ABSTRACT
RB1CC1/FIP200 is a subunit of the ULK1 complex in more complex eukaryotes. This large polypeptide was proposed to be a functional homolog of the Atg17 and Atg11 scaffolding proteins in yeast. Previous studies showed that RB1CC1 can bind to various proteins of the macroautophagy/autophagy machinery, where the RB1CC1 Claw domain directly interacts with a short linear segment of its interactors. A mechanistic insight into how the small globular RB1CC1 Claw domain can interact with such an array of structurally variable proteins has been elusive. The recent study by Zhou et al., discussed here, yields structural data that not only provide a unifying mechanistic explanation of these interactions, but also reveals previously unknown RB1CC1 interactors and opens a new field for exploration of autophagy regulation.
Abbreviations: FIR: FIP200-interacting region; LIR: LC3-interacting region; pS/p-S: phosphorylated serine
The ULK1 complex is an indispensable component of the core molecular machinery in mammalian autophagy. The complex consists of four subunits, a serine-threonine ULK1/2 kinase, ATG13, ATG101, and RB1CC1/FIP200, and functions in the initiation of autophagy nucleation under stress conditions [Citation1,Citation2]. However, recent studies showed that the ULK1 complex also participates in selective autophagy regardless of nutrient status. Specifically, the RB1CC1 Claw domain recognizes a short FIP200-interacting region (FIR) in SQSTM1/p62, a selective autophagy receptor for degradation of ubiquitinated proteins [Citation3], or in CCPG1, a reticulophagy receptor [Citation4]. The FIR motif was also found in ATG16L1 [Citation5,Citation6] and in TBK1 kinase adaptors, TBKBP1/SINTBAD and AZI2/NAP1 [Citation7]. Furthermore, the RB1CC1 Claw domain carries similarities to the C terminal domain of Atg11 that interacts with Atg19, a receptor for the selective cytoplasm-to-vacuole targeting pathway in yeast [Citation8,Citation9]. All these interactions, however, lack a mechanistic explanation. Whether there is a general binding mode between the FIR regions and the RB1CC1 Claw domain is unknown.
Zhou et al. [Citation10] used biochemical and biophysical approaches in combination with crystallography to probe binding of the RB1CC1 Claw domain to the second FIR motif (FIR2) of the reticulophagy receptor CCPG1 or to OPTN (optineurin), an important selective-autophagy receptor for the clearance of pathogens (xenophagy) [Citation11], protein aggregates (aggrephagy) [Citation12], or mitochondria (mitophagy) [Citation13]. These researchers found that both interactions are phosphorylation dependent. Specifically, phosphorylation at the S104 residue in the synthetic 13-residue CCPG1 FIR2 peptide (SDDpSDIVTLEPPK) increases binding affinity of the peptide to the RB1CC1 Claw domain (residues 1490–1594) ~17-fold. In comparison, the TBK1 kinase phosphorylates OPTN at Ser177, a residue directly preceding the LIR motif (F/Y/W_x_x_I/L/V), and this posttranslational modification increases the affinity of binding between the RB1CC1 Claw domain (residues 1490–1594) and phosphorylated OPTN (residues 169–185; SSEDpSFVEIRMAE) ~27-fold, in comparison to the unphosphorylated peptide. Together these data on CCPG1 and OPTN show, in agreement with the previous report [Citation3], that several selective autophagy receptors interact with the RB1CC1 Claw domain in a phosphorylation-dependent manner.
To obtain a mechanistic insight into these interactions, Zhou et al. applied crystallography to solve the structure of the RB1CC1 Claw domain in complex with the phosphorylated CCPG1 FIR2 or OPTN peptides. The RB1CC1 Claw-p-CCPG1 FIR2 structure at 1.4 Å resolution showed that the monomeric RB1CC1 Claw-domain architecture assembles as a 5-stranded anti-parallel β-sheet, an α-helix, and an N terminal β0-strand that packs with the β0-strand of the second monomer, together forming an anti-parallel homodimer. This homodimer binds two p-CCPG1 FIR2 peptides and, thereby, forms a hetero-tetramer with 2:2 stoichiometry. Each p-CCPG1 FIR2 peptide packs with β4 and β5 strands and their connecting loop in the RB1CC1 Claw domain. Detailed analysis showed that hydrogen bonds and salt bridges involve D103, p-S104, D105, V107, T108, and E110 of the peptide, and E1563, Y1564, Q1566, K1568, K1569, and R1573 in the RB1CC1 Claw domain. In addition, I106 and L109 in the peptide occupy a large hydrophobic pocket/LHP, formed by C1565, A1567, F1574, V1576, and F1582, and a small hydrophobic groove/SHG, formed by Y1564 and the aliphatic chain of K1581, in the Claw domain, respectively. These interactions were confirmed by data showing that point mutations of key interface residues disrupt the formation of the RB1CC1 Claw-p-CCPG1 FIR2 complex. The crystal structure of the RB1CC1 Claw-p-OPTN LIR complex at 2 Å resolution revealed an overall binding mode similar to that for the RB1CC1 Claw-p-CCPG1 FIR2 structure. The OPTN peptide forms a β-strand in anti-parallel fashion with the β4 strand of the RB1CC1 Claw domain. Despite this similarity, a few modifications were observed relative to p-CCPG1 FIR2. The aromatic side chain of OPTN F178 occupies the large hydrophobic pocket, and forms a unique cation-π interaction with RB1CC1 R1584. Additional hydrophobic contacts were observed between OPTN V179 and I181 and the aliphatic chain of RB1CC1 K1568. Hydrogen bonds and salt bridges in the RB1CC1 Claw-p-OPTN LIR complex involve E175, D176, p-S177, V179, and I181 of the peptide and Y1564, Q1566, K1568, K1569, R1573 in RB1CC1. Again, point mutations of key interface residues disrupt the complex.
Although the monomeric structures are highly similar, comparison of the overall dimeric conformation of the RB1CC1 Claw domain in the apo-form (PDB ID:6DCE) relative to that in the RB1CC1 Claw-p-CCPG1 FIR2 and RB1CC1 Claw-p-OPTN LIR complexes revealed that binding of the p-CCPG1 FIR2 peptide causes a mild conformational shift in the RB1CC1 Claw domain homodimer relative to the conformation of the homodimer in the apo-form and in the complex with OPTN. In particular, the p-CCPG1 FIR2 binding causes an opening rearrangement of the homodimer. A functional relevance of this structural shift remains to be elucidated.
The phosphomimetic mutants CCPG1S104E and OPTNS177E exhibit a good ability to bind the RB1CC1 Claw domain, suggesting that the domain recognizes also a negatively charged residue, in the binding region of an interactor, in a phosphorylation-independent manner. In addition, molecular modeling analyses showed that the LIR sequence in SQSTM1/p62, NBR1, and RETREG1/FAM134B can have a similar binding mode with the RB1CC1 Claw domain as CCPG1 and OPTN. A sequence alignment of these RB1CC1 Claw domain-binding segments with the binding segments of other RB1CC1 Claw domain interactors, such as ATG16L1, AZI2/NAP1, or TBKBP1/SINTBAD, led to an idea that there can be a common binding mode between the RB1CC1 Claw domain and its interactors. Because the RB1CC1 Claw domain can clearly accommodate aromatic residues in the LIR motifs and Asp or Glu can replace phosphorylated Ser or Thr, Zhou et al. propose a consensus sequence of the RB1CC1 Claw domain interactors to be D/E/pS/pT_F/Y/W/I/L/V_x_x_I/L/V, where x can be any amino acid residue, and name it the FIR core motif. If the first residue in the motif is Asp or Glu, such a motif is phosphorylation independent, but can be further enhanced by either phosphorylation of a Ser or Thr residue or a negatively charged Asp or Glu residue preceding the FIR core motif. The RB1CC1-interacting proteins such as CCPG1, ATG16L1, AZI2/NAP1, TBKBP1/SINTBAD, SQSTM1/p62, NBR1, or RETREG1/FAM134B have Asp as the first acidic residue in the FIR core motif, whereas OPTN utilizes phosphorylated serine in this place, which makes the OPTN FIR phosphorylation dependent.
Zhou et al. also tested binding of CCPG1 FIR2 to six mammalian Atg8 orthologs. They found a direct interaction with all Atg8-family proteins, where family members have distinct binding affinities further enhanced by the phosphorylation of S104. This finding is intriguing, because it not only shows that the LIR motif and the FIR core motif overlap (, left panel), but also that the binding surfaces in Atg8-family proteins and the RB1CC1 Claw domain can compete for the same binding segment of their LIR- or FIR-containing interactors. Zhou et al. confirmed this phenomenon experimentally by NMR-based titration assays, where they showed a competition between the RB1CC1 Claw domain and GABARAP for binding p-CCPG1 FIR2. Here, we use OPTN as an example to visualize the binding of its LIR/FIR to the two competing globular structures, LC3B and the RB1CC1 Claw domain (, middle and right panels).
Figure 1. The LIR motif, for binding to Atg8-family proteins, and the FIR core motif, for binding to the RB1CC1 Claw domain, overlap. Left panel, comparative schematic representation of the LIR motif (F/Y/W_x_x_I/L/V) and the FIR core motif (D/E/pS/pT_F/Y/W/I/L/V_x_x_I/L/V) in autophagy proteins. The legend shows properties of amino acid residues. Both motifs can be preceded by acidic residue(S) (pink) that enhance the binding affinity between the LIR or FIR and Atg8-family proteins or the RB1CC1 Claw domain, respectively. Middle panel, the crystal structure (PDB ID: 2LUE) of the LIR motif (FVEI) in OPTN (multicolored extended/β-stand conformation) bound to LC3B (light gray). The serine residues N-terminal to the LIR can be phosphorylated, especially S177, which enhances the binding affinity between the OPTN LIR and LC3B. Right panel, the crystal structure (PDB ID: 7CZM) of the FIR core motif (multicolored extended/β-stand conformation) in OPTN bound to the RB1CC1 Claw domain (pale green). The two negatively charged residues, E175 and D176, N-terminal to the FIR enhance the binding affinity between the OPTN FIR and the Claw domain. The RB1CC1 Claw domain is visualized as a homodimer that binds two FIR core motifs, forming together a hetero-tetramer with 2:2 stoichiometry.
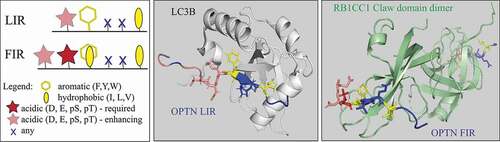
The report by Zhou et al. reveals a puzzling question as to why does the mammalian autophagy machinery have a use for two overlapping motifs that can competitively bind to two, functionally different, globular structures? A plausible explanation can be that the purpose of this competitive mechanism is a layer of autophagy regulation that is currently unknown. The LIR is a central, indispensable motif for the functional autophagy pathway, and, so far, more than eighty LIR-containing proteins are recognized [Citation14]. How many LIR-containing proteins are also FIR-containing polypeptides? Answering this question through future research will likely reveal a complex protein network with an enormous regulatory potential. To mention one example, we looked at the disordered LIR-containing sequence in RB1CC1. The RB1CC1 LIR motif, 702FETI [Citation15], is the phosphorylation-independent FIR core motif, DFETI, preceded by the upstream sequence, 691SPDSIDAHTF, containing multiple phosphorylable/acidic residues. Can the RB1CC1 FIR sequence bind the RB1CC1 Claw domain and, thereby, function as a regulation-prone autoihibitory tool that can prevent the Claw domain from binding to its interactors? Also, can any unknown architecture of many proteins associated with mammalian autophagy form a Claw-like domain? These and other interesting questions arising from the study by Zhou et al. open new gates in the insufficiently explored field of autophagy regulation. Finally, the overlap or fast, phosphorylation-dependent conversion between the LIR and FIR core motifs offers remarkable versatility, and indicates that investigations of the famous LIR motif are far from being over.
Disclosure statement
No potential conflict of interest was reported by the author(s).
Additional information
Funding
References
- Zachari M, Ganley IG. The mammalian ULK1 complex and autophagy initiation. Essays Biochem. 2017;61(6):585–596. PubMed PMID: WOS:000450754800003; English.
- Lin MG, Hurley JH. Structure and function of the ULK1 complex in autophagy. Curr Opin Cell Biol. 2016 Apr;39:61–68. PubMed PMID: 26921696.
- Turco E, Witt M, Abert C, et al. FIP200 claw domain binding to p62 promotes autophagosome formation at ubiquitin condensates. Mol Cell. 2019 Apr 18;74(2):330-+. PubMed PMID: WOS:000465184200012; English.
- Smith MD, Harley ME, Kemp AJ, et al. CCPG1 is a non-canonical autophagy cargo receptor essential for ER-phagy and pancreatic ER proteostasis. Dev Cell. 2018 Jan 22;44(2):217-+. PubMed PMID: WOS:000423448400010; English.
- Gammoh N, Florey O, Overholtzer M, et al. Interaction between FIP200 and ATG16L1 distinguishes ULK1 complex-dependent and -independent autophagy. Nat Struct Mol Biol. 2013 Feb;20(2):144–149. PubMed PMID: 23262492.
- Nishimura T, Kaizuka T, Cadwell K, et al. FIP200 regulates targeting of Atg16L1 to the isolation membrane. EMBO Rep. 2013 Mar 1;14(3):284–291. PubMed PMID: 23392225.
- Ravenhill BJ, Boyle KB, von Muhlinen N, et al. The cargo receptor NDP52 initiates selective autophagy by recruiting the ULK complex to cytosol-invading bacteria. Mol Cell. 2019 Apr 18;74(2):320–329 e6. PubMed PMID: 30853402.
- Pfaffenwimmer T, Reiter W, Brach T, et al. Hrr25 kinase promotes selective autophagy by phosphorylating the cargo receptor Atg19. EMBO Rep. 2014 Aug;15(8):862–870. PubMed PMID: 24968893.
- Tanaka C, Tan LJ, Mochida K, et al. Hrr25 triggers selective autophagy-related pathways by phosphorylating receptor proteins. J Cell Biol. 2014 Oct 13;207(1):91–105. PubMed PMID: 25287303.
- Zhou Z, Liu J, Fu T, et al. Phosphorylation regulates the binding of autophagy receptors to FIP200 claw domain for selective autophagy initiation. Nat Commun. 2021 Mar 10;12(1):1570. PubMed PMID: 33692357.
- Wild P, Farhan H, McEwan DG, et al. Phosphorylation of the autophagy receptor optineurin restricts Salmonella growth. Science. 2011 Jul 8;333(6039):228–233. PubMed PMID: 21617041.
- Li F, Xie X, Wang Y, et al. Structural insights into the interaction and disease mechanism of neurodegenerative disease-associated optineurin and TBK1 proteins. Nat Commun. 2016 Sep 13; 7:12708. PubMed PMID: 27620379.
- Heo JM, Ordureau A, Paulo JA, et al. The PINK1-PARKIN mitochondrial ubiquitylation pathway drives a program of OPTN/NDP52 recruitment and TBK1 activation to promote mitophagy. Mol Cell. 2015 Oct 1;60(1):7–20. PubMed PMID: 26365381.
- Sora V, Kumar M, Maiani E, et al. Structure and dynamics in the ATG8 family from experimental to computational techniques. Front Cell Dev Biol. 2020;8:420. PubMed PMID: 32587856.
- Alemu EA, Lamark T, Torgersen KM, et al. ATG8 family proteins act as scaffolds for assembly of the ULK complex: sequence requirements for LC3-interacting region (LIR) motifs. J Biol Chem. 2012 Nov 16;287(47):39275–39290. PubMed PMID: 23043107.