ABSTRACT
Lysosomes play an essential role in quality control mechanisms by functioning as the primary digestive system in mammalian cells. However, the quality control mechanisms governing healthy lysosomes are not fully understood. Using a method to study lysosome membrane turnover, we discovered that LC3-lipidation on the lysosome limiting membrane is involved in invagination and formation of intralumenal vesicles, an activity known as microautophagy. This activity occurs in response to metabolic stress, in the form of glucose starvation, or osmotic stress induced by treatment with lysosomotropic compounds. Cells rendered deficient in the ability to lipidate LC3 through knockout of ATG5 show reduced ability to regulate lysosome size and degradative function in response to stress. These findings demonstrate that cells can adapt to changing metabolic conditions by turning over selective portions of the lysosomal membrane, using a mechanism that involves lysosome-targeted LC3 lipidation and the induction of selective microautophagy.
KEYWORDS:
The lysosome is an essential eukaryotic organelle that functions as the central cellular digestive system, mediating the turnover of extracellular and intracellular material delivered through endocytic pathways or through cytoplasmic sequestration by macroautophagy (referred to here as autophagy). Lysosomes play important roles in cellular quality control by degrading damaged proteins, protein aggregates, and even whole organelles. Dysfunction of the autophagy-lysosome pathway is implicated in a variety of human diseases, from cancer to neurodegeneration, underscoring a need to further understand its regulation. While mechanisms that control the capture and delivery of substrates to the lysosome have been well studied, by contrast how the lysosome itself is regulated in order to maintain optimum function is less understood.
One mechanism that can eliminate dysfunctional lysosomes is an autophagy-dependent form of turnover called “lysophagy.” Lysophagy is triggered when lysosome membranes become ruptured or damaged, for example as a result of treatment with agents that disrupt lysosomal membrane integrity such as the dipeptide compound L-leucyl-L-leucine methyl ester (LLOMe). The presence of damaged lysosomes can be detected through an imaging-based approach utilizing expression of a cytosolic GFP-tagged LGALS/Galectin reporter protein (e.g., green fluorescent protein [GFP]-LGALS3), which binds to beta-galactosides that become exposed on ruptured lysosomal membranes, leading to the formation of cytoplasmic puncta. The use of such imaging-based reporters has enabled the discovery of mechanisms such as lysophagy that can respond to lysosome damage, but mechanisms that might control lysosome turnover in the absence of membrane rupture have remained more obscure.
In our recent study [Citation1], we developed a method to measure lysosome turnover involving quantification of GFP cleavage from tagged lysosomal transmembrane proteins, an approach that takes advantage of the intrinsic resistance of GFP to lysosomal digestion, and can measure turnover occurring even in the absence of membrane rupture. We demonstrated that this method can be used to quantify LLOMe-induced lysophagy, and sought to identify other conditions that might lead to lysosome turnover. By interrogating the effects of starvation for different nutrients, we found that starvation for glucose, but interestingly not amino-acid starvation, led to significant lysosome turnover, in a manner involving the catabolism of glutamine and subsequent generation of ammonia, a byproduct of glutaminolysis that accumulates within lysosomes.
As the generation of ammonia in glucose-starved cells has been shown to lead to the induction of autophagy, we considered if autophagic clearance of lysosomes, or lysophagy, might play a role in the observed turnover. By interrogating the requirement for different autophagy genes, we indeed found that lysosome turnover was regulated by ATG5 (autophagy related 5), but curiously not by ATG13, suggesting the involvement of an autophagy-related, but potentially non-canonical mechanism. One non-canonical mechanism that can target lysosomes and is also induced by ammonia involves the ATG5-dependent direct lipidation of the autophagy protein MAP1LC3/LC3 (microtubule-associated protein 1 light chain 3) onto lysosomal membranes. Consistent with the involvement of this mechanism, we found that LC3 was lipidated onto lysosomal membranes in response to ammonia, and that treatment with additional compounds reported to induce this mechanism, including nigericin and monensin, also led to significant lysosome turnover.
To try to understand why lysosomes were undergoing turnover in response to these stressors, we investigated if lysosome damage might occur in response to glucose starvation or treatment with ammonia, but did not observe the formation of GFP-LGALS3 puncta, suggesting that lysosomes were not being cleared in response to membrane damage. Because turnover appeared to involve the direct lipidation of LC3, we next considered if invagination of the lysosome membrane and formation of intralumenal vesicles, an activity referred to as microautophagy, might occur in this context. Ultrastructure analysis of ammonia-treated cells revealed a striking increase in multivesicular structures, appearing in an ATG5-regulated manner, and similar results were observed through confocal imaging, consistent with lysosome membrane turnover occurring through the induction of microautophagy.
We then asked by expanding our GFP-tagged reporters which lysosomal membrane proteins undergo turnover through this mechanism. Surprisingly, we could not detect appreciable turnover of GFP-tagged LAMP1 (lysosomal-associated membrane protein 1) or SLC66A1/PQLC2 (solute carrier family 66 member 1) amino acid transporter in response to glucose starvation or treatment with ammonia, even though these reporters showed robust turnover during LLOMe-induced lysophagy. Conversely, GFP-tagged versions of MCOLN1/TRPML1 (mucolipin 1) and SLC38A7/SNAT7 exhibited significant turnover in response to both glucose starvation and ammonia, and the CTNS (cystinosis, nephropathic) amino acid transporter showed an intermediate level of turnover, suggesting overall that microautophagy activated under these conditions targets transmembrane proteins in a selective manner ().
Figure 1. Mechanisms of lysosome membrane turnover. Whole lysosomes are turned over through a macroautophagy-dependent mechanism called lysophagy upon lysosome membrane rupture (left). Selective lysosome membrane turnover can occur, independent of membrane rupture, through a separate mechanism involving direct membrane invagination into the lysosome lumen called microautophagy (right)
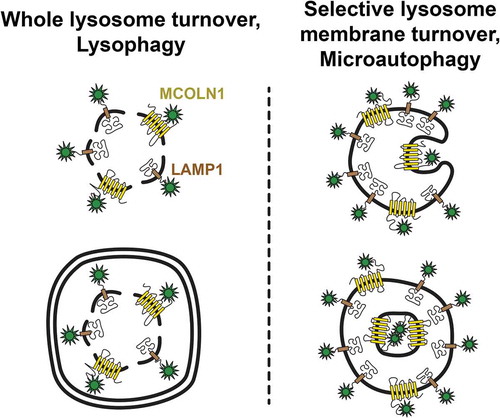
Our study has uncovered a new mode of selective microautophagy that occurs in response to ATG5-regulated LC3-lipdiation on the lysosomal membrane, an activity that we propose remodels lysosomes in response to metabolic stress. We showed that this form of selective microautophagy is indeed important for regulating lysosome size and degradative capacity. Future studies will be aimed at uncovering through the use of additional reporter proteins or proteomics approaches how lysosomes are remodeled, and how the selective turnover of particular proteins may contribute to size control or degradative functions. It is possible that membrane remodeling through microautophagy may be induced in response to other stresses as well, in such a manner as to tune lysosome function to different cellular demands. In addition to lysosome biogenesis, which occurs in response to stress, we propose that selective lysosome membrane turnover is important for the adaptation of lysosome function in stressed cells.
Disclosure statement
All authors declare no conflict of interest.
Additional information
Funding
Reference
- Lee C, Lamech L, Johns E, et al. Selective lysosome membrane turnover is induced by nutrient starvation. Dev Cell. 2020;55(3):S1534-5807(20)30666–3