ABSTRACT
Autophagic degradation of proteasomes (termed proteaphagy) is a conserved mechanism by which cells eliminate excess or damaged particles. This clearance is induced rapidly when organisms are starved for nitrogen and, because proteasomes are highly abundant, their breakdown likely makes an important contribution to the amino acid pools necessary for survival. By contrast, our recent studies found that proteasomes are not degraded in response to carbon starvation, even though bulk macroautophagy is similarly activated. Instead, carbon starvation induces sequestration of proteasomes into membrane-less cytoplasmic condensates previously termed proteasome storage granules (PSGs), which protect proteasomes from autophagic degradation. Preserving proteasomes in PSGs enhances the ability of yeast cells to recover from a variety of stresses, implying that rapid remobilization of stored proteasomes when conditions improve is advantageous to cell fitness. Consequently, the choice of whether to save or degrade proteasomes can profoundly impact cell survival.
The ubiquitin-proteasome system (UPS) plays a central role in cellular proteostasis, being responsible for the degradation of many short-lived, obsolete, or dysfunctional proteins. Peptide-bond cleavage after substrate ubiquitination is catalyzed by the proteasome, a 2.5 MDa, multi-subunit proteolytic complex consisting of the 20S core protease (CP) that houses the catalytic sites for proteolysis, and the 19S regulatory particle (RP) that promotes substrate recognition, deubiquitination, unfolding, and delivery into the CP lumen. Given the critical importance of proteasomes to most facets of eukaryotic biology, it is unsurprising that this particle is regulated at multiple levels, including transcription, assembly, localization, activity, and ultimately turnover.
We and others have recently described the autophagic degradation of proteasomes (proteaphagy) in plants, yeast, and mammals as a mechanism to remove excess or damaged particles. Two genetically separable proteaphagy pathways have been uncovered, one operating in response to inhibition of the complex, and another stimulated by nitrogen deprivation. In the first pathway, inactive proteasomes are exported from the yeast nucleus to the cytoplasm and aggregate into insoluble protein deposit (IPOD)-like structures via a route dependent on the Hsp42 chaperone (). Aggregated proteasomes then become heavily ubiquitinated by one or more ubiquitin-protein ligases (E3s), which encourages recognition by the selective proteaphagy receptors Cue5 (in yeast) or RPN10 (in plants) that tether the ubiquitinated complexes to lipidated Atg8 decorating the enveloping phagophore ().
Figure 1. A model for proteasome storage versus proteasome degradation upon nutrient starvation. When cells are subjected to nitrogen or carbon starvation, proteasomes are exported from the nucleus, and the CP and RP separate. Upon nitrogen starvation, both CP and RP are rapidly encapsulated by expanding phagophores and delivered to the vacuole for degradation. Deubiquitination of one or more CP subunits by Ubp3 might be required; whether specific Atg8-binding autophagy receptors are involved remains unknown. In contrast, carbon starvation triggers CP and RP aggregation into PSGs. This requires numerous factors, including Blm10 for the CP, Spg5 and Rpn11 for the RP, and the NatB N-terminal acetylation complex for both. Preventing sequestration of proteasomes into PSGs leads to their Atg1- and Atg8-dependent degradation. An additional proteaphagic route is stimulated by proteasome inhibition. Here, proteasomes exported from the nucleus aggregate in an Hsp42-dependent manner into IPOD-like structures that are distinct from PSGs. The aggregated proteasomes are then ubiquitinated (Ub) by one or more E3 ligase(s), facilitating recognition by the selective proteaphagy receptors Cue5 or RPN10. By simultaneous interactions with Atg8/ATG8, these receptors deliver inactive proteasomes to enveloping autophagic vesicles for final degradation in the vacuole.
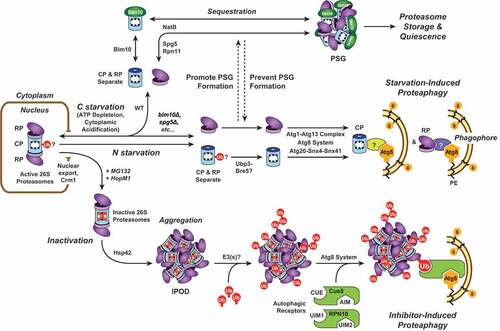
The second, less-defined proteaphagic route occurs upon nitrogen starvation, likely in parallel with the rapid upregulation of autophagic flux that occurs upon nutrient deprivation. In yeast and Arabidopsis, more than 80% of proteasomes are degraded within 8 h of transfer to nitrogen-poor growth conditions. As with nutrient-responsive bulk autophagy, this starvation-induced proteaphagy requires signalling from the Atg1 kinase complex and the core Atg8 lipidation machinery (). In addition, roles for the deubiquitinating enzyme Ubp3 and the sorting nexins Snx4/Atg24, Snx41, and/or Atg20/Snx42 have been reported. Whether starvation-induced proteaphagy in plants and yeast requires a second type of autophagy receptor is unknown; in mammalian cells, SQSTM1/p62 was reported to be essential.
While proteaphagy is clearly stimulated by nitrogen starvation, little attention had been paid to the impact of depleting other key nutrients such as carbon, phosphate, or sulfur. In a recent paper [Citation1], we described proteasome fate in response to carbon deficiency. Surprisingly, proteasomes remain stable upon carbon starvation in both yeast and Arabidopsis, even though this condition activates bulk autophagy. They also remain stable upon simultaneous nitrogen and carbon starvation, implying that carbon starvation overrides the proteaphagic response elicited by nitrogen starvation. Instead, we discovered that carbon deficiency stimulates the rapid deposition of proteasomes into cytoplasmic foci termed proteasome storage granules (PSGs). These enigmatic membrane-less condensates were discovered almost a decade ago during studies on proteasome dynamics in quiescent yeast cells. PSGs form in response to reduced ATP levels and/or cytoplasmic acidification, and they seemingly dissolve within minutes when carbon availability improves, suggesting they represent a storage form of the complex.
Key factors in PSG assembly include Blm10 (for the CP), Spg5 and the C terminus of Rpn11 (for the RP), and the NatB N-terminal acetylation complex (). Unexpectedly, the CP and RP appear to enter PSGs separately after dissociation of the holo-proteasome particle. By testing various conditions known to promote or suppress PSG assembly (low pH, ATP depletion, and mutations in various PSG assembly factors), we confirmed that sequestration of proteasomes into PSGs is antagonistic to proteaphagy; when PSG assembly is allowed, proteaphagy is absent, but when PSG assembly is blocked, proteaphagy occurs in earnest.
The sequestration of proteasomes into PSGs in yeast was previously shown to confer increased cell fitness during stationary phase and upon replicative ageing, and our study supplements these findings by clearly demonstrating that this sequestration is critical for the rapid resumption of cell growth following periods of starvation. For example, cells starved for nitrogen exhibit a 3- to 4-h lag phase before resuming growth upon return to nutrient-rich medium, whereas cells simultaneously starved for both nitrogen and carbon, or pre-treated with 2-deoxyglucose (both of which trigger PSG formation and hence proteasome preservation), resume rapid growth within only an hour. Conversely, when PSG formation is blocked and proteasomes are instead degraded (e.g., in blm10∆, nat3∆ or spg5∆ mutants), cells take much longer to resume growth when nutrient availability improves. Presumably, cells that retain proteasomes can rapidly restore degradative capacity, which we hypothesize aids resumption of cell division given the critical importance of proteasomes in controlling the timed clearance of numerous regulators responsible for cell cycle progression.
When faced with fluctuations in their local environment, including changes in nutrient availability, cells adapt by triggering dynamic re-organization of their internal constituents. In yeast, this includes formation of processing bodies, stress granules, Q bodies, and specific aggregates of individual proteins such as Rim4, Sup35 and Whi3, in addition to PSGs. An intriguing hypothesis is that cytoplasmic aggregation might safeguard proteins from unwanted breakdown. Such protection would contrast with the fact that autophagy mediates clearance of potentially toxic protein aggregates via aggrephagy, often via ubiquitination followed by SQSTM1/p62-, NBR1- or TOLLIP/Cue5-mediated delivery to the vacuole/lysosome for degradation. Consequently, mechanisms must exist that discriminate intracellular aggregates/condensates such as PSGs from seemingly related structures such as IPODs that should be enveloped and degraded by autophagy.
How membrane-less organelles such as PSGs isolate themselves from the surrounding cytoplasmic milieu is unclear, but this could involve liquid-liquid phase separation phenomena. One hypothesis is that nucleation of these condensates is driven by regions of intrinsic disorder within their constituent proteins that promote this physical separation. Intriguingly, multiple proteasome subunits contain intrinsically disordered regions (IDRs), but whether these IDRs are sufficient to drive the highly structured proteasome particles into phase separation remains speculative.
In conclusion, we discovered that the balance between proteasome preservation and degradation in response to certain starvation conditions can clearly influence the growth and survival of plants and yeast. In particular, the formation of PSGs when ATP levels are depleted and intracellular pH levels drop might represent an excellent model for identifying the critical biophysical and biochemical processes responsible for reversible protein condensation. Unravelling the mechanism(s) driving PSG formation and their subsequent evasion from autophagy should thus provide valuable information regarding cellular strategies for defending against proteotoxic stress and maximizing survival during nutrient deficiency.
Acknowledgments
The authors wish to thank Robert C. Augustine for critical reading of the manuscript.
Disclosure statement
No potential conflict of interest was reported by the authors.
Additional information
Funding
References
- Marshall RS, Vierstra RD. Proteasome storage granules protect proteasomes from autophagic degradation upon carbon starvation. eLife. 2018;7:e34532. PMID: 29624167.