ABSTRACT
Moisture plays a key role in rock decay in the built and natural environments. Rock-cut sites are particularly vulnerable to moisture-related weathering as they are carved into rock outcrops and do not have impermeable foundations or roofs to retard the flow of moisture. To characterise the moisture dynamics and its influence on weathering of rock-cut sites, we undertook a moisture monitoring campaign using a non-destructive Microwave Moisture Measurement System (MMMS) at two monolithic rock-cut churches in Lalibela, Ethiopia. The results showed that the walls were more saturated at depth than on the surface during the wet season. This suggests that low surface temperature and higher moisture content at depth will lead to constant-rate drying and accumulation of salts on the surface of the walls during the wet season. In the dry season, there was higher saturation near the surface than at depth (falling-rate drying). High rock surface temperature during the dry season contributes to subsurface drying and accumulation of salts below the surface. This seasonally shifting moisture dynamics will lead to a complex and dynamic damage profile. This study highlights the significant wetting facilitated by a lack of impermeable roofs and foundations at rock-cut structures during rainy periods.
1. Introduction
Moisture plays a crucial role in rock decay in both built and natural environments. Processes that cause decay, such as wetting-drying, salt efflorescence and hygric expansion-contraction, are induced by fluctuations in moisture content. Understanding the spatial and temporal variation of moisture (moisture dynamics) in porous building materials is therefore essential to diagnosing rock decay in the built environment. Three sets of factors control moisture transport within porous building materials: environmental, material and structural characteristics (Hall and Hoff Citation2003). The amount of rain, the duration of rainy periods and the range of temperature fluctuation are key environmental factors that determine moisture dynamics (McCabe et al. Citation2013). Material properties such as the amount of porosity and pore network connectivity also contribute to moisture dynamics. Structural factors, such as building type, whether it is a masonry structure composed of mortar joints and stones or a carved rock-cut site, and wall thickness will hugely influence the rate of moisture transport and evaporation. These three sets of factors collectively determine the rate of drying (evaporation) in porous building materials. Constant-rate (stage I) drying or falling-rate (stage II) drying will occur depending on the environmental conditions and material properties. Constant-rate drying occurs when there is a connected hydraulic pathway that can efficiently transport liquid water to the drying surface of a material; whereas falling-rate drying refers to a slow rate of evaporation where flow to the surface stops and liquid is removed from the surface as vapour through diffusion (Scherer Citation1990).
Much focus has been given to studying moisture transport in masonry and rock walls in temperate climates (Hall Citation2004; Hall et al. Citation2011; McAllister et al. Citation2016; Sass and Viles Citation2006, Citation2010; Smith et al. Citation2011), but other areas, including tropical climates, have received less attention, especially those with strong seasonal contrasts. Many culturally important rock-cut heritage sites exist in climates characterised by seasonal rains, such as Petra in Jordan, Ellora in India, Lalibela in Ethiopia, and several rock-cut sites in Northern Ethiopia. Rock-cut sites that are usually found carved into hills or on cliff faces are homogeneous in composition aside from inherent lithological variation that might be present. They have a unique method of construction, they are made through a subtractive process that involves removing material to create spaces. The output of these works of architecture are structures that are whole in the sense that they are rock masses from which space has been carved out. The roof, walls and base of the structure are all one body of mass or monolithic structure. The wholeness or homogeneity of rock-cut buildings influences how moisture is transported throughout these structures (Sass and Viles Citation2022). Moreover, rock-cut structures are often carved into outcrops that are easy to work (Asrat Citation2002), which usually means the rocks have poor mechanical properties and higher permeability and porosity. Therefore, the moisture dynamics in this type of structure is likely to be different from that of masonry structures that usually have a semi-impermeable foundation that prevents groundwater uptake and a roof that stops rain infiltration. In this study, the spatial and temporal variation in moisture content of the rock-cut walls in the churches at Lalibela is assessed to gain an understanding of the nature of moisture dynamics in rock-cut structures. While rain infiltration and groundwater uptake have been implicated for the significant moisture-related damage in the lower and upper parts of the churches at Lalibela, thus far there hasn’t been any study to confirm this hypothesis. Using field-based monitoring of moisture and onsite observation at Lalibela this study will show the nature of moisture dynamics in rock-cut structures found in seasonal climates.
The main objectives of this study are to identify the temporal and spatial moisture distribution on the surface and subsurface of the rock-cut walls at Lalibela during the wet and dry seasons.
1.1. Study site and research aims
The UNESCO world heritage site of Lalibela is a large complex of rock-cut churches that are carved in the base of Abune Yoseph Mountain in northern Ethiopia. The eleven churches are carved below the ground level and are connected via underground tunnels and trenches. They were built between the 11th to the 13th century CE (Derat et al. Citation2021; Gobezie, Citation2018). They are considered as one of the holiest sites in the Ethiopian Orthodox Church and have been a place of pilgrimage and worship since their establishment in the medieval period (Woldeyes Citation2019). Two monolithic churches at Lalibela were selected for this study: Bete Amanuel and Bete Giyorgis (). Bete Amanuel has a protective open shelter which was installed primarily to stop rain infiltration during the wet season. These two churches were selected to assess the impact of an open shelter in limiting moisture ingress in rock-cut structures that are in climates prone to extreme seasonal variation in rainfall.
2. Methodology
There are three seasons in Lalibela: short rains (Feb–May), main rains (Jun–Sep) and dry (Oct–Jan) seasons. The main rains season is the wettest period and accounts for most of the annual rainfall. In contrast, the dry season is characterised by high rock surface temperatures, low humidity and less than 10 rain days throughout the whole period. Given the difference in rainfall and temperature between the wet and dry seasons, moisture dynamics in these two seasons is likely to be hugely dissimilar. Spatial variation in moisture content in Bete Giyorgis and Bete Amanuel was monitored in the wettest and driest periods of the year to assess the range of temporal variation in moisture in the rock-cut walls. The seasonal variation of the height of moisture rise can also be determined by measuring the spatial variation in moisture seasonally. A handheld non-destructive moisture measuring system was used to measure the moisture content onsite. To meaningfully interpret data collected onsite into spatial variation in saturation, the moisture measuring system was gravimetrically calibrated in a controlled laboratory setting. The temporal variation in moisture content is presented as percentage increase between the dry and wet seasons. Moisture monitoring and interpretation were complemented with onsite observation of moisture-related damage.
2.1. Deterioration survey
Photographic survey and on-site observation (done in 2018 and 2019 field seasons) were used to assess areas of the façade that were damaged or restored. The drawings show moisture-related damage observed on site. This simple assessment allowed us to characterise the spatial distribution and patterns of damage as they appear at Bete Amanuel and Bete Giyorgis. Rock decay features are identified through the use of the ICOMOS-ISCS Illustrated Glossary on Stone Deterioration (Citation2008) and the terminology used to describe decay features is also based on this glossary.
2.2. Microwave Moisture Measurement System (MMMS) and principle
To monitor moisture distribution in porous building materials non-destructive methods that are capable of scanning large areas of a surface at different depths are preferred (Pinchin Citation2008). The Moist 350B Microwave Moisture Measurement System (MMMS) from hf sensor GmbH (Leipzig, DE), which was used in this study, is a suitable technique for field measurements because it is portable, negligibly sensitive to salts and optimised for simple acquisition of data in a grid pattern (Lataste and Göller, Citation2018). This method is well suited for monitoring the temporal and spatial variation of moisture. In this study, two sensors with a penetration depth of up to 2–3 cm (surface sensor—R1M V2) and up to 20–30 cm (subsurface sensor—PM V2) (hf sensor GmbH Citation2014) were used.
The MMMS is a dielectric moisture measurement method that takes advantage of the significant difference in dielectric permittivity between water and most building materials. MMMS can be applied effectively in ambient temperatures of 0–50°C (hf sensor GmbH Citation2014). Both rock-surface and ambient temperature did not exceed 50°C at Lalibela during the periods moisture was monitored. The device uses sensing heads that have a microwave source and detector to measure the reflection coefficient (the ratio of the reflected and transmitted wave) and gives an output in Moisture Index (MI). MI is a unitless value that is a result of the reflection coefficient multiplied by a fixed factor (Lataste and Göller Citation2018). MI is not an absolute measure of moisture content, but as it is related to the microwave reflection coefficient which is determined by the amount of moisture present in the material, it can be usefully employed to indirectly measure moisture content in stone-built heritage (Orr et al. Citation2020). The range in MI between wet and dry states will vary depending on the physical property of the rock. To reliably represent moisture content from MMMS readings, studies have relied on gravimetric calibration (Gartner Citation2010) and percentile representation (Orr et al. Citation2020). Percentile representations are advantageous in that they allow direct interpretation of field data without additional laboratory analysis. However, in data sets that are representative of upper and lower limits of saturation (wet vs dry season) percentile ranking would lead to measurement values clustering near 0 and 100. The advantage of gravimetric calibration is that it determines the range of MI that corresponds to a wider range of unsaturated moisture contents for the rock type under study. It also allows comparison between the surface and subsurface sensors which do not have comparable scales of MI. Laboratory-based gravimetric calibration is used in this study to assess the responsiveness of the MMMS to a range of unsaturated moisture contents for the Lalibela basaltic scoria.
2.3. Gravimetric calibration protocol
The efficacy of the microwave moisture meter in accurately sensing a range of moisture contents for different building stones (Blaeuer et al. Citation2009; Kurik et al. Citation2017; Orr et al. Citation2020) and brick (Gartner Citation2010) has been tested in laboratory settings with different set ups. Granite, limestone, dolostone and sandstones have been the focus of these studies but as yet no studies have been done to test the MMMS on basaltic scoria (as found in Lalibela). To validate how effectively this device senses a range of moisture contents for this particular rock type, gravimetric assessment was done. The sample used in this study was extracted from the Yohannes Amba quarry (2 km from the church complex) and has similar petro-physical properties to the rock the churches are carved into (). The dry weight of the samples was determined following BS EN 16682 (BSI Citation2017) which involved drying the samples at 105°C in a ventilated oven until their mass differed by less than 0.5% in consecutive weighting. After drying for 72 h, the sample were cooled in a desiccator, and 3 moisture readings were taken on each face of the block (generating 18 measurements per block). Measurements were taken at different moisture content levels after the samples were saturated with deionized water for 3 h and allowed to dry under laboratory conditions. The sample was placed on a stone slab during measurements to ensure there was no interference from metallic objects under the laboratory counter.
Table 1. Physical properties of samples used for gravimetric analysis in comparison with data from the churches bete amanuel and bete giyorgis.
Linear regression analysis was done to determine the strength of the relationship between MI values and gravimetric water contents (). This analysis showed a consistent linear (R2 = 0.95) relationship between gravimetric measurements of water contents and MI values for both the sensors used in this study. The range of moisture index measurements from dry to saturated is comparable for the surface and subsurface probes. Given that the range of moisture index and the slope of the regression model of both the probes is comparable; it is reasonable to assume that both probes will respond at a similar rate to an increase in moisture content. Overall, the linear regression assessment showed that the MMMS is equally and sufficiently responsive across the whole range of moisture contents from saturated to dry for the Lalibela basaltic scoria. The gravimetric calibration therefore demonstrates that data collected in the field can be meaningfully interpreted to compare the gradient in moisture content from the surface to the subsurface.
Figure 2. Gravimetric analysis regression models of the saturation range (saturated to oven dry) in ambient drying conditions for the surface (red) and subsurface (black) sensors. The vertical bars represent the standard deviation from the mean of the measurements taken at a range of saturation (%).
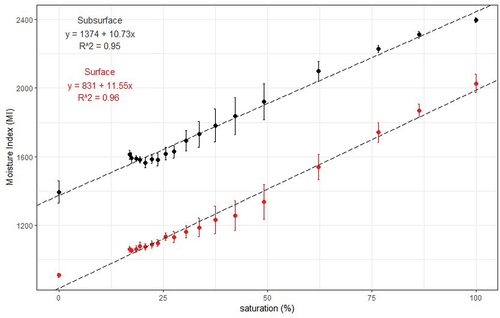
2.4. Data acquisition
The gravimetric assessment showed that the MMMS is able to accurately measure a range of unsaturated moisture contents accurately for basaltic scoria. By taking measurements in the same area at different times of the year, any impact of the heterogeneity in petro-physical properties on measurements can be discounted for assessments of temporal variation. The MMMS was used to take moisture readings on four zones in Bete Giyorgis and four zones in Bete Amanuel in the wet and dry seasons (). Moisture content measurements were taken at regularly spaced points on selected areas of the walls, in a grid pattern (9 rows by 7 columns) with each point 15 cm apart starting from the base of the churches (). At each of the 63 points of measurement, readings were taken with the MMMS surface and subsurface sensors. The areas that were measured were selected based on their accessibility and preservation state. The lower parts of the building that were likely to absorb moisture via capillary uptake were selected to determine the height of moisture rise. The west-facing wall at Bete Amanuel was excluded because the lower part of this facade has been restored with cement-based mortar (). As the range of MI will differ for dissimilar materials, the comparison of moisture content between the original material (basaltic scoria) and restored areas may be misleading. The north-facing wall at Bete Giyorgis was also excluded for similar reasons.
Figure 3. Orthophotos (@Archeovision 2019) of facades of (a) Bete Amanuel (BA14) and (b) Bete Giyorgis (BG1-4) showing zones where measurements were taken. Areas shaded in red and purple are indicating weathered and restored areas, respectively. A close up of areas in the blue squares are presented in
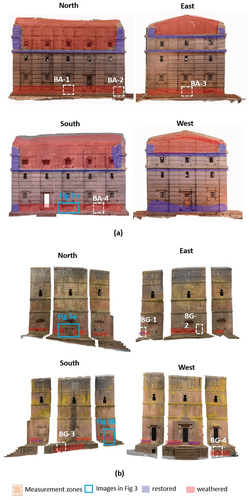
2.5. Data handling and representation
Data acquired in the field are represented in two ways: (1) Percentage increase (% increase) is used to determine the temporal variation in moisture content between the dry and wet seasons. The mean of the 63 readings taken in each zone are used to assess the overall increase in moisture seasonally and to compare the % increase between zones and churches. (2) To assess the spatial distribution of moisture on the surface and subsurface, MI values are converted to percent saturation (%) by using the gravimetric calibration function. Spatial distribution of moisture is represented with saturation (%).
3. Results
3.1. Moisture-related deterioration
Moisture transport through historic rock-cut structures is very different to historic masonry structures. Rock-cut structures are usually found as elements within a hillslope or underground. They are often found in a setting where they are part of the natural catchment hydrological system (Sass and Viles Citation2022) and do not have an impermeable roof or foundation to prevent moisture ingress. These factors will hugely influence moisture transport in rock-cut structures. At Lalibela, the climate is characterised by short intense wet periods and long dry periods. The thick (2 m) gable and flat roofs of the churches are directly exposed to the intense rainfall during the wet season. The base of the structures are affected by moisture absorbed through groundwater uptake. Wind-driven rain is likely to affect the facades to a limited extent, as the churches are underground and are closely surrounded by trench walls. Onsite observation indicates that the areas exposed to direct rainfall and groundwater uptake, the upper and lower parts of the structures, are the most affected by moisture-related damage ().
The type and severity of moisture-related damage varies between the churches. Bete Giyorgis is in a very good state of preservation in comparison to the other churches, the upper part of this church has been discoloured by biological soiling (see )) but has not suffered from extensive damage like the roof at Bete Amanuel (see )). Coving (a cavity formed by granular disintegration) has formed 30 to 60 cm above the base of Bete Giyorgis due to scaling caused by sub-florescence of salts ()). At Bete Amanuel, both the upper and lower parts of the church are damaged. The roof has several cracks and large portions of it have disappeared overtime. It was severely damaged in the late 19th and early 20th century and had to be restored several times in the mid-20th century to prevent infiltration of rain (Taye, Fusade, and Viles Citation2020). Currently, there are several cracks on the ceiling and a shelter has been placed over this church to protect it from further damage by rain. The four walls of Bete Amanuel are significantly weathered due to scaling on the lower parts (60–150 cm above the base of the wall) of the walls ). Bete Giyorgis is in a much better preservation state than Bete Amanuel. While both churches show most of the damage where they come in contact with moisture (see red shaded areas in ), Bete Amanuel, due to reasons that may be associated with the size and stress field, appears to have suffered the most damage.
3.2. Temporal variation
A good indication of the range of moisture fluctuation across the year is the difference in moisture content between the driest and wettest periods or seasons. The difference in moisture content between the dry and wet seasons on the surface and subsurface of the rock walls is presented as % increase in Moisture Index (MI) in . The range of moisture fluctuation on the surface was between 6% and 31% increase and between 16% and 42% increase on the subsurface. The error bars show that there is significant spatial variation in moisture across the measured areas (both at depth and on the surface). Bete Giyorgis shows a slightly higher % increase in moisture compared to Bete Amanuel which is sheltered. At Bete Giyorgis there is a higher % increase at depth than the surface. Conversely at Bete Amanuel, the % increase between the surface and subsurface was comparable. This is likely due to the shelter over Bete Amanuel that plays a role in limiting the amount of moisture that enters the rock walls.
Figure 5. Percentage increase (% increase) in moisture content on the surface and subsurface of the zones measured at Bete Giyorgis (BG) and Bete Amanuel (BA). The error bars show that there is significant spatial variation in moisture across the measured areas (both at depth and on the surface) between dry and wet seasons.
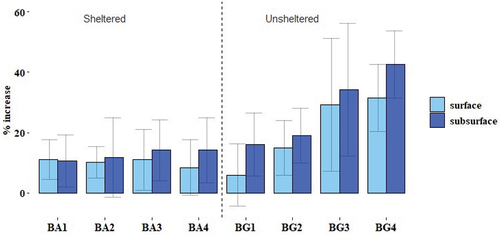
3.3. Spatial variation
Spatial variations of moisture content on the surface and at depth in both the wet and dry season are presented as heatmaps of saturation (%) in . Of the eight zones measured at Bete Amanuel and Bete Giyorgis in the wet and dry seasons, four representative areas zones BG1, BG4, BA1 & BA4 (whose locations are show in ) are presented for this discussion. The saturation (%) varies between the dry and wet seasons both in absolute terms (as shown in ) and spatial distribution (see ). During the dry season the surface is more saturated than the subsurface in both churches, as depicted by generally darker blue coloration in the surface vs subsurface heatmaps on the top rows within ). The surface probe of the MMMS measures moisture up to 3 cm depth, the reading is representative of the saturation (%) up to that depth. That is, the measurements are indicative of moisture present on and near the surface. Given the high rock-surface temperature and low relative humidity during the dry season at Lalibela, any moisture present on or near the surface is unlikely to be from capillary condensation. Rather, it is more likely that it is moisture transported near the surface by capillary uptake. Relatively low levels of moisture and inconsistent distribution in the interior of the rock wall during the dry season may reflect the disconnected hydraulic pathways typical of falling-rate drying.
Figure 6. An image of the measured zone and the surface and subsurface spatial distribution of saturation (%) are shown: (a) BG1, (b) BG4, (c) BA1 and (d) BA4. The axes on the heatmaps show the height and width (cm) of the measured areas.
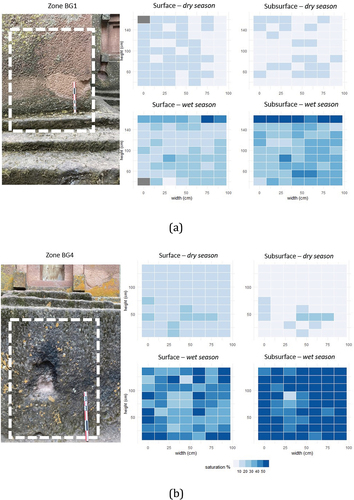
Conversely, in the wet season the walls are wetter at depth than on the surface, as depicted by generally darker blue coloration in the surface vs subsurface heatmaps in the bottom rows within ). The moisture content was higher at the base in Bete Amanuel as shown by the darker blue coloration in the lower parts of the wet season heatmaps in ). While in Bete Giyorgis hotspots of saturation are less uniformly distributed see random distribution of darker blue coloration in the wet season heatmaps in ). Areas on the surface that have high saturation (%) values have a corresponding, but higher, saturation (%) value at depth. This observation rules out moisture ingress from the surface through wind-driven rain or capillary condensation and indicates that connected hydraulic pathways are transporting moisture to the surface. The exposure of the roofs of Bete Giyorgis to direct rainfall contributes to deep wetness in the rock walls. Given the climatic condition in the wet season, that is, high humidity and low temperature, constant-rate drying is likely to occur.
4. Discussion
4.1. Advantages of the MMMS
This study reinforces findings from other studies (Gartner Citation2010; Kurik et al. Citation2017; Orr et al. Citation2020) that the MMMS can be usefully applied to cultural heritage settings to understand moisture dynamics. The calibration done in this study shows that the MMMS can be used on basaltic scoria. It also showed that this technique is particularly effective on rock-cut structures which are uniform in composition and therefore ideally suited for the MMMS, which is sensitive to variation in physical properties. Although directly interpreting the readings taken onsite is not straightforward, gravimetric calibration and representation of temporal and spatial variation in % allows for identification of key aspects of moisture dynamics, such as: (1) the range of moisture fluctuation over time and (2) surface and subsurface moisture distribution. Comparing measurements taken at different depths is not straightforward either, as the MI values of different MMMS sensors are not measured on directly comparable scales. Nonetheless, it is possible to overcome this limitation of the device by instead using % increase in moisture index values, as a proxy for temporal variation in moisture content. Gravimetric calibration () showed that the range of MI measurements (dry to saturated) for the surface and subsurface probes, and the slopes of their regression model were comparable. Therefore, the two probes can be used to compare change in moisture content at surface and in-depth and help to evaluate moisture gradients. Using the calibration function to convert MI values to saturation (%), it is possible to determine the surface to depth moisture gradient driven by seasonal fluctuation in environmental conditions.
4.2. Moisture regime in rock-cut structures
Rock-cut structures are highly exposed to the environment because they do not have roofs or foundations to retard the uptake of moisture. Lack of adequate drainage systems and breathable mortar joints means that these structures are more responsive to high seasonal variation in climate. At Lalibela, the wet and dry seasons have variable amounts of rainfall and very different diurnal thermal and humidity regimes. Due to the direct exposure of the roof to rain, the moisture content of the walls at Bete Giyorgis (unsheltered) rises significantly during the wet season (), especially at depth (up to 20–30 cm inside the wall). Low fluctuation in moisture content between the dry and wet seasons in the sheltered (Bete Amanuel) church demonstrates the role of a sheltering system in lowering moisture ingress in exposed rock-cut roof structures during intense rainy periods.
Based on the measurements presented in this paper, a conceptual model of moisture dynamics in rock-cut walls at Lalibela was developed (see . The conceptual model shows that the sources of moisture and the rate of evaporation differ between the seasons. The main source of moisture during the dry season is groundwater uptake through capillary rise. Because of low humidity, condensation of moisture in the micropores is unlikely to occur in the rock walls. Where as high near surface temperatures during the dry season are likely to lead to steep gradients in moisture content near the surface and drive the evaporation front to the subsurface. In the wet season, the moisture content is higher at depth than on the surface, this is due to a combination of rain infiltration, groundwater uptake, high humidity and low near surface temperature ().
Figure 7. Conceptual diagram of moisture transport and dynamics at rock-cut monolithic structures during wet and dry seasons.
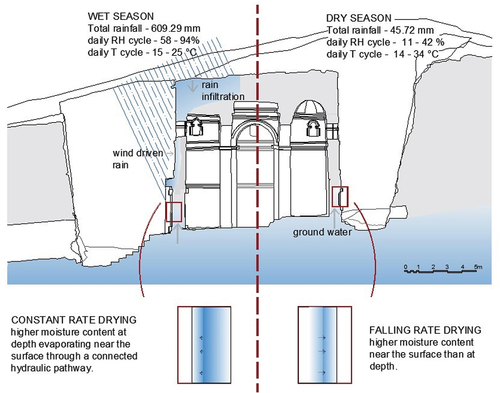
The conceptual model presented in can have wider application to other rock-cut structures and built stone structures (like Angkor Wat) in climatic zones that have intense rainy seasons, and which have directly exposed rock/stone roofs. These types of structures are more likely to experience deep wetting than those that have a sheltering system. Seepage of rainwater to the base of the temples at Angkor Wat has been implicated as the primary means of salt transport to the base of the temples (Siedel et al. Citation2010). Similarly, at the Yungang rock-cut grottoes in China the main source of moisture is the infiltration of rain through the roof (Huang Citation2007). Groundwater uptake has been noted as a significant conservation challenge at the Bingling rock-cut grottoes in China (Chen et al. Citation2019) and the rock-cut churches in Lalibela (Asrat and Ayallew Citation2011). Exposure of rock-cut roof structures and lack of impermeable foundations should be considered as a crucial factor that facilitates moisture related damage to rock-cut heritage sites.
4.3. Seasonal variation in moisture dynamics
Seasonal variation in rainfall and rock-surface temperatures will lead to a significant temporal and spatial variation in moisture within the walls. This, in turn, will lead to a seasonal variation in moisture dynamics, as has been demonstrated by the data presented in this paper. During the wet season, the two conditions that allow for a constant-rate drying to occur are met: (1) an increase in moisture at the surface and in-depth () and (2) a controlled rate of drying (low ambient and surface temperature) which allows for well-connected hydraulic pathways capable of sustaining a steady flow of moisture to the surface. The rise in groundwater level during the wet season will play a huge role as a source of water, even in the absence of rainfall. The lack of a semi-impermeable foundation to slow the rate of water transport at rock-cut sites will sustain the liquid pathway for constant-rate drying (). Conversely, during the dry season, the conditions for falling-rate drying are met. Unlike in the wet season, surface and subsurface hotspots of saturation (%) do not correspond () in the dry season. Due to high temperatures and limited sources of moisture in this season, it is unlikely that a hydraulic pathway that can sustain moisture flow matching the high evaporation rate will exist.
4.4. Implications of moisture dynamics for weathering at Lalibela
Long periods of sustained high flux moisture transport and deep wetness in the wet season (constant-rate drying), and sub-surface drying in the dry season (falling-rate drying) have implications for weathering of rock-cut churches at Lalibela. Higher saturation (%) at depth during the wet season suggests that deep wetness is likely to occur in the wet season, particularly in July and August which are characterised by heavy rainfall (Yimer et al. Citation2020). This can lead to the reduction of mechanical strength (Smith et al. Citation2011). High flux of moisture transport to the surface in the wet season will facilitate salt accumulation near the surface (efflorescence). In the dry season, the drying zone is in the subsurface which will lead to salt crystallisation below the surface (subflorescence). Given the significant presence of swelling clay minerals in the basaltic scoria (Asrat and Ayallew Citation2011), the surface-to-depth moisture gradients will be associated with stress due to tension and buckling (Gonzalez and Scherer Citation2004). The stress caused by wetting and drying and salt crystallisation cycles will be concentrated near the surface during the wet season and in the subsurface during the dry season, as a result of the seasonal variation in moisture dynamics. The combined effect of this, as seen from onsite observation, is granular disintegration of surface material and scaling (due to sub-efflorescence salts and clay swelling) in areas exposed to moisture ().
Comparative analysis of moisture distribution between the dry and wet seasons shows that moisture-related damage observed at Bete Giyorgis and Bete Amanuel is consistent with the rise and fall of the capillary rise at each church. The location of the granular disintegration, contour scaling and coving observed at Bete Giyorgis (30–90 cm) and Bete Amanuel (60–150 cm) corresponds well with the areas that showed a rise in moisture content during the wet season (). These figures may be the lower and upper limit of the rise and fall of the wetting fronts seasonally. Variations in the height of the capillary rise between the churches can be due to a difference in the groundwater table and thickness of the church walls. Similar to the finding in this study, Hall et al. (Citation2011), using their saturated and unsaturated flow models, have shown that the severe damage in El Merdani Mosque in Cairo was consistent with the seasonal variation in the rise and fall of the wetting front. Further investigation needs to be done to confirm this hypothesis.
5. Conclusions
This paper showed that moisture dynamics in the rock walls at Lalibela varies seasonally. As would be expected, the walls are wetter during the wet season than in the dry season. The moisture gradient from the surface to the depth was also different between the dry and wet seasons. During the dry season, there was a higher concentration of moisture near the surface than at depth. Conversely, in the wet season, the walls were more saturated at depth than near the surface. This suggests that constant-rate drying dominates in the wet season while falling-rate drying dominates in the dry season. The implications of the seasonally varying moisture dynamics for weathering are (1) fluctuation of drying to the surface and subsurface will cause salts to efflorescence or subfluorescence to occur and (2) stresses induced by clay swelling/shrinking will occur at different depths seasonally. Seasonally shifting moisture dynamics will contribute to a complex and dynamic damage profile.
This study showed that the MMMS can be applied to assess spatial and temporal variations in moisture content in basaltic scoria. Percentage increase (% increase) in moisture index can be used to assess temporal variation in moisture content. Gravimetric calibration was used in this study to determine the efficacy of the MMMS in measuring a range of unsaturated moisture contents (between oven dry and saturated) in basaltic scoria. The calibration function was used to convert field data to percent saturation (saturation %). This allowed the comparison of data collected with the surface (up to 2–3 cm) and subsurface (up to 20–30 cm) sensors as well as the assessment of surface-to-depth moisture gradients.
Heritage sites having a similar architectural style to rock-cut structures i.e., exposed rock/stone roofs and semi-permeable foundations are likely to experience similar moisture-related damage as shown in this paper. As far as the authors are aware, this is the first study to investigate seasonal moisture dynamics in rock-cut structures in Ethiopia. Given that over 200 rock-cut structures that are located in northern Ethiopia have similar rainfall patterns to Lalibela, the findings of this study have implications beyond the site studied in this chapter. Seasonality in moisture dynamics is also relevant for other rock-cut structures found in tropical climates such as Petra and Ellora.
This study also showed that protective open shelters at Lalibela are reducing the wetness of the walls during the wet season. A comparison of the sheltered (Bete Giyorgis) and unsheltered (Bete Amanuel) churches shows that the walls at Bete Giyorgis were more saturated during the wet season than at Bete Amanuel. Given the significance of the variation between the churches, it is reasonable to suggest that the shelter is playing a role in reducing the amount of moisture in the walls.
Acknowledgments
The authors would like to thank the Authority for Research and Conservation of Cultural Heritage (ARCCH), the North Wollo Culture and Tourism Office and Lalibela Church Administration office for their help in facilitating fieldwork activities. The authors would also like to thank the managers of the Ethio-French Sustainable Lalibela collaboration project for permission to use the orthophotos of Bete Amanuel and Bete Giyorgis.
Disclosure statement
No potential conflict of interest was reported by the author(s).
Additional information
Funding
References
- Asrat, A. 2002. The rock-hewn churches of Tigrai, Northern Ethiopia: A geological perspective. Geoarchaeology - An International Journal 17 (7):649–63. doi:10.1002/gea.10035.
- Asrat, A., and Y. Ayallew. 2011. Geological and geotechnical properties of the medieval rock hewn churches of Lalibela, Northern Ethiopia. Journal of African Earth Sciences 59 (1):61–73.
- Blaeuer, C., C. Science, C. Sarl, C. Science, and C. Sarl. 2009. Attempt to use a microwave moisture mapping system (MOIST 200B) to control and monitor the water uptake of stones in the frame of cultural heritage conservation. (May 2014).
- BSI. 2017. BS EN 16682:2017 conservation of cultural heritage — methods of measurement of moisture content, or water content, in materials constituting immovable cultural heritage.
- Chen, W., R. Liao, N. Wang, and J. Zhang. 2019. Effects of experimental frostthaw cycles on sandstones with different weathering degrees: A case from the bingling temple grottoes, China. Bulletin Of Engineering Geology And The Environment 78 (7):5311–26. doi: 10.1007/s10064-018-01454-2.
- Derat, M.-L., C. Bosc-tiess´e, A. Garric, R. Mensan, F.-X. Fauvelle, Y. Gleize, A.-L. Goujon, Y. Gleize, A.-L. Goujon, and A.-L. Goujon. 2021. The rock-cut churches of Lalibela and the cave church of Washa Mika ’ el: Troglodytism and the Christianisation of the Ethiopian Highlands. Anales de Pediatria 95 (July 2020):467–86. doi:10.1016/j.anpede.2020.11.009.
- Gartner, G. E. A. 2010. Determination of moisture content of the outer wall using hf-sensor technology. 302.
- Gobezie Worku, M. (2018). The Church of Yimrhane Kristos: An Archaeological Investigation (Doctoral dissertation). Lund University. Lund.
- Gonzalez, I. J., and G. W. Scherer. 2004. Effect of swelling inhibitors on the swelling and stress relaxation of clay bearing stones. Environmental Geology 46 (3–4):364–77. doi:10.1007/s00254-004-1038-8.
- Hall, K. 2004. Evidence for freeze–thaw events and their implications for rock weathering in northern Canada. Earth Surface Processes and Landforms 29 (1):43–57. doi:10.1002/esp.1012.
- Hall, C., A. Hamilton, W. D. Hoff, H. A. Viles, and J. A. Eklund. 2011. Moisture dynamics in walls: Response to micro-environment and climate change. Proceedings of the Royal Society A: Mathematical, Physical and Engineering Sciences 467 (2125):194–211. doi:10.1098/rspa.2010.0131.
- Hall, C., and W. Hoff. 2003. Water transport in brick, stone and concrete. Cement, Concrete and Aggregates 25 (1):11926. http://www.astm.org/doiLink.cgi?CCA10518J.
- hf sensor GmbH. 2014. Operation manual - moist 350 B ( tech. rep.). http://www.hf-sensor.de
- Huang, J. Z. 2007. Water-rock interaction and its effect on the weathering of Yungang Grottoes. In Preservation of Natural Stone and Rock Weathering. 1st ed., ed. P. Sola, J. Estaire, and C. Olalla, 33–38 14 July 2007. Preservation of Natural Stone and Rock Weathering, Madrid, Spain. https://www.routledge.com/Preservation-of-Natural-Stone-and-Rock-Weathering-Proceedings-of-the-ISRM/Sola-Estaire-Olalla/p/book/9780415450188
- ICOMOS. 2008. Illustrated glossary on stone deterioration patterns. France: Champigny/Marne. http://www.icomos.org/publications/monumentsandsites/15/pdf/MonumentsandSites15ISCSGlossaryStone.pdf.
- Kurik, L., T. Kalamees, U. Kallavus, and V. Sinivee. 2017. Influencing factors of moisture measurement when using microwave reflection method. Energy Procedia (June):11–14. doi:10.1016/j.egypro.2017.09.675.
- Lataste, J.-F., and A. Göller. 2018. Microwave reflection. doi:10.1007/978-3-319-74231-1_16.
- McAllister, D., P. Warke, S. McCabe, and M. Gomez-Heras. 2016. Evaporative moisture loss from heterogeneous stone: material-environment interactions during drying. Geomorphology 273:308–22 doi:10.1016/j.geomorph.2016.08.008. .
- McCabe, S., P. Brimblecombe, B. J. Smith, D. McAllister, S. Srinivasan, and P. A. Basheer. 2013. The use and meanings of ’time of wetness’ in understanding building stone decay. Quarterly Journal of Engineering Geology and Hydrogeology 46 (4):469–76. doi:10.1144/qjegh2012-048.
- Orr, S. A., L. Fusade, M. Young, D. Stelfox, A. Leslie, J. Curran, and H. Viles. 2020. Moisture monitoring of stone masonry: A comparison of microwave and radar on a granite wall and a sandstone tower. Journal of Cultural Heritage 41:61–73. doi:10.1016/j.culher.2019.07.011.
- Pinchin, S. E. 2008. Techniques for monitoring moisture in walls. Studies in Conservation 53 (sup2):33–45. doi:10.1179/sic.2008.53.supplement-2.33.
- Renzulli, A., F. Antonelli, C. Margottini, P. Santi, and F. Fratini. 2011. What kind of volcanite the rock-hewn churches of the Lalibela UNESCO’s world heritage site are made of? Journal of Cultural Heritage 12 (2):227–35 doi:10.1016/j.culher.2010.11.003. .
- Sass, O., and H. A. Viles. 2006. How wet are these walls? Testing a novel technique for measuring moisture in ruined walls. Journal of Cultural Heritage 7 (4):257–63. doi:10.1016/j.culher.2006.08.001.
- Sass, O., and H. A. Viles. 2010. Wetting and drying of masonry walls: 2D-resistivity monitoring of driving rain experiments on historic stonework in Oxford, UK. Journal of Applied Geophysics 70 (1):72–83 doi:10.1016/j.jappgeo.2009.11.006. .
- Sass, O., and H. Viles. 2022. Heritage hydrology: A conceptual framework for understanding water fluxes and storage in built and rock-hewn heritage. Heritage Science 10 (1):1–18. doi:10.1186/s40494-022-00693-7.
- Scherer, G. W. 1990. Theory of drying. Journal of the American Ceramic Society 73 (1):3–14. doi:10.1111/j.1151-2916.1990.tb05082.x.
- Siedel, H., S. Pfefferkorn, E. Von Plehwe-Leisen, and H. Leisen. 2010. Sandstone weathering in tropical climate: Results of low-destructive investigations at the temple of Angkor Wat, Cambodia. Engineering Geology 115 (3–4):182–92. doi:10.1016/j.enggeo.2009.07.003.
- Smith, B. J., S. Srinivasan, M. Gomez-Heras, P. A. M. Basheer, and H. A. Viles. 2011. Near-surface temperature cycling of stone and its implications for scales of surface deterioration. Geomorphology 130 (1–2):76–82 doi:10.1016/j.geomorph.2010.10.005. .
- Taye, B., L. Fusade, and H. Viles. 2020. Efficacy of traditional conservation methods for the protection of rock-hewn heritage sites: Lessons from Lalibela, Ethiopia. S. Siegesmund, and B. Middendorf, eds. Monument Future - Decay and Conservation of Stone proceedings of the 14th International Congress on the Deterioration and Conservaion of Stone (STONE 2020). Gottingen, Gemany, 7-12 September 2020. Halle, Mitteldeutscher Verlag.
- Woldeyes, Y. G. 2019. Lalibela: Spiritual genealogy beyond epistemic violence in Ethiopia. Genealogy 3 (4):66. doi:10.3390/genealogy3040066.
- Yimer, S. M., N. Kumar, A. Bouanani, B. Tischbein, and C. Borgemeister. 2020. Homogenization of daily time series climatological data in the Eastern Nile basin, Ethiopia. Theoretical and Applied Climatology 143 (1–2):737–60. doi:10.1007/s00704-020-03407-w.