ABSTRACT
When plants are infected by pathogens two distinct responses can occur, the early being a local response in the infected area, and later a systemic response in non-infected tissues. Closure of stomata has recently been found to be a local response to bacterial pathogens. Stomata closure is linked to both salicylic acid (SA), an essential hormone in local responses and systemic acquired resistance (SAR), and absisic acid (ABA) a key regulator of drought and other abiotic stresses. SAR reduces the effects of later infections. In this review we discuss recent research elucidating the role of guard cells in local and systemic immune responses, guard cell interactions with abiotic and hormone signals, as well as putative functions and interactions between long-distance SAR signals.
Introduction
Phytopathogens cause numerous diseases that can lead to decreases in crop yield, economic losses, and they threaten global food security and natural resources.Citation1 Because plants do not have the mobile immune cells that vertebrates have, the plant immune system has been thought to be far less complex and has been less studied. However, plants are capable of highly-specific immune responses.Citation2–Citation4 In addition, recent studies suggest that plants can generate a lifelong “memory” of pathogen interaction, referred to as “priming”, and that they pass the immunity to their offspring through epigenetic changes. In the “primed” state, plants maintain an enhanced ability to activate cellular defense responses quickly and effectively.Citation5,Citation6
The particular plant–pathogen interaction that is the focus of this review is between Arabidopsis thaliana (a reference plant with rich genetic resources) and the bacterium Pseudomonas syringae. During infection, the pathogen secretes coronatine, a molecule that mimics the plant hormone jasmonate-isoleucine and enables bypass of plant immune defenses.Citation7–Citation10 Stomata, which are pores formed by pairs of guard cells on the surfaces of leaves that function in gas exchange and transpiration, respond to pathogen infectionCitation11–Citation13 as well as environmental cues including light, carbon dioxide, and humidity.Citation14–Citation18
Although there are some general aspects to plant immunity that are well characterized, plant-pathogen responses vary greatly from one species to another, and there are still a lot of gaps in our knowledge. For example, local responses to certain pathogens have been well characterized, but there is still a need for information in long distance signaling, how the signals confer immunity, how the immunized tissues respond, and how this is passed on to future generations.Citation19–Citation21 Additionally, recent reviews of plant immunity have not focused on the important role that guard cells play in the initial localized response to pathogen attack, and research on the systemic response that confers immunity has not included the potential role of guard cells.Citation2,Citation4 Recent research found that the plant hormone salicylic acid (SA), which regulates immune responses in systemic acquired resistance (SAR), can also regulate stomatal closure through the cell-specific transcription factor NTM1-LIKE 9 (NTL9).Citation22,Citation23 Therefore, in addition to serving as a primary defense against local pathogen attack, primed guard cells in systemic tissues may play additional roles in preventing future infections as a part of the overall plant immunity.
Local responses to pathogens: PAMP, PTI, and ETI
The site where the pathogen enters a plant organ is referred to as the primary infection site, and the molecular responses in the infected tissues are local responses. Phytopathogens encounter many obstacles when attacking a plant cell, the first being the plant cell wall. Upon activation of defense pathways, the cell wall is further reinforced by deposition of callose (a glucan polymer) at the site of pathogen attack. The plant’s first line of the defense against the invading pathogens are receptors localized in the plant cell membrane that recognize pathogen-associated molecular patterns (PAMPs) or microorganism-associated molecular patterns (MAMPs). These PAMPs (or MAMPs) are conserved, microbial-specific molecules from the pathogen and include lipopolysaccharides, flagellin, and chitin. One type of pattern recognition receptor (PRR) has a conserved structure consisting of an extracellular leucine-rich repeat (LRR) domain and an intracellular protein kinase domain. Several PRRs have been characterized () and include transmembrane EF-Tu receptor (EFR) and Flagellin Sensitive 2 (FLS2). FLS2 is analogous in structure to human Toll-like receptor 5 (TLR5), and it recognizes the pathogen elicitor flg22, a peptide corresponding to the conserved domain of flagellinCitation2 A. thaliana also has receptor-like kinases (RLKs) and receptor-like cytoplasmic kinases (RLCKs) that mediate transmembrane signal transduction as part of plant immunity.Citation25 One such RLK, brassinosteroid insensitive 1 (BRI1)-associated receptor kinase 1 (BAK1) associates with FLS2 which associates with BOTRYTIS-INDUCED KINASE1 (BIK1), a RLCK that functions downstream of BAK1 to activate the respiratory burst oxidase homolog D (RBOH D) and trigger reactive oxygen species (ROS) burst.Citation25 This initial recognition of pathogen attack together with the basal defense response triggered by the PRRs is referred to as PAMP triggered immunity (PTI). PTI is characterized by several molecular and physiological changes in the infected cell including alkalinization, changes in ion flux, increase in ROS and nitric oxide (NO), and activation of mitogen-activated protein kinase (MAPK) cascades.Citation26–Citation29 The overall effect of PTI is to curtail pathogen growth.
Figure 1. Stomatal guard cell response to Pseudomonas syringae. Guard cells play an early role in plant immunity and respond to the pathogen P. syringae in a number of ways. Aperture of the stomatal pore is modulated by turgor pressure of the surrounding guard cells, and the solute potential is controlled by anion channels and K+ channels. During stomatal closure, these channels are regulated by a series of signaling events initiated by perception of the pathogen by the guard cell. PAMPs (or MAMPs), like flg22, released from the pathogen are recognized by transmembrane receptors such as FLS2 and BAK1. BAK1 associates FLS2 which in turn associates with BIK1 to activate RBOH, and initiate ROS burst. MPK3 and MPK6, as well as RBOH, NO, and ROS burst are downstream effects of FLS2 recognition of flg22. PAMP perception also triggers Ca2+ channels leading to intercellular Ca2+ increase and CDPK activation. The overall effect of PTI and ETI is to stop the spread of the pathogen. While generation of ROS signals play an important role, various plant hormones including SA, JA, and ABA also play a role in this stomatal response. Some evidence shows that SA-induced stomatal closure is linked to NO and the NTL9 transcription factor which controls SA biosynthesis in this process. Increase in SA activates NPR1, a key regulator of SAR signaling. Increased levels of endogenous ABA during the biotic stress inhibit H+ extrusion, activate Ca2+ uptake channels, and also activate both slow ‘S-type’ and rapid ‘R-type’ anions efflux channels. These changes lead to K+ efflux via the voltage-gated outward K+ channel GORK and membrane depolarization.Citation24 These K+ and anion changes increase water potential in the guard cells and drive water to move out, causing stomatal closure. ABA also activates stomatal closure via the activation of the serine-threonine protein kinase OST1. Phosphorylation by OST1 activates SLAC1 anion channels and inhibits the KAT1 K+ inward rectifying channels. Ions move out and water potential in the guard cells is increased, water moves out and turgor pressure decreases, resulting in closure of the stomatal pore. During pathogen-induced stomatal opening, the pathogen effector COR structurally mimics the plant hormone JA-Ile and binds to COI1 to trigger the degradation of JAZ proteins. Additionally, COR induces NAC transcription factors that bind MYC2 and repress SA synthesis leading to reopening of stomata.
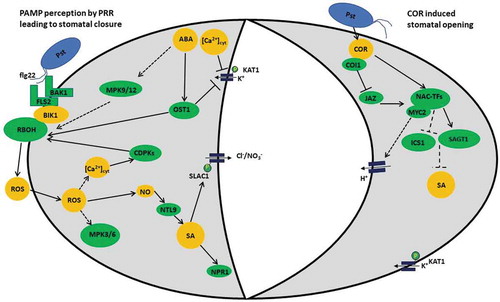
Certain pathogens, including bacterial pathogens with type-III secretion pili and some fungal pathogens, have developed ways to suppress PTI. Pathogenic bacteria in the genera of Pseudomonas, Ralstonia, Xanthomonas, and Erwinia can suppress the primary PTI defense of the plant via injection of strain-specific effector molecules into the plant cell.Citation30–Citation32 However, plants have a second level of immune response, called effector-triggered immunity or ETI, which developed through co-evolution with specific pathogensCitation33 When effectors are recognized by intracellular nucleotide-binding (NB)-LRR receptors, ETI is triggered. As part of ETI, plants have intracellular receptors known as resistance (R) proteins that detect the presence of specific effector proteins known as avirulence (Avr) or type-three effectors (T3E). When Avr proteins are recognized by the plant’s R proteins, a type of localized programmed cell death (PCD) called hypersensitive responses (HR) results in restriction of the pathogen spread. Additionally, interactions of Avr effectors with R proteins lead to the expression of pathogenesis related (PR) genes, which are tightly linked to SARCitation33 that increases the resistance of distal tissues to infection. The beneficial effects of SARCitation34 are enhanced resistance to a broad spectrum of pathogens in non-infected parts of the plant.Citation4 However, the HR and local senescence are not required for SAR, as evidenced by recent research with P. syringae maculicola.Citation35 Acronyms related to plant immune response are summarized in .
Table 1. Acronyms and abbreviations related to plant immune response. Acronyms and abbreviations for factors involved in plant immunity that are mentioned in this review are listed with their meanings and a brief summary of their functions. These factors include both local and systemic plant immune responses.
Role of guard cells in perception of pathogen attack
Guard cells are dynamic specialized cells that respond to many different environmental cues, allowing them to close the stomata in unfavorable conditions and then open the stomata when the environment is favorable. Guard cells have the unique ability to integrate multiple, diverse, and at times conflicting signals in response to light, photoperiod, abiotic stress, and biotic stress and translate them into appropriate physiological and physical responses.Citation14,Citation16,Citation17,Citation36
Although it was previously assumed that these surface openings were merely passive portals of entry for foliar plant pathogens, recent research has shown that guard cells are pathogen responsive and play an early role in the plant immune response.Citation7,Citation11,Citation12 Guard cell perception of PAMPs leads to a cell-specific response, and stomata closure is triggered. When leaves or epidermal peels of A. thaliana were exposed to a suspension of P. syringae pv tomato (Pst) DC3000, a marked reduction in the number of open stomata (from circa 70–80% to 30%) was observed within 1–2 h of incubation, and the average stomatal aperture was reduced from approximately 2 µm to 1 µm.Citation11 Perception of PAMPs by plant cell PRRs triggers calcium channel activation in guard cells, increasing Ca,2+ which then activates calcium-dependent protein kinases (CDPK). CDPK then activates a plasma membrane localized NADPH oxidases (RBOHs) and induces extracellular ROS production. CDPK also activates anion channels leading to membrane depolarization, which in turn activates outwardly-rectifying K+ channels and K+ efflux. This decreases guard cell turgor and leads to stomatal closure. Stomatal closure is also promoted by the phytohormones SA, jasmonic acid (JA), and abscisic acid (ABA). Interestingly, these phytohormones also regulate pathogen responses in SAR, which will be discussed later. Playing a central role as a common regulator of many of these guard cell signal transduction pathways is OPEN STOMATA 1 (OST1, aka SnRK 2.6), a serine-threonine protein kinase that initiates downstream signaling.Citation37,Citation38 OST1 was identified by Mustilli et al. using infrared thermal imaging to screen a population of ethyl methane sulfonate-mutagenized Arabidopsis for mutants with lower leaf temperatures.Citation38 Mutations in OST1 prevent stomatal closure in the presence of ABA.Citation38 OST1 phosphorylates a number of substrates including the RBOH and slow anion channel-associated 1 (SLAC1) S-type anion channel, which is activated by OST1. Another substrate, K+ inward rectifying channels in A. thaliana (KAT1) are inhibited by OST1 phosphorylation.Citation39 The promotion of anion efflux and suppression of cation influx promote decrease in turgor pressure in guard cells and stomatal closure. OST1 also targets RBOH that generates ROS. Bursts of ROS activate Ca2+ channels in both the plasma membrane and vacuolar membrane, and the resulting changes in Ca2+ in the cytosol lead to downstream signaling responses.Citation39
When stomata in leaves or epidermal peels of A. thaliana are exposed to a suspension of Pst DC3000, they close within 1–2 h and reverted to an open state after 3 h.Citation11 As mentioned earlier, the opening is due to the effector molecule produced by some strains of P. syringae called coronatine (COR), which structurally mimics JA-isoleucine (JA-Ile), the active form of the hormone,Citation18 and induces stomatal opening as shown in . COR binds to CORONATINE INSENSITIVE 1 (COI1), an F-box protein that senses JA-Ile. This binding triggers COI1-dependent degradation of the substrates of COI1, the JASMONATE ZIM DOMAIN (JAZ) proteins.Citation40 COR also induces expression of three transcription factors (TFs) in the NAC family, ANAC019 (At1G52890), ANAC055 (At3G15500), and ANAC072 (At4G27410), which have binding sites for the MYC2. MYC2 is a TF released from a complex with JAZ, after COR-triggered degradation of JAZ proteins. A triple knockout mutant of the NAC TFs is insensitive to COR-triggered stomatal reopening and maintains higher levels of SA than wild type Arabidopsis after bacterial inoculation. The NAC TFs putatively decrease SA synthesis via transcriptional repression of isochorismate synthase gene 1 (ICS1) and increase SA storage via SA glucosyl transferase gene 1 (SAGT1), aiding in the reopening of stomata.Citation18,Citation41,Citation42
JA and SA often exhibit antagonistic effects in plant defense responses.Citation43,Citation44 P. syringae-triggered stomatal closure in both Phaseolus vulgaris and A. thaliana was found to be compromised during high relative humidity, which was accompanied by early up-regulation of the JA pathway and simultaneous down-regulation of the SA pathway in guard cells.Citation45 Recent results show that a transcription factor NTM1-LIKE 9 (NTL9) is required for SA biosynthesis in guard cells and closure of stomata in response to pathogens, linking increase of SA to the stomatal closure response.Citation22 Additionally, results from two Arabidopsis NO mutants, nia1 and nia2, demonstrate that SA-induced stomatal closure is regulated by NO generation in guard cells.Citation23
Convergence of biotic and abiotic stress signaling
While guard cells are able to perceive pathogen attack and close stomata, they also respond to abiotic conditions including heat, drought, high or low CO2, and high or low humidity. ABA is a key regulator that controls many abiotic stress responses, including stomatal movement.Citation46 In guard cells, levels of endogenous ABA increase during abiotic stress and cause stomatal closure by decreasing solute potential.Citation24 However, in other plant cell types during combined abiotic and biotic stress response, ABA can act either synergistically with JA or antagonistically with SA during stress responses.Citation46 Exogenously applied or endogenously produced ABA is correlated with increased bacterial growth in Arabidopsis challenged with P. syringae, and the ABA biosynthetic mutant aba3-1 showed reduced susceptibility to P. syringae.Citation47 Overall, ABA may act to fine tune the cellular response to pathogens by promoting JA signaling while suppressing SA signaling.Citation48 This hormone crosstalk is specific for the type of pathogen, in that biotrophic pathogens induce SA biosynthesis and SAR, while necrotrophic pathogens induce ethylene and JA biosynthesis and signaling that are antagonistic to SA.Citation46
Several MPKs are activated to induce stomatal closure in response to abiotic and biotic stresses. Both MPK3 and MPK6 function interdependently of ABA signaling, and both the ABA and MPK3/6 cascades are required for stomatal immunity.Citation39,Citation49 The MPK3/6 cascade leads to decrease in malate/citrate levels in the guard cells and altered osmolarity to reduce tugor pressure and close the stomata, whereas ABA signaling activates OST1, which phosphorylates RBOHD and SLAC1.Citation39,Citation49 Cl− efflux mediated by SLAC1 leads to K+ efflux from guard cells and stomatal closure. MPK9 and MPK12 are guard-cell specific and involved in ABA and ROS responsive stomatal closure via SLAC1.Citation49
Convergence of these biotic and abiotic signaling pathways has been the subject of investigation for decades. The complex interplay between the susceptibility of the plant, the inoculum potential of the pathogen, and the impact of the environmental conditions is a concept first described in the 1960s by George McNew as the disease triangle.Citation50 The disease triangle is a concept regarding the role of the environment in disease control. McNew proposed that there were six factors relating the environment, pathogen, and plant, and they interact to determine the impact of pathogens on a host under favorable conditions. These factors include: the physical environment (temperature, humidity, etc.) and duration of the infection, pathogen prevalence and virulence, age of the plant, and inherent susceptibility of host plant. The disease triangle concept is widely accepted in the field of plant pathology and is supported by current research.Citation50,Citation51 Recent transciptomic analysis found 138 pathogen-induced genes that exhibited stronger transcriptional response when the host plant Vitis vinifera was exposed simultaneously to infection by the pathogen Xylella fastidiosa and drought stress than either stress alone.Citation52 These molecular results correlated to the physiological and phenotypic results with respect to water content, photosynthesis rate, severity of disease symptoms, and extent of pathogen colonization. Similarly, results of microarray and RT-qPCR experiments showed 20 novel genes that were uniquely regulated during concurrent drought and pathogen stress in A. thaliana infected with Pst DC3000.Citation52 Future studies involving other abiotic and biotic stress combinations will greatly facilitate elucidation of the “cross-tolerance” mechanism in the disease triangle.Citation54–Citation56
Mobile signals for SAR
For SAR to occur, mobile signals must be generated at the site of infection and move to uninfected tissues. Most of these signals are thought to travel via the plant vasculature (xylem and phloem), however, there is some evidence for volatile signals as well.Citation21,Citation57 There are six metabolites that have been identified as potential long-distance signals, which include methyl salicylate (MeSA), glycerol-3-phosphate (G3P), DEFECTIVE IN INDUCED RESISTANCE 1–1 (DIR1), azelaic acid (AzA), dehydroabietinal (DA), and pipecolic acid (PiP). Evidence for their being mobile signals is reviewed by Dempsey and Klessig (2012) .Citation21
Methyl salicylate (MeSA)
MeSA increases in leaves inoculated with pathogens that induce SAR resonse.Citation20 When SA accumulates in pathogen-infected leaves, some of it is converted to MeSA. As MeSA accumulates, it travels to systemic leaves via the phloem. Once in the systemic leaves, MeSA is converted back to SA. This newly released SA triggers (primes) systemic defense responses, as will be discussed in the next section.Citation58 MeSA has also been implicated as an airborne signal for plant defenses.Citation59
Glycerol-3-phosphate (G3P)
SUPPRESSOR OF FATTY ACID DESATURASE DEFICIENCY 1 (SFD1) encodes a glycerol-3-phosphate dehydrogenase (G3PDH), aka dihydroxyacetone phosphate (DHAP) reductase.Citation60,Citation61 This enzyme is required to catalyze the reduction of DHAP in an NADH-dependent manner to generate G3P. This is a necessary precursor for the synthesis of all glycerolipids, which include membrane and storage lipids. Activity of G3PDH expressed from SFD1 is required in the chloroplasts for SAR and lipid metabolism to occur.Citation21 The signal that is derived from SFD1 may be G3P, but this is still unclear. G3P increases in leaves inoculated with SAR-inducing pathogens and azelaic acid (AzA) promotes accumulation of G3P. In addition, G3P also promotes the conversion of MeSA to SA in distal leaves.Citation20
Defective in induced resistance 1-1 (DIR1)
DIR1 encodes a novel type of lipid transfer protein (LTP) that is able to bind two molecules of long-chain fatty acids with high affinity.Citation62,Citation63 Evidence suggests that DIR1 can enter the phloem via the cytosol of companion cells and act as a long-distance chaperone to systemic tissue for three other long distance SAR signaling molecules, G3P, dehydroabietinal (DA), and AzA.Citation4,Citation64 DIR1 appears to interact with itself and AZELAIC ACID-INDUCED 1 (AZI1) (see below). Both DIR1 and AZI1 are required for G3P- and AzA-induced SAR. The presence of DIR1 and AZI1 enhance sensitivity to the signaling form of DA (see below).Citation20
AzA
Treatment with AzA did not induce SA accumulation or PR expression, but did enhance PR gene activation after secondary pathogen inoculation. AZI1 is a predicted secreted protease inhibitor/seed storage protein/LTP, which is not homologous to DIR1. AZI1 is possibly involved in the production or transport of the mobile SAR signal, but not the perception of it.Citation65,Citation66 AzA levels increase in leaves inoculated with SAR-inducing pathogens and AzA is transported to distal leaves.Citation20
DA
Both DA and AzA were found in an analysis of small molecules enriched in the petiole exudates of Arabidopsis plants treated with avirulent (Avr) P. syringae. AzA and DA can induce local and systemic resistance to virulent pathogens when applied locally. However, DA appears to require the interaction of one or more proteins for SAR induction, as trypsin treatment was able to abolish the SAR-inducing activity.Citation67–Citation69 During SAR, total content of DA does not change and it is proposed that DA is mobilized from a non-signaling form to a signaling form which is presumably transported via the phloem.Citation20
Pipecolic acid (pip)
PiP is a product of lysine degradation,Citation21 found in elevated levels in petiole extracts and systemic leaves of pathogen-inoculated plants. Like AzA, PiP does not increase SA levels, but primes systemic tissue for faster and stronger secondary response.Citation70,Citation71 PiP is induced by P. syringae in an AGD2-LIKE DEFENSE RESPONSE PROTEIN1 (ALD1)-dependent mannerCitation70,Citation73 and is suggested to amplify its own synthesis as well as the activity of the SA via an SA synthesis enzyme (ICSI) possibly by a transcriptional amplification loop.Citation20,Citation70 SA amplification involves FMO1 (FLAVIN-DEPENDENT MONOOXYGENASE 1) and PAD4 (PHYTOALEXIN-DEFICIENT 4). The SA amplification loop activates NON-EXPRESSER OF PR1 (NPR1) by SA, ultimately resulting in SAR signaling.Citation20
Research on mobile signals that induce SAR has focused on the transmission of these signals from infected mesophyll cells to systemic non-infected mesophyll cells, but has not addressed potential interactions with guard cells, which have been shown to respond to pathogen infection.Citation11 Signals from mesophyll apoplasts can induce stomatal responses to CO2 in Commelina communis.Citation74 Furthermore, by using mesophyll segments that were sandwiched by gel and doughnut-shaped spacers made of either cellophane or polyethylene, the authors found that the mesophyll signals that move to the epidermis were in an aqueous, not a gaseous phase.Citation74 Interestingly, they also found that stomatal opening was dependent on mesophyll photosynthesis, but that stomatal closure was less dependent on mesophyll photosynthesis signals. This demonstrates that guard cells can perhaps receive signals both from the mesophyll cells and also from the outside environment to induce stomatal movement. As guard cells mature, their plasmodesmata become truncated and nonfunctional, which eliminates intercellular communication with surrounding epidermal cells.Citation75 However, plants maintain some plasmodesmata between the bundle sheath/phloem parenchyma cells and the sieve element-companion cell complexes possibly for of apoplastic loading and long distance intercellular communication.Citation76 It seems plausible that plants cells would require a method of exchange of mobile signals during pathogen attack, and that different cell types would be involved in this signaling process.
Signal amplification in systemic leaves
When long-distance SAR signaling molecules reach distal tissues from pathogen infected tissues, they must be perceived by the cells in the systemic tissues in order to initiate the global SAR response. Although the phenomenon of SAR has been known since the 1930s, the receptors of mobile signals (see the previous section) have not been identified. What is best known is that SA is accumulated in the systemic tissue sometime after the mobile signal is sent. SA accumulation leads to the secretion of antimicrobial pathogenesis-related (PR) proteins. This leads to the rest of the plant being protected from a broad spectrum of pathogens for weeks to months. PR proteins and peptides consisting of a large number of members which differ in occurrence (spatial and temporal), expression levels, and biological activities.Citation77
The master regulator of SA signaling is NPR1.Citation78 NPR1 was first identified in A. thaliana and is required for PR gene expression, SA signaling and SAR.Citation4 SA directly binds to NPR1 adapter proteins. NPR1 is one of ten TGA transcription factors (a family of transcription factors with a TGACG motif) in Arabidopsis, and seven of them have been found to interact with NPR1 in yeast two-hybrid screens.Citation4 TGAs have been shown to bind directly to PR gene promoters. PR proteins are the activators of SAR with a molecular weight range of 5–75 kDa that are secreted or targeted to the vacuole.Citation77 It is difficult to test the contribution of each PR protein because they work in concert and are encoded by multiple genes in gene clusters.Citation77 One point of confusion in the field is the temporal aspect of the SAR response, as treatments are done in different plants species, at different ages, in different organs or leaves, and under different growth conditions. In general, expression of PR genes is up-regulated within 24–48 h in systemic tissues after the primary inoculation.Citation4
Kumar et al. found that inoculation of Arabidopsis plants with the rhizobacteria Bacillus subtilis FB17 via the roots, restricted the entry of Pst DC3000 through the stomata when the plants were co-inoculated with both microorganisms.Citation79 Arabidopsis plants that were inoculated only with the rhizobacteria showed reduced stomatal aperture one hour after inoculation, similar to inoculation with Pst DC3000. However, the rhizobacteria-inoculated plants maintained a reduced stomatal aperture even at three hours post-inoculation, unlike Pseudomonas-inoculated stomata that reopen after three hours.Citation79 This demonstrates the interconnection between environmental conditions and immune response, and the ability of the plant immune system to prepare systemic tissues for response to different microbial organisms.
Defense priming in systemic tissues
A relatively new and exciting aspect of SAR research is the SAR defense priming or conditioning. This can be thought of as enabling the plant to maintain a vigilant or alarmed status in which they are able to react faster and more effectively to pathogen attack.Citation80 Research by Jung et al. has shown that exogenous AzA treatment enabled plants to accumulate higher levels of SA and PR1 transcripts.Citation5 They also found that primary inoculation with SAR-inducing pathogens led to defense priming in distal leaves, and that the whole plant mobilized defense more rapidly upon secondary infection. Exogenous application of PiP had similar effects, in that primed plants had stronger induction of defense genes after an initial challenge infection.Citation70 Priming is found to be dependent on two MAP kinases, MPK3 and MPK6.Citation81 These SAR signaling mechanisms and priming responses are summarized in .
Figure 2. Local and systemic response to P. syringae pv. Tomato (Pst) infection in A. thaliana. Plant immune response requires the convergence and interaction of both localized pathogen response pathways as well as initiation of enhanced pathogen resistance in non-infected systemic tissues. The process begins with 1) the primary infection by Pst, which then triggers 2) PTI through perception of MAMP (PAMP) by PRRs and the activation of MAPKs and production of NO, ROS, and callose deposition. Additionally, certain pathogens including Pst activate ETI response by producing effector proteins that bind to NB-LRR containing R proteins in the plant. This often, but not always, triggers HR response in the locally infected plant tissue. 3) Putative SAR signals including DIR1, G3P, DA, AzA, and MeSA are produced in the infected tissue and transported to systemic tissues. DIR1, a novel type of lipid transfer protein, acts as a long-distance chaperone for G3P, DA, and AzA and can interact with itself as well as AZI in the process. These SAR signals lead to 4) the accumulation of SA in non-infected tissue via FMO1 and PAD4. Additionally, PiP is induced by ALD1, which amplifies its own synthesis as well as activates SA synthesis. This SA amplification loop leads to 5) NPR1 activation, a key regulator in SAR signaling. Direct binding of SA to NPR1 adapter proteins leads to transcriptional activation of a family of PR genes. PR gene expression is upregulated within 24–48 h in systemic tissues after primary infection. SAR response in non-infected tissue leads to 6) Defense priming, which enables the plant to maintain a state of vigilance to pathogen attack, and react faster and with greater effectiveness. Priming of non-infected, systemic tissue requires MPK3 and MPK6 and leads to defense gene expression as well as heritable effects due to histone methylation and modifications of chromatin. Please note leaves 4 to 6 represent systemic tissues and the depicted processes can occur in any of them, therefore no arrows were used.
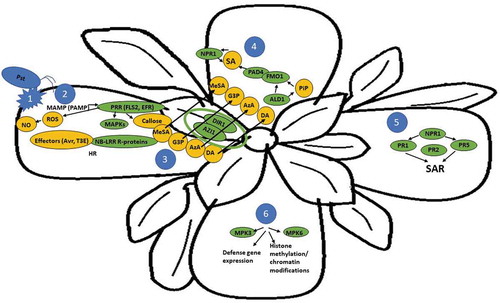
One issue with the research on priming is that it is largely based on gene expression data of whole leaf tissue. This masks the responses of specialized cells such as guard cells and it overlooks protein and metabolite regulations (e.g., post-translational modifications and hormone crosstalk) that may play important roles in the priming and the strong response of the primed tissue. Future research focusing on single cell types and their cross-talk in local and distant tissues will greatly improve knowledge of plant defense mechanisms, which can be applied toward enhancing plant productivity and food security.
Conclusion
Plant hosts have coevolved with their pathogens to form a highly complex immune system. The mechanisms by which plants confer immunity to non-infected tissues have been studied for decades, and yet new factors involved in immune response are being elucidated. One recent development has been the important role that stomata play in the local immune response to plant pathogens. Additionally, the plant hormone SA, which regulates SAR response in non-infected tissues has also been shown to regulate stomatal closure in response to pathogens. Potential SAR long-distance signals have been identified, but it is still unknown how these signals affect different cell types of the systemic tissue as part of immune priming. Future research focusing on molecular changes in different cells and cell types in response to local and distal pathogen infection under various environmental conditions will greatly improve our understanding of the disease triangle and inform rational engineering of crops for enhanced disease resistance without compromising yield.
Acknowledgments
Work on guard cell signaling in the Chen laboratory has been supported by NSF Grants MCB-0818051, MCB-1158000, MCB-1412547, and IOS-1758820.
Additional information
Funding
References
- Misra BB, Chaturvedi R. When plants brace for the emerging pathogens. Physiol Mol Plant Pathol. 2015;92:181–185. doi:10.1016/j.pmpp.2015.03.004.
- Spoel SH, Dong XN. How do plants achieve immunity? Defense without specialized immune cells. Nat Rev Immunol. 2012;12(2):89–100. doi:10.1038/nri3141.
- van Wersch R, Li X, Zhang YL. Mighty dwarfs: arabidopsis autoimmune mutants and their usages in genetic dissection of plant immunity. Front Plant Sci. 2016;7:1717. doi:10.3389/fpls.2016.01717.
- Fu ZQ, Dong XN. Systemic acquired resistance: turning local infection into global defense. In: Merchant SS, editor. Annual Review of Plant Biology Vol. 64. Palo Alto, USA. Annual Reviews; 2013. p. 839–863. doi:10.1146/annurev-arplant-042811-105606.
- Jung HW, Tschaplinski TJ, Wang L, Glazebrook J, Greenberg JT. Priming in systemic plant immunity. Science. 2009;324(5923):89–91. doi:10.1126/science.1170025.
- Yi HS, Yang JW, Choi HK, Ghim SY, Ryu CM. Benzothiadiazole-elicited defense priming and systemic acquired resistance against bacterial and viral pathogens of pepper under field conditions. Plant Biotechnol Rep. 2012;6:373–380. doi:10.1007/s11816-012-0234-3.
- Zeng W, Brutus A, Kremer JM, Withers JC, Gao X, Jones AD, He SY. A genetic screen reveals Arabidopsis stomatal and/or apoplastic defenses against Pseudomonas syringae pv. tomato DC3000. PLoS Pathog. 2011;7(10):e1002291. doi:10.1371/journal.ppat.1002291.
- Gohre V, Robatzek S. Breaking the barriers: microbial effector molecules subvert plant immunity. Annu Rev Phytopathol. 2008;46:189–215. doi:10.1146/annurev.phyto.46.120407.110050.
- Stavrinides J, McCann HC, Guttman DS. Host-pathogen interplay and the evolution of bacterial effectors. Cell Microbiol. 2008;10(2):285–292. doi:10.1111/j.1462-5822.2007.01078.
- Brooks DM, Hernandez-Guzman G, Kloek AP, Alarcon-Chaldez F, Sreedharan A, Rangaswamy V, Penaloza-Vazquez A, Bender CL, Kunkel BN. Identification and characterization of a well-defined series of coronatine biosynthetic mutants of Pseudomonas syringae pv. tomato DC3000. Mol Plant Microbe Interact. 2004;17(11):162–174. doi:10.1094/MPMI.2004.17.2.162.
- Melotto M, Underwood W, He SY. Role of stomata in plant innate immunity and foliar bacterial diseases. Annu Rev Phytopathol. 2008;46:101–122. doi:10.1146/annurev.phyto.121107.104959.
- McLachlan DH, Kopischke M, Robatzek S. Gate control: guard cell regulation by microbial stress. New Phytol. 2014;203(4):1049–1063. doi:10.1111/nph.12916.
- Sawinski K, Mersmann S, Robatzek S, Bohmer M. Guarding the green: pathways to stomatal immunity. Mol Plant Microbe Interact. 2013;26(6):626–632. doi:10.1094/MPMI-12-12-0288-CR.
- Montillet JL, Leonhardt N, Mondy S, Tranchimand S, Rumeau D, Boudsocq M, Garcia AV, Douki T, Bigeard J, Lauriere C, et al. An abscisic acid-independent oxylipin pathway controls stomatal closure and immune defense in Arabidopsis. PLoS Biol. 2013;11(3): e1001513. doi:10.1371/journal.pbio.1001513.
- Shang Y, Dai C, Lee MM, Kwak JM, Nam KH. BRI1-associated receptor kinase 1 regulates guard cell ABA signaling mediated by open stomata 1 in Arabidopsis. Mol Plant. 2016;9(3):447–460. doi:10.1016/j.molp.2015.12.014.
- Engineer CB, Hashimoto-Sugimoto M, Negi J, Israelsson-Nordstrom M, Azoulay-Shemer T, Rappel WJ, Iba K, Schroeder JI. CO2 sensing and CO2 peculation of stomatal conductance: advances and open questions. Trends Plant Sci. 2016;21(1):16–30. Epub 2015 Oct 5. doi:10.1016/j.tplants.2015.08.014.
- Munemasa S, Hauser F, Park J, Waadt R, Brandt B, Schroeder JI. Mechanisms of abscisic acid-mediated control of stomatal aperture. Curr Opin Plant Biol. 2015;28:154–162. doi:10.1016/j.pbi.2015.10.010.
- Melotto M, Underwood W, Koczan J, Nomura K, He SY. Plant stomata function in innate immunity against bacterial invasion. Cell. 2006;126(5):969–980. doi:10.1016/j.cell.2006.06.054.
- Carella P, Merl-Pham J, Wilson DC, Dey S, Hauck SM, Vlot AC, Cameron RK. Comparative proteomics analysis of phloem exudates collected during the induction of systemic acquired resistance. Plant Physiol. 2016;171(2):1495–1510. doi:10.1104/pp.16.00269.
- Shah J, Zeier J. Long-distance communication and signal amplification in systemic acquired resistance. Front Plant Sci. 2013;4(30):1–16. doi:10.3389/fpls.2013.00030.
- Dempsey DA, Klessig DF. SOS - too many signals for systemic acquired resistance? Trends Plant Sci. 2012;17(9):538–545. doi:10.1016/j.tplants.2012.05.011.
- Zheng XY, Zhou M, Yoo H, Pruneda-Paz JL, Spivey NW, Kay SA, Dong XNA. Spatial and temporal regulation of biosynthesis of the plant immune signal salicylic acid. Proc Natl Acad Sci U S A. 2015;112(30):9166–9173. doi:10.1073/pnas.1511182112.
- Hao FS, Zhao SL, Dong H, Zhang H, Sun LR, Miao C. Nia1 and Nia2 are involved in exogenous salicylic acid-induced nitric oxide generation and stomatal closure . J Int Plant Biol. 2010;52(3):298–307. doi:10.1111/j.1744-7909.2010.00920.
- Assmann SM, Jegla T. Guard cell sensory systems: recent insights on stomatal responses to light, abscisic acid, and CO2. Curr Opin Plant Biol. 2016;33:157–167. doi:10.1016/j.pbi.2016.07.003.
- Liu J, Chen SF, Chen LJ, Zhou Q, Wang ML, Feng DR, Li JF, Wang JF, Wang HB, Liu B. BIK1 cooperates with BAK1 to regulate constitutive immunity and cell death in Arabidopsis. J Integr Plant Biol. 2017;59(4):234–239. doi:10.1111/jipb.12529.
- Mersmann S, Bourdais G, Rietz S, Robatzek S. Ethylene signaling regulates accumulation of the FLS2 receptor and is required for the oxidative burst contributing to plant immunity. Plant Physiol. 2010;154(1):391–400. doi:10.1104/pp.110.154567.
- Jeworutzki E, Roelfsema MRG, Anschutz U, Krol E, Elzenga JTM, Felix G, Boller T, Hedrich R, Becker D. Early signaling through the Arabidopsis pattern recognition receptors FLS2 and EFR involves Ca2+-associated opening of plasma membrane anion channels. Plant J. 2010;62(3):367–378. doi:10.1111/j.1365-313X.2010.04155.x.
- Macho AP, Boutrot F, Rathjen JP, Zipfel C. ASPARTATE OXIDASE plays an important role in Arabidopsis stomatal immunity. Plant Physiol. 2012;159(4):1845–1856. doi:10.1104/pp.112.199810.
- Lee S, Yang DS, Uppalapati SR, Sumner LW, Mysore KS. Suppression of plant defense responses by extracellular metabolites from Pseudomonas syringae pv. tabaci in Nicotiana benthamiana. BMC Plant Biol. 2013;13:65. doi:10.1186/1471-2229-13-65.
- Jones JDG, Dangl JL. The plant immune system. Nature. 2006;444(7117):323–329. doi:10.1038/nature05286.
- Zipfel C, Rathjen JP. Plant immunity: avrPto targets the frontline. Cur Biol. 2008;18(5):R218–R220. doi:10.1016/j.cub.2008.01.016.
- Monaghan J, Zipfel C. Plant pattern recognition receptor complexes at the plasma membrane. Curr Opin Plant Biol. 2012;15(4):349–357. doi:10.1016/j.pbi.2012.05.006.
- Ross AF. Systemic effects of local lesion formation. Beemster ABR, Dijkstra J. editors. Viruses of Plants. Amsterdam: North-Holland Publishing Company;1966. p. 127–150.
- Chester KS. The problem of acquired physiological immunity in plants. Q Rev Biol. 1933;8:129–154. doi:10.1086/394430.
- Mishina TE, Zeier J. Pathogen-associated molecular pattern recognition rather than development of tissue necrosis contributes to bacterial induction of systemic acquired resistance in Arabidopsis. Plant J. 2007;50(3):500–513. doi:10.1111/j.1365-313X.2007.03067.x.
- Chen C, Xiao YG, Li X, Ni M. Light-regulated stomatal aperture in Arabidopsis. Mol Plant. 2012;5(3):566–572. doi:10.1093/mp/sss039.
- Belin C, de Franco PO, Bourbousse C, Chaignepain S, Schmitter JM, Vavasseur A, Giraudat J, Barbier-Brygoo H, Thomine S. Identification of features regulating OST1 kinase activity and OST1 function in guard cells. Plant Physiol. 2006;141(4):1316–1327. doi:10.1104/pp.106.079327.
- Mustilli AC, Merlot S, Vavasseur A, Fenzi F, Giraudat J. Arabidopsis OST1 protein kinase mediates the regulation of stomatal aperture by abscisic acid and acts upstream of reactive oxygen species production. Plant Cell. 2002;14(12):3089–3099. doi:10.1105/tpc.007906.
- Zhang T, Chen S, Harmon AC. Protein phosphorylation in stomatal movement. Plant Signal Behav. 2014;9(11):e972845. doi:10.4161/15592316.2014.972845.
- Yan J, Zhang C, Gu M, Bai Z, Zhang W, Qi T, Cheng Z, Peng W, Luo H, Nan F, et al. The Arabidopsis CORONATINE INSENSITIVE1 protein is a jasmonate receptor. Plant Cell. 2009;21(8):2220–2236. doi:10.1105/tpc.109.065730.
- Zheng XY, Spivey NW, Zeng WQ, Liu PP, Fu ZQ, Klessig DF, He SY, Dong XN. Coronatine promotes Pseudomonas syringae virulence in plants by activating a signaling cascade that inhibits salicylic acid accumulation. Cell Host Microbe. 2012;11(6):587–596. doi:10.1016/j.chom.2012.04.014.
- Zhang L, Zhang F, Melotto M, Yao J, He SY. Jasmonate signaling and manipulation by pathogens and insects. J Exp Bot. 2017;68(6):1371–1385. doi:10.1093/jxb/erw478.
- Ndamukong I, Al Abdallat A, Thurow C, Fode B, Zander M, Weigel R, Gatz C. SA- inducible Arabidopsis glutaredoxin interacts with TGA factors and suppresses JA-responsive PDF1.2 transcription. Plant J. 2007;50(1):128–139. doi:10.1111/j.1365-313X.2007.03039.x.
- Leon-Reyes A, Van der Does D, De Lange ES, Delker C, Wasternack C, Van Wees SCM, Ritsema T, Pieterse CMJ. Salicylate-mediated suppression of jasmonate-responsive gene expression in Arabidopsis is targeted downstream of the jasmonate biosynthesis pathway. Planta. 2010;232(6):1423–1432. doi:10.1007/s00425-010-1265-z.
- Panchal S, Chitrakar R, Thompson BK, Obulareddy N, Roy D, Hambright WS, Melotto M. Regulation of stomatal defense by air relative humidity. Plant Physiol. 2016;172(3):2021–2032. doi:10.1104/pp.16.00696.
- Atkinson NJ, Urwin PE. The interaction of plant biotic and abiotic stresses: from genes to the field. J Exp Bot. 2012;63(10):3523–3543. doi:10.1093/jxb/ers100.
- Fan J, Hill L, Crooks C, Doerner P, Lamb C. Abscisic acid has a key role in modulating diverse plant-pathogen interactions. Plant Physiol. 2009;150(4):1750–1761. doi:10.1104/pp.109.137943.
- Zhou Y, Vroegop-Vos I, Schuurink RC, Pieterse CMJ, Van Wees SCM. Atmospheric CO2 alters resistance of Arabidopsis to Pseudomonas syringae by affecting abscisic acid accumulation and stomatal responsiveness to coronatine. Front Plant Sci. 2017;8:700. doi:10.3389/fpls.2017.00700.
- Su JB, Zhang MM, Zhang L, Sun TF, Liu YD, Lukowitz W, Xu J, Zhang SQ. Regulation of stomatal immunity by interdependent functions of a pathogen-responsive MPK3/MPK6 cascade and abscisic acid. Plant Cell. 2017;29(3):526–542. doi:10.1105/tpc.16.00577.
- Scholthof KBG. The disease triangle: pathogens, the environment and society. Nat Rev Microbiol. 2007;5:152–156. doi:10.1038/nrmicro1596.
- McNew GL. The nature, origin, and evolution of parasitism. In: Horsfall JG, Dimond AE, editors. Plant pathology: an advanced treatise. New York: Academic Press; 1960. p. 19–69.
- Choi HK, Iandolino A, Da Silva FG, Cook DR. Water deficit modulates the response of Vitis vinifera to the Pierce’s disease pathogen Xylella fastidiosa. Mol Plant Microbe Interact. 2013;26(6):643–657. doi:10.1094/MPMI-09-12-0217-R.
- Gupta A, Sarkar AK, Senthil-Kumar M. Global transcriptional analysis reveals unique and shared responses in Arabidopsis thaliana exposed to combined drought and pathogen stress. Front Plant Sci. 2016;7:686. doi:10.3389/fpls.2016.00686.
- Zhao C, Wang X, Wang X, Wu K, Li P, Chang N, Wang J, Wang F, Li J, Bi Y. Glucose-6-phosphate dehydrogenase and alternative oxidase are involved in the cross tolerance of highland barley to salt stress and UV-B radiation. J Plant Physiol. 2015;181:83–95. doi:10.1016/j.jplph.2015.03.016.
- Capiati DA, País SM, Téllez-Iñón MT. Wounding increases salt tolerance in tomato plants: evidence on the participation of calmodulin-like activities in cross-tolerance signalling. J Exp Bot. 2006;57(10):2391–2400. doi:10.1093/jxb/erj212.
- Manavella PA, Arce AL, Dezar CA, Bitton F, Renou JP, Crespi M, Chan RL. Cross-talk between ethylene and drought signalling pathways is mediated by the sunflower Hahb-4 transcription factor. Plant J. 2006;48(1):125–137. doi:10.1111/j.1365-313X.2006.02865.x.
- Shah J, Chaturvedi R, Chowdhury Z, Venables B, Petros RA. Signaling by small metabolites in systemic acquired resistance. Plant J. 2014;79(4):645–658. doi:10.1111/tpj.12464.
- Park SW, Kaimoyo E, Kumar D, Mosher S, Klessig DF. Methyl salicylate is a critical mobile signal for plant systemic acquired resistance. Science. 2007;318(5847):113–116. doi:10.1126/science.1147113.
- Baldwin IT, Halitschke R, Paschold A, von Dahl CC, Preston CA. Volatile signaling in plant-plant interactions: “Talking trees” in the genomics era. Science. 2006;311(5762):812–815. doi:10.1126/science.1118446.
- Nandi A, Welti R, Shah J. The Arabidopsis thaliana dihydroxyacetone phosphate reductase gene SUPPRESSOR OF FATTY ACID DESATURASE DEFICIENCY1 is required for glycerolipid metabolism and for the activation of systemic acquired resistance. Plant Cell. 2004;16(2):465–477. doi:10.1105/tpc.016907.
- Chanda B, Xia Y, Mandal MK, Yu KS, Sekine KT, Gao QM, Selote D, Hu YL, Stromberg A, Navarre D, et al. Glycerol-3-phosphate is a critical mobile inducer of systemic immunity in plants. Nat Genet. 2011;43(5):421–427. doi:10.1038/ng.798.
- Maldonado AM, Doerner P, Dixon RA, Lamb CJ, Cameron RK. A putative lipid transfer protein involved in systemic resistance signalling in Arabidopsis. Nature. 2002;419(6905):399–403. doi:10.1038/nature00962.
- Lascombe MB, Bakan B, Buhot N, Marion D, Blein JP, Larue V, Lamb C, Prange T. The structure of “defective in induced resistance” protein of Arabidopsis thaliana, DIR1, reveals a new type of lipid transfer protein. Protein Sci. 2008;17(9):1522–1530. doi:10.1110/ps.035972.108.
- Champigny MJ, Shearer H, Mohammad A, Haines K, Neumann M, Thilmony R, He SY, Fobert P, Dengler N, Cameron RK. Localization of DIR1 at the tissue, cellular and subcellular levels during systemic acquired resistance in Arabidopsis using DIR1: gUSand DIR1: eGFPreporters. BMC Plant Biol. 2011;11:125. doi:10.1186/1471-2229-11-125.
- Cecchini NM, Steffes K, Schlappi MR, Gifford AN, Greenberg JT. Arabidopsis AZI1 family proteins mediate signal mobilization for systemic defence priming. Nat Commun. 2015;23(6):7658. doi:10.1038/ncomms8658.
- Kachroo A, Robin GP. Systemic signaling during plant defense. Curr Opin Plant Bio. 2013;16(4):527–533. doi:10.1016/j.pbi.2013.06.019.
- Chaturvedi R, Venables B, Petros RA, Nalam V, Li VM, Wang XM, Takemoto LJ, Shah J. An abietane diterpenoid is a potent activator of systemic acquired resistance. Plant J. 2012;71(1):161–172. doi:10.1111/j.1365-313X.2012.04981.x.
- Isaacs M, Carella P, Faubert J, Rose JKC, Cameron RK. Orthology analysis and in vivo complementation studies to elucidate the role of DIR1 during systemic acquired resistance in Arabidopsis thaliana and Cucumis sativus. Front Plant Sci. 2016;7:566. doi:10.3389/fpls.2016.00566.
- Cameron RK, Carella P, Isaacs M, Champigny M, Merl-Pham J, Dey S, Vlot AC. Using DIR1 to investigate long-distance signal movement during systemic acquired resistance. Can J Plant Pathol. 2016;38(1):19–24. doi:10.1080/07060661.2016.1147497.
- Navarova H, Bernsdorff F, Doring AC, Zeier J. Pipecolic acid, an endogenous mediator of defense amplification and priming, is a critical regulator of inducible plant immunity. Plant Cell. 2012;24(12):5123–5141. doi:10.1105/tpc.112.103564.
- Bernsdorff F, Doring AC, Gruner K, Schuck S, Brautigam A, Zeier J. Pipecolic acid orchestrates plant systemic acquired resistance and defense priming via salicylic acid-dependent and -independent pathways. Plant Cell. 2016;28(1):102–129. doi:10.1105/tpc.15.00496.
- Dixon DC, Cutt JR, Klessig DF. Differential targeting of the tobacco PR-1 pathogenesis-related proteins to the extracellular space and vacuoles of crystal idioblasts. EMBO J. 1991;10(6):1317–1324. doi:10.1002/j.1460-2075.1991.tb07650.x.
- Cecchini NM, Jung HW, Engle NL, Tschaplinski TJ, Greenberg JT. ALD1 regulates basal immune components and early inducible defense responses in Arabidopsis. Mol Plant Microbe Interact. 2015;28(4):455–466. doi:10.1094/MPMI-06-14-0187-R.
- Fujita T, Noguchi K, Terashima I. Apoplastic mesophyll signals induce rapid stomatal responses to CO2 in Commelina communis. New Phytol. 2013;199(2):395–406. doi:10.1111/nph.12261.
- Palevitz BA, Hepler PK. Changes in dye coupling of stomatal cells of Allium and Commelina demonstraded by micorinjection of lucifer yellow. Planta. 1985;164(4):473. doi:10.1007/BF00395962.
- Oparka KJ, Cruz SS. The great escape: phloem transport and unloading of macromolecules. Annu Rev Plant Physiol Plant Mol Biol. 2000;51:323–347. doi:10.1146/annurev.arplant.51.1.323.
- Sels J, Mathys J, De Coninck BMA, Cammue BPA, De Bolle MFC. Plant pathogenesis-related (PR) proteins: A focus on PR peptides. Plant Physiol Biochem. 2008;46(11):941–950. doi:10.1016/j.plaphy.2008.06.011.
- Pajerowska-Mukhtar KM, Emerine DK, Mukhtar MS. Tell me more: roles of NPRs in plant immunity. Trends Plant Sci. 2013;18(7):402–411. doi:10.1016/j.tplants.2013.04.004.
- Kumar AS, Lakshmanan V, Caplan JL, Powell D, Czymmek KJ, Levia DF, Bais HP. Rhizobacteria Bacillus subtilis restricts foliar pathogen entry through stomata. Plant J. 2012; 72(4):694–706. doi:10.1111/j.1365-313X.2012.05116.x.
- Conrath U. Molecular aspects of defence priming. Trends Plant Sci. 2011;16(10):524–531. doi:10.1016/j.tplants.2011.06.004.
- Beckers GJM, Jaskiewicz M, Lui Y, Underwood WR, He SY, Zhang S, Conrath U. Mitogen-Activated Protein Kinases 3 and 6 are required for full priming stress responses in Arabidopsis thaliana. Plant Cell. 2009;21(3):944–953. doi:10.1105/tpc.108.062158.