PMID:23255136; http://dx.doi.org/10.4161/cc.23273
- Wang B, Ling S, Lin W-C. 14-3-3Tau regulates Beclin 1 and is required for autophagy. PloS One 2010; 5:e10409; PMID:20454448; http://dx.doi.org/10.1371/journal.pone.0010409
- Ghosh J, Bose M, Roy S, Bhattacharyya SN. Leishmania donovani targets Dicer1 to downregulate miR-122, lower serum cholesterol, and facilitate murine liver infection. Cell Host Microbe 2013; 13:277-88; PMID:23498953; http://dx.doi.org/10.1016/j.chom.2013.02.005
- Crauwels P, Bohn R, Thomas M, Gottwalt S, Jäckel F, Krämer S, Bank E, Tenzer S, Walther P, Bastian M, et al. Apoptotic-like Leishmania exploit the host's autophagy machinery to reduce T-cell-mediated parasite elimination. Autophagy 2015; 11:285-97; PMID:25801301; http://dx.doi.org/10.1080/15548627.2014.998904
- Miller TE, Ghoshal K, Ramaswamy B, Roy S, Datta J, Shapiro CL, Jacob S, Majumder S. MicroRNA-221/222 confers tamoxifen resistance in breast cancer by targeting p27Kip1. J Biol Chem 2008; 283:29897-903; PMID:18708351; http://dx.doi.org/10.1074/jbc.M804612200
- Frankel LB, Wen J, Lees M, Høyer-Hansen M, Farkas T, Krogh A, Jäättelä M, Lund AH. microRNA-101 is a potent inhibitor of autophagy. EMBO J 2011; 30:4628-41; PMID:21915098; http://dx.doi.org/10.1038/emboj.2011.331
- Jing Z, Han W, Sui X, Xie J, Pan H. Interaction of autophagy with microRNAs and their potential therapeutic implications in human cancers. Cancer Lett 2015; 356:332-8; PMID:25304373; http://dx.doi.org/10.1016/j.canlet.2014.09.039
- Garofalo M, Croce CM. microRNAs: Master regulators as potential therapeutics in cancer. Annu Rev Pharmacol Toxicol 2011; 51:25-43; PMID:20809797; http://dx.doi.org/10.1146/annurev-pharmtox-010510-100517
- Singh AK, Pandey RK, Siqueira-Neto JL, Kwon Y-J, Freitas-Junior LH, Shaha C, Madhubala R. Proteomic-based approach to gain insight into reprogramming of THP-1 cells exposed to Leishmania donovani over an early temporal window. Infect Immun 2015; 83:1853-68; PMID:25690103; http://dx.doi.org/10.1128/IAI.02833-14
- Dweep H, Gretz N, Sticht C. miRWalk database for miRNA-target interactions. Methods Mol Biol Clifton NJ 2014; 1182:289-305; PMID:NOT_FOUND; http://dx.doi.org/10.1007/978-1-4939-1062-5_25
- Subramanian M, Shaha C. Up-regulation of Bcl-2 through ERK phosphorylation is associated with human macrophage survival in an estrogen microenvironment. J Immunol Baltim Md 1950 2007; 179:2330-8; PMID:17675494
- Livak KJ, Schmittgen TD. Analysis of relative gene expression data using real-time quantitative PCR and the 2(-Delta Delta C(T)) Method. Methods San Diego Calif 2001; 25:402-8; PMID:11846609; http://dx.doi.org/10.1006/meth.2001.1262
- Siqueira-Neto JL, Moon S, Jang J, Yang G, Lee C, Moon HK, Chatelain E, Genovesio A, Cechetto J, Freitas-Junior LH. An image-based high-content screening assay for compounds targeting intracellular Leishmania donovani amastigotes in human macrophages. PLoS Negl Trop Dis 2012; 6:e1671; PMID:22720099; http://dx.doi.org/10.1371/journal.pntd.0001671
- Anand S, Madhubala R. Genetically Engineered Ascorbic acid-deficient Live Mutants of Leishmania donovani induce long lasting Protective Immunity against Visceral Leishmaniasis. Sci Rep 2015; 5:10706; PMID:26035062; http://dx.doi.org/10.1038/srep10706