ABSTRACT
CXCR4 is involved in many facets of cancer, including being a major player in establishing metastasis. This is in part due to the deregulation of CXCR4, which can be attributed to many genetic and epigenetic mechanisms, including aberrant microRNA–CXCR4 interaction. MicroRNAs (miRNAs) are a type of small non-coding RNA that primarily targets the 3’ UTR of mRNA transcripts, which in turn suppresses mRNA and subsequent protein expression. In this review, we reported and characterized the many aberrant miRNA–CXCR4 interactions that occur throughout human cancers. In particular, we reported known target sequences located on the 3’ UTR of CXCR4 transcripts that tumour suppressor miRNAs bind and therefore regulate expression by. From these aberrant interactions, we also documented affected downstream genes/pathways and whether a particular tumour suppressor miRNA was reported as a prognostic marker in its respected cancer type. In addition, a limited number of cancer-causing miRNAs coined ‘oncomirs’ were reported and described in relation to CXCR4 regulation. Moreover, the mechanisms underlying both tumour suppressor and oncomir deregulations concerning CXCR4 expression were also explored. Furthermore, the miR-146a-CXCR4 axis was delineated in oncoviral infected endothelial cells in the context of virus-causing cancers. Lastly, miRNA-driven therapies and CXCR4 antagonist drugs were discussed as potential future treatment options in reported cancers pertaining to deregulated miRNA–CXCR4 interactions.
Introduction
Chemokines are immunomodulatory cytokines (8–10 kDa peptides) involved in directional migration of immune cells and play important role in preserving the homoeostasis of the immune system (adaptive and innate immune system) [Citation1-3]. Chemokines are classified based on the position of first two cysteine residues into four families (two major types: CC and CXC and two minor types: C and CX3C chemokines) [Citation4,Citation5]. Normal immune function requires a balance between the differential and stably regulated expression of chemokines and its receptors. Imbalanced and aberrant expression of chemokines and chemokine receptors results in various immunological disorders, especially autoimmune diseases, and cancer [Citation6,Citation7]. In addition, chemokines are also utilized by tumour cells to actively progress and evade immunosurveillance. Chemokines control multistage carcinogenic process by affecting survival, proliferation, and metastatic spread of malignant cells as well as indirect modulation of neovascularization or angiogenesis [Citation8,Citation9] or in recruitment of immunosuppressive cells that cause escape from immunosurveillance [Citation10–13].
CXCR4, also known as Fusin/LESTR and cluster of differentiation-184 (CD-184) [Citation14,Citation15] is one of the best studied chemokine receptors because of its role as co-receptor for HIV entry [Citation16] and mediator of metastasis in a variety of cancers [Citation17]. Though CXCR4 expression is low or absent in many normal tissues, it is the most widely expressed chemokine receptor in almost 23 human cancers, including melanoma, ovary, breast, prostate, and colorectal cancer (CRC) [Citation1,Citation3,Citation18]. CXCR4 is a 352 amino acid chemokine receptor and functions as rhodopsin-like G protein-coupled receptor (GPCR) [Citation5,Citation19]. CXCR4 can exist as monomer, dimer, oligomer, or nanoclusters in the plasma membrane [Citation20]. It has N-terminus outside the cell and C-terminus in the cytoplasm. Moreover, there are three extracellular and three intracellular loops with seven transmembrane helices [Citation21]. While chemokine receptors can bind multiple ligands, CXCR4, selectively binds CXC chemokine stromal cell factor 1 (SDF-1) also referred to as CXCL12 [Citation19,Citation22]. However, it can also bind to cytokine MIF (macrophage migration inhibitory factor) as well as ubiquitin [Citation23,Citation24]. CXCR4-CXCL12 is the best identified axis involved in homing and retention of haematopoietic stem cells in the bone marrow [Citation25] and involved in tumour growth, invasion, angiogenesis, and metastasis in various cancer types [Citation26–28]. Binding of CXCR4 to CXCL12 activates multiple G protein-dependent (AKT, PI3K, mTOR and EGFR) as well as G protein-independent signalling pathways (JAK/STAT, p53 MAPK and ERK) [Citation29–31], resulting in downstream cellular phenotypic effects that are pertinent to several essential biological processes including haematopoiesis, gestational development, vascularization, and organogenesis [Citation32–37]. Moreover, CXCR4 deregulated overexpression frequently associates with promoting cell migration [Citation38–45], proliferation [Citation31,Citation43,Citation46–48], invasion [Citation43,Citation49–53], inflammation [Citation45], angiogenesis [Citation44], and metastasis [Citation3,Citation42,Citation54–57] in numerous cancer types. CXCR4 expression is also altered in human oncovirus-infected progenitor blood cells. For example, CXCR4 overexpression is found in HIV+ patients and functions as a coreceptor for HIV and therefore promotes viral integration in haematopoietic stem cells (HSC), which serve as reservoirs of latent HIV [Citation58]. CXCR4 is regulated by several different biological mechanisms including transcription factor binding, G protein signalling, and various epigenetic processes. In the context of T-cell-tropic HIV-1 entry, a bimodal pattern of CXCR4 expression is controlled through promoter binding of basal transcription factors Nuclear Respiratory Factor-1 (NRF-1) as a positive regulator and Ying Yang (YY1) as a negative regulator [Citation59,Citation60]. In addition, intracellular second messengers also regulate transcriptional control of CXCR4. For instance, second messengers like cyclic AMP [Citation61], calcium (Ca2+) [Citation59], transmembrane proteins CD3 and CD28 [Citation59], and several cytokine interleukins promote CXCR4 expression [Citation59, Citation62–64], while cytokines related to inflammation IL-1β, INF-γ, and TNF-α reduce CXCR4 expression [Citation63–66]. Interestingly, G protein signalling can be initiated in the absence of ligand-bound induced CXCR4 activation. Alternatively, it has been shown that CXCR4 can form homodimers in T-cells and potentially illicit a downstream signal, which may have implications associated with HIV-1 entry [Citation67].
Aberrant epigenetic modifications also affect CXCR4 expression in cancer. For example, CXCR4 promoter hyper/hypo methylation deregulates CXCR4 expression observed in several cancers and contributes to metastasis. For instance, prior studies have linked increased CXCR4 promoter 5-methylcytosine (5mC) to decreased CXCR4 protein expression in primary breast cancer [Citation54], melanoma cells [Citation68], and primary cervical cancer [Citation69]. On the other hand, decreased promoter methylation and CXCR4 overexpression is an individual prognostic factor associated with a poorer outcome in patients with glioblastoma multiforme [Citation70].
First identified in C. elegans in the early 1990s [Citation71], microRNAs (miRNAs) are small oligonucleotides that function as an epigenetic regulator that plays a vital role in post-transcriptional gene regulation as it pertains to essential biological processes, but are commonly deregulated in cancer, which can lead to tumourigenesis. Extraordinarily, miRNAs are a type of small non-coding RNA ranging between only 21–22 nucleotides in length by which they negatively regulate genes through imperfect complementary binding of the 3'UTR sequence on mRNA transcripts [Citation72]. It is well known that miRNAs primarily play an instructive role in cell development and differentiation. Quintessentially, ectopic overexpression of miRNAs miR-9 and miR-124 in human fibroblasts leads to the activation of neurogenic related pathways that drastically change the cellular morphology of fibroblasts, which are then ultimately converted into neuronal cells that exhibit neuronal functions and may therefore have future therapeutic implications in treating neurological diseases [Citation73]. In cancer, many miRNAs are deregulated, therefore compromising their ability to target and dismantle oncogenic mRNA transcripts like CXCR4. In addition, some miRNAs can enhance oncogenic expression and are typically upregulated in cancer.
There are several reviews in the past focusing on clinical role of chemokines in cancer [Citation74], regulation of CXCR4 signalling [Citation30] or role of CXCR4 signalling in cell proliferation, invasion, and metastasis of cancer [Citation29,Citation75–77], and mechanism of miRNAs action in cancers [Citation78,Citation79]. However, none of them are focused on miRNA interactions with CXCR4, particularly in cancers. This is the first review, where we summarized the current knowledge pertaining to deregulated miRNA-CXCR4 targeted interaction in the context of human cancers and explored the utility of miRNAs as a potential therapeutic drug in targeting CXCR4 in cancer.
Characterization of tumour suppressor miRNAs and their clinical significance in deregulating CXCR4 in cancer
In cancer, tumour suppressor miRNA loss of function or repression can disable their ability to degrade oncogenic mRNA through 3'UTR-miRNA targeted interaction and therefore perturbs their ability to regulate genes involved in molecular processes like cell proliferation, differentiation, and apoptosis. For example, numerous studies have reported that decreased expression of tumour suppressor miRNA-let-7 leads to unregulated protein overexpression of its target oncogene RAS and therefore contributes to the tumour phenotype observed in lung cancer [Citation80,Citation81].
Given CXCR4's established reputation as an oncogene augmented with metastatic capabilities, it comes to no surprise that the majority of aberrantly expressed miRNAs targeting CXCR4 are downregulated, enabling overexpression of CXCR4. Thus, currently, the majority of the reported miRNAs that interact with CXCR4 in cancer function as tumour suppressors in their respective cell types. A total of 24 tumour suppressor miRNAs targeting CXCR4 are downregulated in various cancers ranging from solid tumours like colon cancer: miR-126 [Citation82–85], miR-133b [Citation86], miR-193a-5p [Citation87], miR-622 [Citation88] and breast cancer: miR-146a [Citation89], miR-302a [Citation90], miR-381[Citation91], miR-494 [Citation92] to blood cancers such as leukaemia of either myeloid: miR-146a [Citation93–95], miR-335 [Citation93], miR-622 [Citation93], miR-9 [Citation96] or lymphoid: miR-139 [Citation97] of origin ().
Table 1. Deregulated tumour suppressor (n = 24) or oncogenic (n = 3) miRNAs identified as CXCR4 targets in human cancers with corresponding downstream target genes and pathways.
Indeed, many of the deregulated tumour suppressor miRNAs targeting CXCR4 in cancer hold prognostic value in terms of predicting patient outcome. These include miR-126, miR-133b in colorectal cancer [Citation84,Citation86]; miR-139 in gastric cancer, T-cell acute lymphoblastic leukaemia, and laryngeal squamous cell carcinoma [Citation97,Citation106,Citation107]; miR-622 in hepatocellular carcinoma [Citation108]; miR-494-3p, miR-338-3p in prostate cancer [Citation109,Citation110]; miR-143 in oral squamous cell cancer [Citation111]; miR-494 in pancreatic cancer [Citation112]; miR-9 in head and neck squamous cell and nasopharyngeal carcinoma [Citation113,Citation114]; and miR-663 in glioblastoma [Citation115]. In particular, deregulated miR-494-CXCR4 interactions have been reported in a myriad of cancers. Examples include breast, prostate, pancreatic, and synovial cancers, in which tumour suppressor miR-494 is downregulated, leaving CXCR4 unchecked and overexpressed [Citation92,Citation110,Citation112,Citation116]. Although, it is important to note that like many other miRNAs, miR-494 can act as an oncogene in other forms of cancer. For example, in hepatocellular carcinoma and colorectal cancers, miR-494 is upregulated and functions as an oncogene by targeting and subsequently downregulating important tumour suppressors like PTEN, CDKN1B, PUMA and APC [Citation117,Citation118]. Other notable underexpressed miRNAs targeting CXCR4 include miR-9, which lacks tumour suppressor function in several forms of head/neck-related cancers including oral squamous cell and nasopharyngeal carcinomas, emphasizing the importance of the miR-9-CXCR4 axis as a potential therapeutic target in head and neck cancers [Citation113,Citation114,Citation119].
Tumour suppressor microRNAs targeting CXCR4 are downregulated in cancer via epigenetic regulation
Little is known of the mechanism(s) contributing to tumour suppressor miRNA underexpression themselves, which can result in CXCR4 overexpression in many cancer forms. Currently, two general processes of miRNA underexpression in CXCR4-targeted regulation have been described. The first epigenetic mechanism reflects a fairly recent hypothesis of competitive endogenous RNAs (ceRNAs) that function as a microRNA ‘sponge’ through RNA–RNA mediated interaction leading to subsequent miRNA degradation and therefore inhibiting the miRNA-3'UTR interactivity of its oncogenic target [Citation120]. For example, tumour suppressors miR-206 and miR-494 are neutralized by overexpressed circular RNAs circ_0056618 and circ-UBAP2 that function as ceRNAs in gastric and pancreatic cancers, respectively [Citation112,Citation121]. Therefore, the counter activity imposed by ceRNAs on miR-206 and miR-494 leads to CXCR4 upregulation and subsequent tumourigenesis. Similarly, lncRNA growth arrest-specific transcript 5 (GAS5) is overexpressed in oesophageal cancer cells and behaves like a ceRNA by sponging CXCR4 targeted miR-301a transcripts [Citation122]. Interestingly, in the case of oesophageal cancer, Li et al. reported that CXCR4 itself functions as a tumour suppressor, where induced overexpression of CXCR4 promotes apoptosis. The authors demonstrated that miR-301a functions as a positive regulator of CXCR4. Therefore, similar to [miR-206 and miR-494], miR-301a also functions as a tumour suppressor, but instead as a positive regulator of CXCR4.
The second epigenetic mechanism in CXCR4 driven cancers describes a decrease of tumour suppressor miRNA function by the means of promoter hypermethylation coupled with histone modifications that leads to an inactive (silenced) heterochromatin conformational state of miRNAs. For instance, the promoter of tumour suppressor miR-9 is hypermethylated and silenced in nasopharyngeal carcinomas, while the promoter of miR-622 is hypermethylated and transcriptionally repressed by EZH2-induced post-translational histone modification H3K27 trimethylation in hepatocellular carcinoma [Citation108,Citation114]. In addition, miR-139 undergoes histone deacetylation, thus impeding its tumour-suppressor function in gastric cancer [Citation106]. One could therefore hypothesize that at least some of the unknown mechanisms downregulating the remaining tumour suppressor miRNAs targeting CXCR4, consists of some form of RNA–RNA mediated interaction or downregulation by DNA hypermethylation or modifications of amino acid residues on histone tails, residing in the promoter region. For this reason, epigenetic-targeted therapies may apply to deregulated miRNA-CXCR4 related cancers.
CXCR4 is deregulated by overexpressed oncomirs in cancer
Overexpressed miRNAs in cancers are typically categorized as oncogenes, coined ‘oncomirs,’ which are less well described than tumour suppressor miRNAs. Oncomirs contribute to tumour development by either negatively regulating tumour suppressor genes through miRNA–3'UTR interaction and subsequent mRNA degradation or by positively regulating target oncogenes through mechanisms not completely understood. Interestingly, clusters of oncomirs can exist as a single polycistronic transcript within the human genome. For instance, the existence of the miR-17-92 miRNA cluster in the human genome has been well described in cancer and is the first to be identified in human developmental diseases [Citation123]. The miR-17-92 cluster is comprised of 7 miRNAs: miR-17-5p, miR-17-3p, miR-18a, miR-19a, miR-19b-1, miR-20a, miR-92-1 that play multifaceted roles essential to normal embryonic and skeletal development [Citation123]. Indeed, deletion of the cluster was found to be perinatal lethal in mice, emphasizing its importance in mammalian development. As an oncomir, overexpression of the miR-17-92 cluster is a common feature observed in lymphoma and lung cancer [Citation123,Citation124]. In addition, their molecular interplay and subsequent downregulation of notable tumour suppressor PTEN and antiapoptotic regulator BIM was shown to increase lymphocyte hyper-proliferation and autoimmune diseases in mice [Citation125,Citation126].
A mere total of 5 miRNAs were documented as oncomirs targeting CXCR4 in various cancer types () [Citation127–131]. In the case of colorectal cancer, osteosarcoma, melanoma cells, breast cancer, and non-small cell lung cancer: miR-125b, miR-301a, and miR-199a-5p, Let-7 f, miR-410, respectively, are upregulated and function as oncomirs by positively regulating CXCR4 and thereby promoting tumourigenesis and metastasis [Citation127–131]. For example, one of the mechanisms contributing to miR-125b upregulation in colorectal cancer cells includes an established positive feedback loop leading to increased CXCR4 expression and ultimately triggering epithelial–mesenchymal transition (EMT) and cell invasion. In modest detail, activation of the (SDF-1/CXCL12)/CXCR4 axis induces miR-125b expression in colorectal cancer cells, which in turn enhances CXCR4 expression, thus completing the positive feedback loop [Citation129]. In another example, non-coding RNA gene UCA1 is speculated to positively regulate the expression of miR-301a in some manner leading to CXCR4 upregulation as demonstrated in UCA1 knockdown studies in osteosarcoma cells. However, the mechanistic underpinnings describing oncomir driven upregulation of CXCR4 and other oncogenes in general are lacking and require further study. A recent novel mechanism reported by Place et al. describes miR-373 positively regulating the expression of its target genes through complementary binding of promoter sequences and thereby inducing transcription, thus expression [Citation132]. Future studies analysing oncomir interactions in the promoter regions of CXCR4 are therefore warranted.
CXCR4 is targeted by miR-146a in oncovirus infected endothelial cells
In addition to tumour cells, the miRNA-CXCR4 axis is altered in oncoviral infected endothelial cells carrying, for example, the human herpesvirus 8 (HHV8) observed in Kaposis sarcoma or the Epstein–Barr virus (EBV) involved in lymphomas like Burkitt lymphoma and Hodgkin/non-Hodgkin's lymphomas [Citation133,Citation134]. Endothelial cells harbouring oncoviruses HHV8 and EBV produce viral proteins which directly bind and upregulate their miRNA target, which in turn downregulates CXCR4. For example, miR-146a is upregulated by viral protein LMP1 and functions like a tumour suppressor in EBV+ human endothelial cells by targeting CXCR4, which inhibits cell growth and promotes apoptosis [Citation134]. Paradoxically, Wang et al. suggests that the biological effects of CXCR4 targeting by miR-146a results in protecting the oncovirus in latently infected EBV+ endothelial cells by decreasing arrest of the G0/G1 phase cell growth. Therefore, the LMP1-miR-146a-CXCR4 axis serves an important function in EBV+ endothelial cells by delicately shifting between regulating cell growth and evading anti-tumour immune responses.
In HHV8 latent infected endothelial cells, viral protein K13 induces miR-146a expression, which in turn targets and downregulates CXCR4. Punj et al. hypothesizes that CXCR4 downregulation may result in premature release of progenitor endothelial cells infected with HHV8 into circulation, considering increased CXCR4 surface expression is a marker of developing immature endothelial cells that are maintained in the bone marrow [Citation133]. In summary, the miR-146a-CXCR4 axis plays a crucial role in oncoviral infected endothelial cells and may therefore serve as an important therapeutic target in the future.
MicroRNA ‘mimics’ as a therapeutic approach in targeting CXCR4 in cancer
Given that RNA transcripts vastly outnumber protein-coding genes, the potential use of small RNA drugs in a clinical setting remains largely untapped [Citation111,Citation135]. The future use of miRNA-targeted therapy may hold a unique advantage in terms of eliminating chemo-resistant cancer cells. Indeed, miRNAs are pleiotropic in nature, meaning several hundred or even thousands of gene transcripts may be targeted. This notion implies that entire deregulated biological pathways may be targeted in cancer [Citation136]. However, the potential unwanted off-target biological effects remains a potential issue. Although, ectopic expression of miRNA ‘mimics’ are typically designed to conserve most of their endogenous counterpart miRNAs sequence with the intent to mitigate off-targeting.
Countless studies have demonstrated the potential therapeutic utility of overexpressing tumour suppressor miRNA ‘mimics’ to target and downregulate CXCR4 and thereby decreasing migration, invasion, and/or proliferation of cancer cells. For instance, ectopic overexpression of the entire miR-302-367 cluster suppressed the stemness and tumourigenic properties of glioblastoma cells, in part by targeted downregulating CXCR4 [Citation137]. The same research team again utilized glioma cells to express the miR-302-367 cluster and determined that glioma cells expel the miRNA cluster via exosome secretion, which are then internalized by neighbouring glioma cells [Citation138]. Indeed, their glioblastoma in vivo model demonstrated that brain tumour development was inhibited in rats treated with miR-302-367 compared to untreated controls. Therefore, miRNA ‘mimics’ remain a viable option in targeting CXCR4 and potentially a broad span of other oncogenes involved in cancer.
CXCR4 antagonists as a therapeutic approach to restore endogenous expression of CXCR4 targeted miRNAs in cancer
Administration of CXCR4 antagonist not only successfully inhibited CXCR4 at the protein level, but the reported downstream effects included restoration of miRNA tumour suppressor or anti-arthritic function. Previously, we have discussed the use of CXCR4-CXCL12 axis in the therapy of Gastrointestinal cancers both in pre-clinical and clinical trials and its limitations [Citation139]. Multiple antagonists of CXCR4 (bicyclams and T22) and peptide analogues designed for the N-terminal of CXCL12 (TN14003, CTCE-9908 and ALX40-4C) are in use to target CXCR4 in multiple cancers [Citation140–143]. AMD3100 (plerixafor or mozobil) is the most frequently used CXCR4 antagonist drug in clinical trials targeting the CXCR4-CXCL12 axis [Citation144]. Immunotherapy targeting of the CXCR4-CXCL12 axis has provided encouraging results in ovarian cancer [Citation145], multiple myeloma [Citation146], acute myeloid leukaemia [Citation147], and cervical cancer [Citation148] as well as relapsed or refractory multiple myeloma [Citation149].
Targeted therapies involving CXCR4-CXCL12 axis in combination with silencing or inhibiting expression of oncogenes by small interfering RNA (siRNA) and microRNA (miR) could be another approach for cancer therapy [Citation150]. SiRNA-based drugs patisiran [Citation151], givosiran [Citation152], and lumasiran [Citation153] are approved by FDA for phase 3 clinical trials. However, only a few miRNA-based therapeutics have been in phase 1 or 2 clinical trials, and most of them have been discontinued because of off-target effects resulting in adverse events/death [Citation154,Citation155].
CXCR4 antagonist drug TN14003 upregulated CXCR4 target miR-146a in leukaemic cells [Citation156]. A similar effect was also seen in colorectal cancer cells upon antimicrobial peptide (AMP) treatment, where CXCR4 was downregulated and its tumour suppressor miRNA target, miR-663a was upregulated and therefore functionally revived [Citation157]. Taken together, the prior miRNA-driven studies suggest a bright future for miRNA-mediated therapy as an additional treatment option in cancer. Future miRNA-driven therapies may therefore include overexpressing tumour suppressor miRNAs that target CXCR4 transcripts. Moreover, CXCR4 antagonist drugs not only inhibited CXCR4 protein function, but also alleviated previously stifled tumour suppressor miRNA function.
Conclusion
This is the first review where we have identified and characterized many deregulated miRNAs that target CXCR4 in various cancers, where most of these miRNAs serve as tumour suppressors in their respective cell types. In addition to tumour suppressor miRNAs, but to a much lesser extent, oncomirs targeting CXCR4 were also identified and their complex and not fully understood mechanisms described. Furthermore, the miR-146a-CXCR4 axis was explored in oncoviral infected endothelial cells. Recent evidence suggests that there are many undiscovered miRNAs, potentially thousands [Citation158]. Therefore, it is highly likely that CXCR4 is regulated by many other miRNAs that would presumably succumb to deviant expression, which may then deregulate CXCR4 in cancer. For instance, using the microRNA prediction software tool ‘TargetScanHuman 7.2’ and searching for broadly conserved microRNAs that target CXCR4 in humans, we found 4 miRNAs, 3 of which were already identified as tumour suppressors in this review: miR-140, miR-1, and miR-9 () [Citation159]. Intriguingly, miR-655 has not been investigated as a potential target of CXCR4 in any biological context and may be worth exploring, given its tumour suppressor role in many cancers [Citation160–162]. Furthermore, identifying miR-CXCR4 complements may hold the key to unlocking novel therapies in cancer, which may include tumour suppressor miRNA ‘mimics’ and other miR-driven forms of treatment.
Figure 1. UCSC Genome Browser (hg19 build) depiction of 4 conserved putative microRNA binding sites within the 3’ UTR (gold bar) of CXCR4 (blue and black bars) as predicted by in silico analysis using TargetScanHuman 7.2. Site type prediction: Red miR = exact match to positions 2–8 of the mature miRNA, Purple miR = an exact match to positions 2–8 of the mature miRNA followed by an ‘A.’
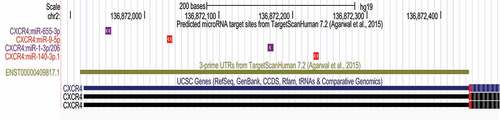
Key highlights
CXCR4 transcripts are targeted by tumour suppressor microRNAs and oncomirs in cancer.
The CXCR4-miRNA axis plays an important role in oncoviral function within the cell.
MiRNA ‘mimics’ and CXCR4 targeted antagonist therapeutics help in combating tumour progression.
Data availability
was created using the UCSC Genome Browser.
Disclosure statement
No potential conflict of interest was reported by the author(s).
Additional information
Funding
References
- Balkwill F. Cancer and the chemokine network. Nat Rev Cancer. 2004;4(7):540–550.
- Moser B, Loetscher P. Lymphocyte traffic control by chemokines. Nat Immunol. 2001;2(2):123–128.
- Muller A, Homey B, Soto H, et al. Involvement of chemokine receptors in breast cancer metastasis. Nature. 2001;410(6824):50–56.
- Bacon K, Baggiolini M, Broxmeyer H, et al. Chemokine/chemokine receptor nomenclature. J Interferon Cytokine Res. 2002;22(10):1067–1068.
- Zlotnik A, Yoshie O. Chemokines: a new classification system and their role in immunity. Immunity. 2000;12(2):121–127.
- Oppenheim JJ, Yang D, Biragyn A, et al. Chemokine receptors on dendritic cells promote autoimmune reactions. Arthritis Res. 2002;4(3):S183–188.
- Ploix C, Lo D, Carson MJ. A ligand for the chemokine receptor CCR7 can influence the homeostatic proliferation of CD4 T cells and progression of autoimmunity. J Immunol. 2001;167(12):6724–6730.
- Strieter RM, Polverini PJ, Kunkel SL, et al. The functional role of the ELR motif in CXC chemokine-mediated angiogenesis. J Biol Chem. 1995;270(45):27348–27357.
- Varney ML, Johansson SL, Singh RK. Tumour-associated macrophage infiltration, neovascularization and aggressiveness in malignant melanoma: role of monocyte chemotactic protein-1 and vascular endothelial growth factor-A. Melanoma Res. 2005;15(5):417–425.
- Fushimi T, Kojima A, Moore MA, et al. Macrophage inflammatory protein 3alpha transgene attracts dendritic cells to established murine tumors and suppresses tumor growth. J Clin Invest. 2000;105(10):1383–1393.
- Kumamoto T, Huang EK, Paek HJ, et al. Induction of tumor-specific protective immunity by in situ Langerhans cell vaccine. Nat Biotechnol. 2002;20(1):64–69.
- Mendez R, Ruiz-Cabello F, Rodriguez T, et al. Identification of different tumor escape mechanisms in several metastases from a melanoma patient undergoing immunotherapy. Cancer Immunol Immunother. 2007;56(1):88–94.
- Nukiwa M, Andarini S, Zaini J, et al. Dendritic cells modified to express fractalkine/CX3CL1 in the treatment of preexisting tumors. Eur J Immunol. 2006;36(4):1019–1027.
- Burger M, Glodek A, Hartmann T, et al. Functional expression of CXCR4 (CD184) on small-cell lung cancer cells mediates migration, integrin activation, and adhesion to stromal cells. Oncogene. 2003;22(50):8093–8101.
- Tachibana K, Nakajima T, Sato A, et al. CXCR4/fusin is not a species-specific barrier in murine cells for HIV-1 entry. J Exp Med. 1997;185(10):1865–1870.
- Feng Y, Broder CC, Kennedy PE, et al. HIV-1 entry cofactor: functional cDNA cloning of a seven-transmembrane, G protein-coupled receptor. Science. 1996;272(5263):872–877.
- Zlotnik A. Involvement of chemokine receptors in organ-specific metastasis. Contrib Microbiol. 2006;13:191–199.
- Scotton CJ, Wilson JL, Milliken D, et al. Epithelial cancer cell migration: a role for chemokine receptors? Cancer Res. 2001;61(13):4961–4965.
- Murphy PM, Baggiolini M, Charo IF, et al. International union of pharmacology. XXII. Nomenclature for chemokine receptors. Pharmacol Rev. 2000;52(1):145–176.
- Martinez-Munoz L, Rodriguez-Frade JM, Barroso R, et al. Separating actin-dependent chemokine receptor nanoclustering from dimerization indicates a role for clustering in CXCR4 signaling and function. Mol Cell. 2018;70(1):106–119 e110.
- Wu B, Chien EY, Mol CD, et al. Structures of the CXCR4 chemokine GPCR with small-molecule and cyclic peptide antagonists. Science. 2010;330(6007):1066–1071.
- Fredriksson R, Lagerstrom MC, Lundin LG, et al. The G-protein-coupled receptors in the human genome form five main families. Phylogenetic analysis, paralogon groups, and fingerprints. Mol Pharmacol. 2003;63(6):1256–1272.
- Bernhagen J, Krohn R, Lue H, et al. MIF is a noncognate ligand of CXC chemokine receptors in inflammatory and atherogenic cell recruitment. Nat Med. 2007;13(5):587–596.
- Saini V, Marchese A, Majetschak M. CXC chemokine receptor 4 is a cell surface receptor for extracellular ubiquitin. J Biol Chem. 2010;285(20):15566–15576.
- Moll NM, Ransohoff RM. CXCL12 and CXCR4 in bone marrow physiology. Expert Rev Hematol. 2010;3(3):315–322.
- Li LN, Jiang KT, Tan P, et al. Prognosis and clinicopathology of CXCR4 in colorectal cancer patients: a meta-analysis. Asian Pac J Cancer Prev. 2015;16(9):4077–4080.
- Luker KE, Luker GD. Functions of CXCL12 and CXCR4 in breast cancer. Cancer Lett. 2006;238(1):30–41.
- Sleightholm RL, Neilsen BK, Li J, et al. Emerging roles of the CXCL12/CXCR4 axis in pancreatic cancer progression and therapy. Pharmacol Ther. 2017;179:158–170.
- Bianchi ME, Mezzapelle R. The chemokine receptor CXCR4 in cell proliferation and tissue regeneration. Front Immunol. 2020;11:2109.
- Busillo JM, Benovic JL. Regulation of CXCR4 signaling. Biochim Biophys Acta. 2007;1768(4):952–963.
- Zuo J, Wen M, Li S, et al. Overexpression of CXCR4 promotes invasion and migration of non-small cell lung cancer via EGFR and MMP-9. Oncol Lett. 2017;14(6):7513–7521.
- Ma Q, Jones D, Borghesani PR, et al. Impaired B-lymphopoiesis, myelopoiesis, and derailed cerebellar neuron migration in CXCR4- and SDF-1-deficient mice. Proc Natl Acad Sci U S A. 1998;95(16):9448–9453.
- McGrath KE, Koniski AD, Maltby KM, et al. Embryonic expression and function of the chemokine SDF-1 and its receptor, CXCR4. Dev Biol. 1999;213(2):442–456.
- Nagasawa T, Hirota S, Tachibana K, et al. Defects of B-cell lymphopoiesis and bone-marrow myelopoiesis in mice lacking the CXC chemokine PBSF/SDF-1. Nature. 1996;382(6592):635–638.
- Nagasawa T, Tachibana K, Kishimoto T. A novel CXC chemokine PBSF/SDF-1 and its receptor CXCR4: their functions in development, hematopoiesis and HIV infection. Semin Immunol. 1998;10(3):179–185.
- Tachibana K, Hirota S, Iizasa H, et al. The chemokine receptor CXCR4 is essential for vascularization of the gastrointestinal tract. Nature. 1998;393(6685):591–594.
- Zou YR, Kottmann AH, Kuroda M, et al. Function of the chemokine receptor CXCR4 in haematopoiesis and in cerebellar development. Nature. 1998;393(6685):595–599.
- Arai J, Yasukawa M, Yakushijin Y, et al. Stromal cells in lymph nodes attract B-lymphoma cells via production of stromal cell-derived factor-1. Eur J Haematol. 2000;64(5):323–332.
- Gu F, Ma Y, Zhang J, et al. Function of Slit/Robo signaling in breast cancer. Front Med. 2015;9(4):431–436.
- Hwang JH, Hwang JH, Chung HK, et al. CXC chemokine receptor 4 expression and function in human anaplastic thyroid cancer cells. J Clin Endocrinol Metab. 2003;88(1):408–416.
- Jin Z, Zhao C, Han X, et al. Wnt5a promotes Ewing sarcoma cell migration through upregulating CXCR4 expression. BMC Cancer. 2012;12(1):480.
- Murakami T, Maki W, Cardones AR, et al. Expression of CXC chemokine receptor-4 enhances the pulmonary metastatic potential of murine B16 melanoma cells. Cancer Res. 2002;62(24):7328–7334.
- Orimo A, Gupta PB, Sgroi DC, et al. Stromal fibroblasts present in invasive human breast carcinomas promote tumor growth and angiogenesis through elevated SDF-1/CXCL12 secretion. Cell. 2005;121(3):335–348.
- Rempel SA, Dudas S, Ge S, et al. Identification and localization of the cytokine SDF1 and its receptor, CXC chemokine receptor 4, to regions of necrosis and angiogenesis in human glioblastoma. Clin Cancer Res. 2000;6(1):102–111.
- Yu X, Wang D, Wang X, et al. CXCL12/CXCR4 promotes inflammation-driven colorectal cancer progression through activation of RhoA signaling by sponging miR-133a-3p. J Exp Clin Cancer Res. 2019;38(1):32.
- Eck SM, Cote AL, Winkelman WD, et al. CXCR4 and matrix metalloproteinase-1 are elevated in breast carcinoma-associated fibroblasts and in normal mammary fibroblasts exposed to factors secreted by breast cancer cells. Mol Cancer Res. 2009;7(7):1033–1044.
- Ottaiano A, Di Palma A, Napolitano M, et al. Inhibitory effects of anti-CXCR4 antibodies on human colon cancer cells. Cancer Immunol Immunother. 2005;54(8):781–791.
- Thomas RM, Kim J, Revelo-Penafiel MP, et al. The chemokine receptor CXCR4 is expressed in pancreatic intraepithelial neoplasia. Gut. 2008;57(11):1555–1560.
- Bertolini F, Dell’Agnola C, Mancuso P, et al. CXCR4 neutralization, a novel therapeutic approach for non-Hodgkin’s lymphoma. Cancer Res. 2002;62(11):3106–3112.
- Corcione A, Ottonello L, Tortolina G, et al. Stromal cell-derived factor-1 as a chemoattractant for follicular center lymphoma B cells. J Natl Cancer Inst. 2000;92(8):628–635.
- Koshiba T, Hosotani R, Miyamoto Y, et al. Expression of stromal cell-derived factor 1 and CXCR4 ligand receptor system in pancreatic cancer: a possible role for tumor progression. Clin Cancer Res. 2000;6(9):3530–3535.
- Robledo MM, Bartolome RA, Longo N, et al. Expression of functional chemokine receptors CXCR3 and CXCR4 on human melanoma cells. J Biol Chem. 2001;276(48):45098–45105.
- Scotton CJ, Wilson JL, Scott K, et al. Multiple actions of the chemokine CXCL12 on epithelial tumor cells in human ovarian cancer. Cancer Res. 2002;62(20):5930–5938.
- Alevizos L, Kataki A, Derventzi A, et al. Breast cancer nodal metastasis correlates with tumour and lymph node methylation profiles of Caveolin-1 and CXCR4. Clin Exp Metastasis. 2014;31(5):511–520.
- Geminder H, Sagi-Assif O, Goldberg L, et al. A possible role for CXCR4 and its ligand, the CXC chemokine stromal cell-derived factor-1, in the development of bone marrow metastases in neuroblastoma. J Immunol. 2001;167(8):4747–4757.
- Libura J, Drukala J, Majka M, et al. CXCR4-SDF-1 signaling is active in rhabdomyosarcoma cells and regulates locomotion, chemotaxis, and adhesion. Blood. 2002;100(7):2597–2606.
- Murakami T, Kawada K, Iwamoto M, et al. The role of CXCR3 and CXCR4 in colorectal cancer metastasis. Int J Cancer. 2013;132(2):276–287.
- Wegner SA, Ehrenberg PK, Chang G, et al. Genomic organization and functional characterization of the chemokine receptor CXCR4, a major entry co-receptor for human immunodeficiency virus type 1. J Biol Chem. 1998;273(8):4754–4760.
- Moriuchi M, Moriuchi H, Margolis DM, et al. USF/c-Myc enhances, while Yin-Yang 1 suppresses, the promoter activity of CXCR4, a coreceptor for HIV-1 entry. J Immunol. 1999;162(10):5986–5992.
- Moriuchi M, Moriuchi H, Turner W, et al. Cloning and analysis of the promoter region of CXCR4, a coreceptor for HIV-1 entry. J Immunol. 1997;159(9):4322–4329.
- Cristillo AD, Highbarger HC, Dewar RL, et al. Up-regulation of HIV coreceptor CXCR4 expression in human T lymphocytes is mediated in part by a cAMP-responsive element. FASEB J. 2002;16(3):354–364.
- Jourdan P, Vendrell JP, Huguet MF, et al. Cytokines and cell surface molecules independently induce CXCR4 expression on CD4+ CCR7+ human memory T cells. J Immunol. 2000;165(2):716–724.
- Murdoch C, Monk PN, Finn A. Cxc chemokine receptor expression on human endothelial cells. Cytokine. 1999;11(9):704–712.
- Wang J, Guan E, Roderiquez G, et al. Role of tyrosine phosphorylation in ligand-independent sequestration of CXCR4 in human primary monocytes-macrophages. J Biol Chem. 2001;276(52):49236–49243.
- Feil C, Augustin HG. Endothelial cells differentially express functional CXC-chemokine receptor-4 (CXCR-4/fusin) under the control of autocrine activity and exogenous cytokines. Biochem Biophys Res Commun. 1998;247(1):38–45.
- Han Y, Wang J, He T, et al. TNF-alpha down-regulates CXCR4 expression in primary murine astrocytes. Brain Res. 2001;888(1):1–10.
- Babcock GJ, Farzan M, Sodroski J. Ligand-independent dimerization of CXCR4, a principal HIV-1 coreceptor. J Biol Chem. 2003;278(5):3378–3385.
- Mori T, Kim J, Yamano T, et al. Epigenetic up-regulation of C-C chemokine receptor 7 and C-X-C chemokine receptor 4 expression in melanoma cells. Cancer Res. 2005;65(5):1800–1807.
- Yadav SS, Prasad SB, Das M, et al. Epigenetic silencing of CXCR4 promotes loss of cell adhesion in cervical cancer. Biomed Res Int. 2014;2014:581403.
- Ma X, Shang F, Zhu W, et al. CXCR4 expression varies significantly among different subtypes of glioblastoma multiforme (GBM) and its low expression or hypermethylation might predict favorable overall survival. Expert Rev Neurother. 2017;17(9):941–946.
- Lee RC, Feinbaum RL, Ambros V. The C. elegans heterochronic gene lin-4 encodes small RNAs with antisense complementarity to lin-14. Cell. 1993;75(5):843–854.
- Lai EC. Micro RNAs are complementary to 3’ UTR sequence motifs that mediate negative post-transcriptional regulation. Nat Genet. 2002;30(4):363–364.
- Yoo AS, Sun AX, Li L, et al. MicroRNA-mediated conversion of human fibroblasts to neurons. Nature. 2011;476(7359):228–231.
- Dell’Agnola C, Biragyn A. Clinical utilization of chemokines to combat cancer: the double-edged sword. Expert Rev Vaccines. 2007;6(2):267–283.
- Cojoc M, Peitzsch C, Trautmann F, et al. Emerging targets in cancer management: role of the CXCL12/CXCR4 axis. Onco Targets Ther. 2013;6:1347–1361.
- Luker GD, Yang J, Richmond A, et al. At the bench: pre-clinical evidence for multiple functions of CXCR4 in cancer. J Leukoc Biol. 2021;109(5):969–989.
- Portella L, Bello AM, Scala S. CXCL12 signaling in the tumor microenvironment. Adv Exp Med Biol. 2021;1302:51–70.
- Peng Y, Croce CM. The role of microRNAs in human cancer. Signal Transduct Target Ther. 2016;1(1):15004.
- Si W, Shen J, Zheng H, et al. The role and mechanisms of action of microRNAs in cancer drug resistance. Clin Epigenetics. 2019;11(1):25.
- Johnson CD, Esquela-Kerscher A, Stefani G, et al. The let-7 microRNA represses cell proliferation pathways in human cells. Cancer Res. 2007;67(16):7713–7722.
- Johnson SM, Grosshans H, Shingara J, et al. RAS is regulated by the let-7 microRNA family. Cell. 2005;120(5):635–647.
- Li Z, Li N, Wu M, et al. Expression of miR-126 suppresses migration and invasion of colon cancer cells by targeting CXCR4. Mol Cell Biochem. 2013;381(1–2):233–242.
- Liu Y, Zhou Y, Feng X, et al. MicroRNA-126 functions as a tumor suppressor in colorectal cancer cells by targeting CXCR4 via the AKT and ERK1/2 signaling pathways. Int J Oncol. 2014;44(1):203–210.
- Liu Y, Zhou Y, Feng X, et al. Low expression of microRNA-126 is associated with poor prognosis in colorectal cancer. Genes Chromosomes Cancer. 2014;53(4):358–365.
- Yuan W, Guo YQ, Li XY, et al. MicroRNA-126 inhibits colon cancer cell proliferation and invasion by targeting the chemokine (C-X-C motif) receptor 4 and Ras homolog gene family, member A, signaling pathway. Oncotarget. 2016;7(37):60230–60244.
- Duan FT, Qian F, Fang K, et al. miR-133b, a muscle-specific microRNA, is a novel prognostic marker that participates in the progression of human colorectal cancer via regulation of CXCR4 expression. Mol Cancer. 2013;12(1):164.
- Shirafkan N, Shomali N, Kazemi T, et al. microRNA-193a-5p inhibits migration of human HT-29 colon cancer cells via suppression of metastasis pathway. J Cell Biochem. 2019;5:8775–8783.
- Fang Y, Sun B, Wang J, et al. miR-622 inhibits angiogenesis by suppressing the CXCR4-VEGFA axis in colorectal cancer. Gene. 2019;699:37–42.
- Zheng T, Chou J, Zhang F, et al. CXCR4 3'UTR functions as a ceRNA in promoting metastasis, proliferation and survival of MCF-7 cells by regulating miR-146a activity. Eur J Cell Biol. 2015;94(10):458–469.
- Liang Z, Bian X, Shim H. Inhibition of breast cancer metastasis with microRNA-302a by downregulation of CXCR4 expression. Breast Cancer Res Treat. 2014;146(3):535–542.
- Xue Y, Xu W, Zhao W, et al. miR-381 inhibited breast cancer cells proliferation, epithelial-to-mesenchymal transition and metastasis by targeting CXCR4. Biomed Pharmacother. 2017;86:426–433.
- Song L, Liu D, Wang B, et al. miR-494 suppresses the progression of breast cancer in vitro by targeting CXCR4 through the Wnt/beta-catenin signaling pathway. Oncol Rep. 2015;34(1):525–531.
- Alfano D, Gorrasi A, Li Santi A, et al. Urokinase receptor and CXCR4 are regulated by common microRNAs in leukaemia cells. J Cell Mol Med. 2015;19(9):2262–2272.
- Spinello I, Quaranta MT, Paolillo R, et al. Differential hypoxic regulation of the microRNA-146a/CXCR4 pathway in normal and leukemic monocytic cells: impact on response to chemotherapy. Haematologica. 2015;100(9):1160–1171.
- Spinello I, Quaranta MT, Riccioni R, et al. MicroRNA-146a and AMD3100, two ways to control CXCR4 expression in acute myeloid leukemias. Blood Cancer J. 2011;1(6):e26.
- Zhu B, Xi X, Liu Q, et al. MiR-9 functions as a tumor suppressor in acute myeloid leukemia by targeting CX chemokine receptor 4. Am J Transl Res. 2019;11(6):3384–3397.
- Qin L, Deng HY, Chen SJ, et al. miR-139 acts as a tumor suppressor in T-cell acute lymphoblastic leukemia by targeting CX chemokine receptor 4. Am J Transl Res. 2017;9(9):4059–4070.
- Feng JA. Target research on tumor biology characteristics of mir-155-5p regulation on gastric cancer cell. Pak J Pharm Sci. 2016;29(2 Suppl):711–718.
- He B, He Y, Shi W, et al. Bioinformatics analysis of gene expression alterations in microRNA122 knockout mice with hepatocellular carcinoma. Mol Med Rep. 2017;15(6):3681–3689.
- Leone V, D’Angelo D, Rubio I, et al. MiR-1 is a tumor suppressor in thyroid carcinogenesis targeting CCND2, CXCR4, and SDF-1alpha. J Clin Endocrinol Metab. 2011;96(9):E1388–1398.
- Qian Y, Wang X, Lv Z, et al. MicroRNA126 is downregulated in thyroid cancer cells, and regulates proliferation, migration and invasion by targeting CXCR4. Mol Med Rep. 2016;14(1):453–459.
- Zhu Y, Tang L, Zhao S, et al. CXCR4-mediated osteosarcoma growth and pulmonary metastasis is suppressed by microRNA-613. Cancer Sci. 2018;109(8):2412–2422.
- Zhang M, Zhang L, Cui M, et al. miR-302b inhibits cancer-related inflammation by targeting ERBB4, IRF2 and CXCR4 in esophageal cancer. Oncotarget. 2017;8(30):49053–49063.
- Yao C, Huang S, Wu J, et al. MicroRNA-140 inhibits tumor progression in nasopharyngeal carcinoma by targeting CXCR4. Int J Clin Exp Pathol. 2017;10(7):7750–7759.
- Xu X, Cao L, Zhang Y, et al. MicroRNA-1246 inhibits cell invasion and epithelial mesenchymal transition process by targeting CXCR4 in lung cancer cells. Cancer Biomark. 2018;21(2):251–260.
- Bao W, Fu HJ, Xie QS, et al. HER2 interacts with CD44 to up-regulate CXCR4 via epigenetic silencing of microRNA-139 in gastric cancer cells. Gastroenterology. 2011;141(6):2076–2087. e2076.
- Luo HN, Wang ZH, Sheng Y, et al. MiR-139 targets CXCR4 and inhibits the proliferation and metastasis of laryngeal squamous carcinoma cells. Med Oncol. 2014;31(1):789.
- Liu H, Liu Y, Liu W, et al. EZH2-mediated loss of miR-622 determines CXCR4 activation in hepatocellular carcinoma. Nat Commun. 2015;6(1):8494.
- Bakkar A, Alshalalfa M, Petersen LF, et al. microRNA 338-3p exhibits tumor suppressor role and its down-regulation is associated with adverse clinical outcome in prostate cancer patients. Mol Biol Rep. 2016;43(4):229–240.
- Shen PF, Chen XQ, Liao YC, et al. MicroRNA-494-3p targets CXCR4 to suppress the proliferation, invasion, and migration of prostate cancer. Prostate. 2014;74(7):756–767.
- Mesgarzadeh AH, Aali M, Farhadi F, et al. Transfection of microRNA-143 mimic could inhibit migration of HN-5 cells through down-regulating of metastatic genes. Gene. 2019;716:144033.
- Zhao R, Ni J, Lu S, et al. CircUBAP2-mediated competing endogenous RNA network modulates tumorigenesis in pancreatic adenocarcinoma. Aging (Albany NY). 2019;11(19):8484–8501.
- Hersi HM, Raulf N, Gaken J, et al. MicroRNA-9 inhibits growth and invasion of head and neck cancer cells and is a predictive biomarker of response to plerixafor, an inhibitor of its target CXCR4. Mol Oncol. 2018;12(12):2023–2041.
- Lu J, Luo H, Liu X, et al. miR-9 targets CXCR4 and functions as a potential tumor suppressor in nasopharyngeal carcinoma. Carcinogenesis. 2014;35(3):554–563.
- Shi Y, Chen C, Yu SZ, et al. miR-663 suppresses oncogenic function of CXCR4 in glioblastoma. Clin Cancer Res. 2015;21(17):4004–4013.
- Pazzaglia L, Pollino S, Vitale M, et al. miR494.3p expression in synovial sarcoma: role of CXCR4 as a potential target gene. Int J Oncol. 2019;54(1):361–369.
- Pollutri D, Patrizi C, Marinelli S, et al. The epigenetically regulated miR-494 associates with stem-cell phenotype and induces sorafenib resistance in hepatocellular carcinoma. Cell Death Dis. 2018;9(1):4.
- Zhang Y, Guo L, Li Y, et al. MicroRNA-494 promotes cancer progression and targets adenomatous polyposis coli in colorectal cancer. Mol Cancer. 2018;17(1):1.
- Yu T, Liu K, Wu Y, et al. MicroRNA-9 inhibits the proliferation of oral squamous cell carcinoma cells by suppressing expression of CXCR4 via the Wnt/beta-catenin signaling pathway. Oncogene. 2014;33(42):5017–5027.
- Salmena L, Poliseno L, Tay Y, et al. A ceRNA hypothesis: the Rosetta stone of a hidden RNA language? Cell. 2011;146(3):353–358.
- Li H, Yao G, Feng B, et al. Circ_0056618 and CXCR4 act as competing endogenous in gastric cancer by regulating miR-206. J Cell Biochem. 2018;119(11):9543–9551.
- Li W, Zhao W, Lu Z, et al. Long noncoding RNA GAS5 promotes proliferation, migration, and invasion by regulation of miR-301a in esophageal cancer. Oncol Res. 2018;26(8):1285–1294.
- Zhang ZW, An Y, Teng CB. [The roles of miR-17-92 cluster in mammal development and tumorigenesis]. Yi Chuan. 2009;31(11):1094–1100.
- Osada H, Takahashi T. let-7 and miR-17-92: small-sized major players in lung cancer development. Cancer Sci. 2011;102(1):9–17.
- Ohyashiki M, Ohyashiki JH, Hirota A, et al. Age-related decrease of miRNA-92a levels in human CD8+ T-cells correlates with a reduction of naive T lymphocytes. Immun Ageing. 2011;8(1):11.
- Ventura A, Young AG, Winslow MM, et al. Targeted deletion reveals essential and overlapping functions of the miR-17 through 92 family of miRNA clusters. Cell. 2008;132(5):875–886.
- Egea V, Kessenbrock K, Lawson D, et al. Let-7f miRNA regulates SDF-1alpha- and hypoxia-promoted migration of mesenchymal stem cells and attenuates mammary tumor growth upon exosomal release. Cell Death Dis. 2021;12(6):516.
- Ke X, Yuan Y, Guo C, et al. MiR-410 induces stemness by inhibiting Gsk3beta but upregulating beta-catenin in non-small cells lung cancer. Oncotarget. 2017;8(7):11356–11371.
- Yu X, Shi W, Zhang Y, et al. CXCL12/CXCR4 axis induced miR-125b promotes invasion and confers 5-fluorouracil resistance through enhancing autophagy in colorectal cancer. Sci Rep. 2017;7(1):42226.
- Zhou J, Liu R, Wang Y, et al. miR-199a-5p regulates the expression of metastasis-associated genes in B16F10 melanoma cells. Int J Clin Exp Pathol. 2014;7(10):7182–7190.
- Zhu G, Liu X, Su Y, et al. Knockdown of urothelial carcinoma-associated 1 suppressed cell growth and migration through regulating miR-301a and CXCR4 in osteosarcoma MHCC97 cells. Oncol Res. 2018;27(1):55–64.
- Place RF, Li LC, Pookot D, et al. MicroRNA-373 induces expression of genes with complementary promoter sequences. Proc Natl Acad Sci U S A. 2008;105(5):1608–1613.
- Punj V, Matta H, Schamus S, et al. Kaposi’s sarcoma-associated herpesvirus-encoded viral FLICE inhibitory protein (vFLIP) K13 suppresses CXCR4 expression by upregulating miR-146a. Oncogene. 2010;29(12):1835–1844.
- Wang W, Zhang Y, Liu W, et al. LMP1-miR-146a-CXCR4 axis regulates cell proliferation, apoptosis and metastasis. Virus Res. 2019;270:197654.
- International Human Genome Sequencing Consortium. International Human Genome Sequencing C: finishing the euchromatic sequence of the human genome. Nature. 2004;431(7011):931–945.
- Bartel DP. MicroRNAs: target recognition and regulatory functions. Cell. 2009;136(2):215–233.
- Fareh M, Turchi L, Virolle V, et al. The miR 302-367 cluster drastically affects self-renewal and infiltration properties of glioma-initiating cells through CXCR4 repression and consequent disruption of the SHH-GLI-NANOG network. Cell Death Differ. 2012;19(2):232–244.
- Fareh M, Almairac F, Turchi L, et al. Cell-based therapy using miR-302-367 expressing cells represses glioblastoma growth. Cell Death Dis. 2017;8(3):e2713.
- Khare T, Bissonnette M, Khare S. CXCL12-CXCR4/CXCR7 axis in colorectal cancer: therapeutic target in preclinical and clinical studies. Int J Mol Sci. 2021;22(14):7371.
- Donahue RE, Jin P, Bonifacino AC, et al. Plerixafor (AMD3100) and granulocyte colony-stimulating factor (G-CSF) mobilize different CD34+ cell populations based on global gene and microRNA expression signatures. Blood. 2009;114(12):2530–2541.
- Liang Z, Yoon Y, Votaw J, et al. Silencing of CXCR4 blocks breast cancer metastasis. Cancer Res. 2005;65(3):967–971.
- Porvasnik S, Sakamoto N, Kusmartsev S, et al. Effects of CXCR4 antagonist CTCE-9908 on prostate tumor growth. Prostate. 2009;69(13):1460–1469.
- Wong D, Korz W. Translating an antagonist of chemokine receptor CXCR4: from bench to bedside. Clin Cancer Res. 2008;14(24):7975–7980.
- Daniel SK, Seo YD, Pillarisetty VG. The CXCL12-CXCR4/CXCR7 axis as a mechanism of immune resistance in gastrointestinal malignancies. Semin Cancer Biol. 2020;65:176–188.
- Righi E, Kashiwagi S, Yuan J, et al. CXCL12/CXCR4 blockade induces multimodal antitumor effects that prolong survival in an immunocompetent mouse model of ovarian cancer. Cancer Res. 2011;71(16):5522–5534.
- De Clercq E. Mozobil(R) (Plerixafor, AMD3100), 10 years after its approval by the US food and drug administration. Antivir Chem Chemother. 2019;27:2040206619829382.
- Uy GL, Rettig MP, Motabi IH, et al. A phase 1/2 study of chemosensitization with the CXCR4 antagonist plerixafor in relapsed or refractory acute myeloid leukemia. Blood. 2012;119(17):3917–3924.
- Chaudary N, Pintilie M, Jelveh S, et al. Plerixafor improves primary tumor response and reduces metastases in cervical cancer treated with radio-chemotherapy. Clin Cancer Res. 2017;23(5):1242–1249.
- Ghobrial IM, Liu CJ, Zavidij O, et al. Phase I/II trial of the CXCR4 inhibitor plerixafor in combination with bortezomib as a chemosensitization strategy in relapsed/refractory multiple myeloma. Am J Hematol. 2019;94(11):1244–1253.
- Fire A, Xu S, Montgomery MK, et al. Potent and specific genetic interference by double-stranded RNA in Caenorhabditis elegans. Nature. 1998;391(6669):806–811.
- Mullard A. FDA drug approvals. Nat Rev Drug Discov. 2019;18(2):85–89.
- Mullard A. 2019 FDA drug approvals. Nat Rev Drug Discov. 2020;19(2):79–84.
- Mullard A. FDA drug approvals. Nat Rev Drug Discov. 2021;20(2):85–90
- Grimaldi AM, Salvatore M, Incoronato M. miRNA-based therapeutics in breast cancer: a systematic review. Front Oncol. 2021;11:668464.
- Zhang S, Cheng Z, Wang Y, et al. The risks of miRNA therapeutics: in a drug target perspective. Drug Des Devel Ther. 2021;65:721–733.
- Jia D, Li Y, Han R, et al. miR146a5p expression is upregulated by the CXCR4 antagonist TN14003 and attenuates SDF1induced cartilage degradation. Mol Med Rep. 2019;19(5):4388–4400.
- Kuroda K, Fukuda T, Krstic-Demonacos M, et al. miR-663a regulates growth of colon cancer cells, after administration of antimicrobial peptides, by targeting CXCR4-p21 pathway. BMC Cancer. 2017;17(1):33.
- Friedlander MR, Lizano E, Houben AJ, et al. Evidence for the biogenesis of more than 1,000 novel human microRNAs. Genome Biol. 2014;15(4):R57.
- Agarwal V, Bell GW, Nam JW, et al. Predicting effective microRNA target sites in mammalian mRNAs. Elife. 2015;12:4.
- Liang HQ, Wang RJ, Diao CF, et al. The PTTG1-targeting miRNAs miR-329, miR-300, miR-381, and miR-655 inhibit pituitary tumor cell tumorigenesis and are involved in a p53/PTTG1 regulation feedback loop. Oncotarget. 2015;6(30):29413–29427.
- Wang Q, Lv L, Li Y, et al. MicroRNA655 suppresses cell proliferation and invasion in oral squamous cell carcinoma by directly targeting metadherin and regulating the PTEN/AKT pathway. Mol Med Rep. 2018;18(3):3106–3114.
- Wang W, Cao R, Su W, et al. miR-655-3p inhibits cell migration and invasion by targeting pituitary tumor-transforming 1 in non-small cell lung cancer. Biosci Biotechnol Biochem. 2019;83(9):1703–1708.