ABSTRACT
One of the most important challenges for individual plants is coexistence with their neighbors. To compensate for their sessile lifestyle, plants developed complex and sophisticated chemical systems of communication among each other. Site-specific biotic and abiotic factors constantly alter the physiological activity of plants, which causes them to release various secondary metabolites in their environments. Volatile organic compounds (VOCs) are the most common cues that reflect a plant’s current physiological status. In this sense, the identity of its immediate neighbors may have the greatest impact for a plant, as they share the same available resources. Plants constantly monitor and respond to these cues with great sensitivity and discrimination, resulting in specific changes in their growth pattern and adjusting their physiology, morphology, and phenotype accordingly. Those typical competition responses in receivers may increase their fitness as they can be elicited even before the competition takes place. Plant–plant interactions are dynamic and complex as they can include many different and important surrounding cues. A major challenge for all individual plants is detecting and actively responding only to “true” cues that point to real upcoming threat. Such selective responses to highly specific cues embedded in volatile bouquets are of great ecological importance in understanding plant–plant interactions. We have reviewed recent research on the role of VOCs in complex plant–plant interactions in plant-cross kingdom and highlighted their influence on organisms at higher trophic levels.
Introduction
In nature, plants are members of complex and dynamic communities, where they experience a variety of neighbors of different identity. Coexistence with other plants is a constant challenge for individual plants and characterized by fierce confrontation over available resources. The wide range of interactions between plants in these communities determine species’ coexistence and performance, as well as community organization.Citation1,Citation2 Plants perceive their neighbors through different kinds of cues that indicate their proximity, such as light quality,Citation3,Citation4 root chemicals,Citation5,Citation6 acoustic cues,Citation7 mechanical stimuliCitation8, and airborne volatile organic compounds (VOCs).Citation9 The constantly present chemical cues that plants sense force them to distinguish between essential one predicting competitive neighbors from cues that are not crucial for their own fitness.Citation10 Although the mechanisms with which plants can accurately detect and distinguish a variety of cues from their neighbors by relevant gradients remain unknown, the advantage of this perception is self-evident, as it could provide valuable advance information about the presence of future competition.Citation11 As the occurrence of neighbors can differ over space and time, plants have evolved the ability to detect their presence in a timely manner.Citation12 In response to chemical cues that point out nearby competitors, a single plant can exhibit a multitude of adaption responses including physiological and morphological changes or resource allocation to optimize their performance.Citation13–Citation16 Those plant responses to potential competitor are not fixed due to their dependence on various environmental conditions. In other words, plants can have highly specific responses to the proximity of their neighbor under one set of conditions, but not under another set.Citation17 In this review, we present the role of VOCs produced by plants in response to various abiotic and biotic factors in interactions between plants taking into consideration the implications of these interactions on some organisms across different trophic levels.
Volatile-mediated chemical interactions
For every individual plant, the production, storage, and release of secondary metabolites are essential components of their phenotype as they influence important ecological functions.Citation18 Each plant species is capable of synthesizing a unique set of secondary metabolites that depends on a diverse set of environmental conditions under which plants grow.Citation19,Citation20 Plants are known to synthesize and emit in significant amounts more than 1700 VOCs, which conveys detailed information about their identity and physiological condition.Citation21 Fast-growing plants are considered to emit more VOCs due to their high physiological activity.Citation22 Plants in an early stage of development produce and release more VOCs.Citation23 VOCs can serve as external signals in within-plant communication providing an advantage to the producer but also as cues that can induce responses in nearby plants.Citation24 From the receiver’s perspective, VOCs represent the fastest and most reliable cues in plant neighbor detectionCitation25 since they are constantly present compared to periodical cues such as light, mechanical contact, or acoustic cues. In this case, any other cues received may provide additional information or extra confirmation about potential upcoming challenges. Some VOCs with higher volatility such as isoprene, methanol, phytohormone ethylene, and some monoterpenes are limited to plant interactions at shorter distances, while heavier compounds with less volatility, like terpene, methyl jasmonate (MeJA), methyl salicylate (MeSA), or green leaf volatiles, can transfer cues at longer distances.Citation26 Many of these VOCs are emitted constitutively in bouquets in which the ratio of compounds in that bouquet is often considered to be driven by species taxonomy with an amplitude of differentiation among species.Citation27 Such fluctuating and species-specific ratios between individual compounds released in a bouquet can determine plant–plant interactions and thus prevent or reduce the risk of eavesdropping by other species.Citation28
The ecological relevance of VOCs in plant–plant communications is limited and can be effective only at shorter distances due to their rapid dilution in the air to inactive concentrations.Citation29,Citation30 The sensitivity of the receiver to specific volatile bouquets or single compounds at different concentrations, coupled with the duration of exposure, makes plant responses much more complex. For instance, periodic exposure during three weeks to trace amounts of (Z)-3-hexenol and (Z)-3-hexenyl acetate emitted by damaged plants can induce defense responses in nearby neighborsCitation31 ((2)). In another study, airborne (Z)-3-hexenol produced by infested tomato plants was involved in the synthesis of (Z)-3-hexenylvicianoside (HexVic) in exposed conspecific plants that protect them against herbivores.Citation32 The resistance of lima bean to a bacterial pathogen was significantly enhanced after the exposure to nonanal for 6 and over 24 h, whereas this effect was only observed after 24 h when exposed to MeSA.Citation33 These findings indicate that plant interactions by specific VOCs also involve accumulations of volatiles up to certain quantity in receivers to induce defense response. Apart from active response to VOCs, plants like Betula spp. and broccoli can adsorb ledene, ledol, and palustrol produced by Rhododendron tomentosum and then re‐release, making them less attractive to herbivore insectsCitation34,Citation35 ((3)).
Figure 1. Volatiles from neighboring plants can have informative value for a receiver plant about the presence of (1) plants of the same species, (2) herbivore-infested plants of the same species, (3) plants of another species, (4) herbivore-infested plants of another species, (5) plants exposed to low red:far-red light, (6) highly competitive plants (weeds), (7) mechanically stimulated plants, and (8) root VOCs.
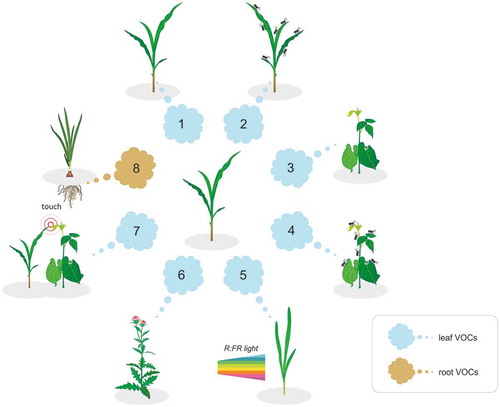
The emission of highly specific VOCs allows the identification of, and response to, cues from the same species or even relatives.Citation36 In that way, nearby plants can exploit volatile cues about the presence of specific neighbors, inducing growth responses that increase their competitiveness.Citation26,Citation30,Citation37
Roots can also synthesize and release VOCs in the rhizosphere which can play an important role in mediating belowground plant–plant interactions.Citation38,Citation39 Plants also use these belowground cues to identify the presence of their neighborsCitation40 and prepare for competition with a non-self neighborCitation41 or avoid competitors' rootsCitation42 ((8)). The presence of a specific neighbor can be an important factor in belowground plant–insect interaction as well. For instance, exposure to root VOCs released from Centaurea stoebe induces changes in Taraxacum officinale and makes it more susceptible to the larvae of Melolontha melolontha.Citation43 Secondary metabolites released by roots like benzoxazinoids can interfere with root-associated fungal and bacterial communities, reducing plant growth but increasing defenses against herbivores in the next generation of plants.Citation44 Plant root volatiles are also known to modify soil microbial community and thus have an important feedback that determines growth and defense.Citation45,Citation46 Microorganisms also alter the emission of plant volatiles.Citation47,Citation48 However, it is still unknown whether these altered variations in root VOCs may have implications on plant–plant interactions and further effects on other trophic levels.
The above-mentioned examples show that individual plant growth strategies in plant communities are not exclusively formed by resource limitations as suggestedCitation49,Citation50 but also by chemical interactions among plants. Thus, volatile interactions among plants should not be ignored in plant community theory because they have various consequences such as compensatory growth and increased resource uptake.Citation12
Plant volatiles in allelobiotic interactions
To describe a wider trophic effect of chemical interactions between plants, the term allelobiosis was introduced.Citation51 Allelobiosis is defined as beneficial interactions between undamaged plants, which may affect organisms at other trophic levels, such as herbivores and/or their natural enemies.Citation52 Undamaged plants constantly release VOCs that can be exploited by con- or hetero-specific neighbors. For instance, barley cultivar Kara exposed to VOCs from another cultivar Alva ((1)) allocated more biomass from shoots to roots than unexposed plants or Kara exposed to VOCs of another Kara.Citation16 The aforementioned response enhances the competitive ability of the exposed plants because resource allocation to root biomass may contribute to the fitness by facilitating higher nutrient uptake, especially in habitats characterized by low productivity.Citation53 The exposure of one barley cultivar to VOCs from another cultivar in laboratory experiments reduced aphid acceptance, but only in specific emitter and receiver combinations.Citation54 Cultivar combinations that affected aphid plant acceptance resulted also in significantly decreased aphid population development in the field, suggesting that volatile interactions also occurred under field conditions and that herbivore response was dependent on the identity of the neighboring cultivar.Citation55
Plant allelobiotic responses were observed in the volatile interaction between plants of different species ((3)). Potato exposed to onion released significantly higher quantities of (E)-nerolidol and (3E,7E)-4,8,12-Trimethyltrideca-1,3,7,11-tetraene (TMTT) repelling aphids in laboratory experiments and resulting also in significantly reduced aphid abundance in the field.Citation56 This type of VOC-mediated plant interactions is specific and depends on the plant species involved. For instance, volatile chemical interactions between different weed species and barley ((6)) only affected aphid plant acceptance after exposure of the two weed species Cirsium arvense and Cirsium vulgare and not when exposed to 16 other weed species.Citation57,Citation58
Volatile interactions between undamaged plants have also been shown to affect herbivores' natural enemies. The odour of potato previously exposed to onion VOCs became more attractive to ladybirds than unexposed potato, indicating that volatile communication between plants may contribute to increased abundance of natural enemies in complex plant habitats.Citation59 This is in line with the study of Ninkovic and Pettersson,Citation60 who found a higher frequency of ladybirds in barley plots containing high densities of weeds such as thistle (Cirsium arvense) and couch grass (Elytrigia repens) than in control plots only with barley. This was supported by laboratory studies in which barley plants exposed to VOCs released by thistles were more attractive to ladybirds in olfactory experiments than unexposed barley plants but not after exposure with couch grass.Citation60 Ladybirds preferred specific cultivar combinations over others used in the field, which occurred even before aphids arrived in the field and again when aphids were emigrating.Citation61 Higher olfactory attractiveness of ladybirds to specific cultivar combinations in the field and laboratory suggests that plant–plant volatile interaction is an underlying mechanism of ladybird’s habitat preference.Citation61 These studies show that volatile communication between undamaged plants affects not only the plants themselves but can have wider trophic effects on herbivore insects and their natural enemies. However, these interactions seem to occur only in specific combinations of emitter and receiver both within and between species.
Stress-induced plant volatiles
Both abiotic and biotic stress factors, such as high temperatures, high light intensity, or herbivore attack, may have increased VOC emissions as a consequenceCitation62 and alter emission patterns, which can also be species specific. Alternation in the VOC bouquet is frequently associated with stimulating or suppressing the emissions that can be induced in a systemic wayCitation63 by wounding, herbivore feeding, or after environmental stresses.Citation64–Citation67 These herbivore-induced plant volatiles (HIPVs) can stimulate neighbors to adjust their defenses at the right time, which subsequently reduces herbivore feeding damageCitation68–Citation70 ((2) and (4)) and increases their attractiveness to carnivores by providing them with biologically relevant information about the presence of their prey.Citation71,Citation72 The presence of a neighbor plant with a specific identity can drastically increase the emission of HIPVs of a focal species.Citation73
The emission of induced VOCs from plants is known to increase at high temperatures, which has been observed for different terpenes, including isoprene,Citation74 monoterpenes, and sesquiterpenes.Citation75 Increased temperatures due to climate change might accumulate VOCs such as sesquiterpenes and MeSA because of their low volatility, which could serve as a new mode of defense against herbivores or pathogens.Citation62 For example, the high light intensity increased the emission rates of β-caryophyllene.Citation76 Salt-induced VOC emissions include terpenes such as isoprene, mono- and sesquiterpenes, lipoxygenase (LOX) products, and methanol from Arabidopsis plants, which are relevant in priming stress tolerance and triggering induction of high salt resistance in neighboring plants.Citation77 Relative growth rate, stomatal conductance, and photosynthetic rate were significantly higher in exposed plants, making them better prepared for upcoming salt stress.Citation78
VOC as cues in indication of complex interaction between neighboring plants
Volatile cues in a plant’s environment can initiate physiological changes, altering their own volatile profile, which in turn carry information for other proximate plants that can respond to it, reflecting the complexity of plant–plant–plant interactions. Thus, receivers can respond to these cues in completely different ways and adapt to neighbors’ presence depending on their response to nearby plants. For instance, it has been shown that barley Kara plants allocate more biomass to root when exposed to the Alva variety.Citation16 However, when emitter Alva plants were exposed to low red:far red light, they significantly reduced the emission of (E)-β-caryophyllene, α-humulene, and caryophyllene oxideCitation3 ((5)). Such modified volatile emission of Alva induced a typical shade avoidance response in exposed Kara plants grown in normal light, which allocated more resources into aboveground biomass than to roots.
Mechanical stimuli are one of the most common cues by which plants can rapidly detect the presence of neighbors and respond by adjusting their growth; these include inhibition of inflorescence elongation which makes them stronger and more resistant to damage.Citation79 Touch between plants can induce changes in their volatile emissionsCitation80 that can have further impact on neighbors in their proximity. A recent study demonstrated that within minutes in response to brief and light contact, maize plants trigger transcripts of touch- and defense-related genes and consequently rapidly change emission of isoprene fragment, C4 aldehydes fragment, and (E)-3-hexenal and terpene fragments.Citation81 One-minute treatment per day over a period of 6 d triggered a slower but longer increase in emission of methanol, isoprene fragment, isoprene, alkyl fragment, and terpene fragment. The complex series of coordinated defense responses expressed in neighbors exposed to these volatiles leads to the activation of the same touch- and defense-related genes (transcriptional mirroring effect), making these plants less suitable hosts for aphidsCitation81 ((7)). Surprisingly, aboveground touch stimuli can also modify belowground plant–plant communication, revealing a new dimension to the functional role of touch induce cues in plant interactions at the community level.Citation82 This point out on a new level of complexity in belowground plant–plant interactions, showing that the direction and extent of plant root responses to neighbors can be affected by the aboveground touch interaction to which neighbors are exposed. The extraordinary specific response pattern made by the exposed plant could be difficult to explain if we consider the variety, quantity, and quality of competing cues perceived (VOCs, root exudates, brief mechanical contact), taking into account their interactions with the complex mixture of biotic and abiotic factors.
The aforementioned examples indicate that plant volatiles have important informative value about the physiological status of the emitters that are exposed to complex interactions with their neighbors (). Thus, volatiles can differentially modulate plant growth responses of the same neighbor depending on their response to different biotic stressors or interference mechanisms. The exposed plant can use such VOCs carrying specific cues in the detection of forthcoming competitive neighbors, as reflected in their mirroring response that occurred even before the competition took place.
Figure 2. Volatile cues in network plant interactions. Exposure to volatile cues from emitter (E0) reveals their physiological status and change volatile emission of receiver 1 (R1) which becomes emitter 1 (E1) reflecting through specific volatiles their own responses to the presence of E0 plants further to receiver 2 (R2).
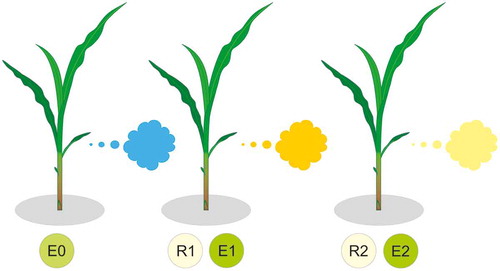
Conclusions and future perspectives
Recent studies on plant responses to the diversity of volatile cues received from neighbors have demonstrated their capacity to rapidly modify growth strategies to meet various ecological challenges. Possible forthcoming threats converted into volatile cues showed to have important ecological role preparing responding plants for future stressors that also influence interactions at higher trophic levels. The fact that volatile cues can initiate physiological changes in receivers, altering their own volatile profile, which in turn carry information for other proximate plants, reflects the complexity of cascading plant–plant interactions (). Effective chemical interaction among plants is an important functional component in nature but also in agricultural systems where it could be widely exploited. Highly specific volatile cues that activate adaption responses and induce defense in exposed plants represent an important scientific research area in the future and should be considered when studying plant responses either to biotic or abiotic stresses.
Author Contributions
All authors contributed to the review through the development of ideas, drafting of the initial text, and providing feedback for revisions. DM prepared the figures.
Disclosure of Potential Conflicts of Interest
No potential conflicts of interest were disclosed.
Additional information
Funding
References
- Thorpe AS, Thelen GC, Diaconu A, Callaway RM. Root exudate is allelopathic in invaded community but not in native community: field evidence for the novel weapons hypothesis. J Ecol. 2009;97:641–645. doi:10.1111/j.1365-2745.2009.01520.x.
- Bruno JF, Stachowicz JJ, Bertness MD. Inclusion of facilitation into ecological theory. Trends Ecol Evol. 2003;18:119–125. doi:10.1016/S0169-5347(02)00045-9.
- Kegge W, Ninkovic V, Glinwood R, Welschen RAM, Voesenek LACJ, Pierik R. Red:far-red light conditions affect the emission of volatile organic compounds from barley (Hordeum vulgare), leading to altered biomass allocation in neighbouring plants. Ann Bot. 2015;115:961–970. doi:10.1093/aob/mcv036.
- Gruntman M, Groß D, Májeková M, Tielbörger K. Decision-making in plants under competition. Nat Commun. 2017;8. doi:10.1038/s41467-017-02147-2.
- Biedrzycki ML, Jilany TA, Dudley SA, Bais HP. Root exudates mediate kin recognition in plants. Commun Integr Biol. 2010;3:28–35. doi:10.4161/cib.3.1.10118.
- Delory BM, Delaplace P, Fauconnier ML, Du Jardin P. Root-emitted volatile organic compounds: can they mediate belowground plant-plant interactions? Plant Soil. 2016;402:1–26. doi:10.1007/s11104-016-2823-3.
- Gagliano M, Grimonprez M, Depczynski M, Renton M. Tuned in: plant roots use sound to locate water. Oecologia. 2017;184:151–160. doi:10.1007/s00442-017-3862-z.
- Markovic D, Nikolic N, Glinwood R, Seisenbaeva G, Ninkovic V. Plant responses to brief touching: a mechanism for early neighbour detection? PLoS One. 2016;11:e0165742. doi:10.1371/journal.pone.0165742.
- Ninkovic V, Markovic D, Dahlin I. Decoding neighbour volatiles in preparation for future competition and implications for tritrophic interactions. Perspect Plant Ecol Evol Syst. 2016;23:11–17. doi:10.1016/j.ppees.2016.09.005.
- Baldwin IT. Plant volatiles. Curr Biol. 2010;20:392–397. doi:10.1016/j.cub.2010.02.052.
- Shemesh H, Ovadia O, Novoplansky A. Anticipating future conditions via trajectory sensitivity. Plant Signal Behav. 2010;5:1501–1503. doi:10.4161/psb.5.11.13660.
- Callaway RM, Pennings SC, Richards CL. Phenotypic plasticity and interactions among plants. Ecology. 2003;84:1115–1128. doi:10.1890/0012-9658(2003)084[1115:PPAIAP]2.0.CO;2.
- Pierik R, Mommer L, Voesenek LA. Molecular mechanisms of plant competition: neighbour detection and response strategies. Funct Ecol. 2013;27:841–853. doi:10.1111/1365-2435.12010.
- Violle, C., Garnier, E., Lecoeur, J., Roumet, C., Podeur, C., Blanchard, A., and Navas, M. L. Competition, traits and resource depletion in plant communities. Oecologia. 2009;160:747–755. doi:10.1007/s00442-009-1333-x.
- Yamawo A, Tagawa J, Hada Y, Suzuki N. Different combinations of multiple defence traits in an extrafloral nectary-bearing plant growing under various habitat conditions. J Ecol. 2014;102:238–247. doi:10.1111/1365-2745.12169.
- Ninkovic V. Volatile communication between barley plants affects biomass allocation. J Exp Bot. 2003;54:1931–1939. doi:10.1093/jxb/erg192.
- Callaway RM. Positive interactions among plants. Bot Rev. 1995;61:306–349. doi:10.1093/sysbio/syr125.
- Metlen KL, Aschehoug ET, Callaway RM. Plant behavioural ecology: dynamic plasticity in secondary metabolites. Plant Cell Environ. 2009;32:641–653. doi:10.1111/j.1365-3040.2008.01910.x.
- Sampaio BL, Edrada-ebel R, Batista F, Costa D. Effect of the environment on the secondary metabolic profile of Tithonia diversifolia: a model for environmental metabolomics of plants. Nat Publ Gr. 2016;1–11. doi:10.1038/srep29265.
- Kessler A, Kalske A. Plant secondary metabolite diversity and species interactions. Annu Rev Ecol Evol Syst. 2018;49:115–138. doi:10.1146/annurev-ecolsys-110617-062406.
- Dicke M. Herbivore-induced plant volatiles as a rich source of information for arthropod predators: fundamental and applied aspects. J Indian Inst Sci. 2015;95:35–42.
- Loreto F, Dicke M, Schnitzler J, Turlings TCJ. Plant volatiles and the environment. Plant Cell Environ. 2014;37:1905–1908. doi:10.1111/pce.12369.
- Dudareva N, Klempien A, Muhlemann JK, Kaplan I. Biosynthesis, function and metabolic engineering of plant volatile organic compounds. New Phytol. 2013;198:16–32. doi:10.1111/nph.12145.
- Heil M, Silva Bueno JC. Within-plant signaling by volatiles leads to induction and priming of an indirect plant defense in nature. Proc Natl Acad Sci USA. 2007;104:5467–5472. doi:10.1073/pnas.0610266104.
- Dicke M, Loreto F. Induced plant volatiles: from genes to climate change. Trends Plant Sci. 2010;15:115–117. doi:10.1016/j.tplants.2010.01.007.
- Baldwin I, Halitschke R, Paschold A, von Dahl C, Preston C. Volatile signaling in plant-plant interactions: “talking trees” in the genomics era. Science. 2006;311:812–816. doi:10.1126/science.1118446.
- Bruce TJA, Wadhams LJ, Woodcock CM. Insect host location: a volatile situation. Trends Plant Sci. 2005;10:269–274. doi:10.1016/j.tplants.2005.04.003.
- Ueda H, Kikuta Y, Matsuda K. Plant communication: mediated by individual or blended VOCs? Plant Signal Behav. 2012;7:222–226. doi:10.4161/psb.18765.
- Preston CA, Laue G, Baldwin IT. Methyl jasmonate is blowing in the wind, but can it act as a plant–plant airborne signal? Biochem Syst Ecol. 2001;29:1007–1023. doi:10.1016/S0305-1978(01)00047-3.
- Heil M, Karban R. Explaining evolution of plant communication by airborne signals. Trends Ecol Evol. 2010;25:137–144. doi:10.1016/j.tree.2009.09.010.
- Shiojiri K, Ozawa R, Matsui K, Sabelis MW, Takabayashi J. Intermittent exposure to traces of green leaf volatiles triggers a plant response. Sci Rep. 2012;2:1–5. doi:10.1038/srep00378.
- Sugimoto K, Matsui K, Iijima Y, Akakabe Y, Muramoto S, Ozawa R. Intake and transformation to a glycoside of (Z)-3-hexenol from infested neighbors reveals a mode of plant odor reception and defense. Proc Natl Acad Sci USA. 2014;111:7144–7149. doi:10.1073/pnas.1320660111.
- Girón-Calva PS, Molina-Torres J, Heil M. Volatile dose and exposure time impact perception in neighboring plants. J Chem Ecol. 2012;38:226–228. doi:10.1007/s10886-012-0072-3.
- Himanen SJ, Bui TNT, Maja MM, Holopainen JK. Utilizing associational resistance for biocontrol: impacted by temperature, supported by indirect defence. BMC Ecol. 2015;15:1–12. doi:10.1186/s12898-015-0048-6.
- Himanen SJ, Blande JD, Klemola T, Pulkkinen J, Heijari J, Holopainen JK. Birch (Betula spp.) leaves adsorb and re-release volatiles specific to neighbouring plants – a mechanism for associational herbivore resistance? New Phytol. 2010;186:722–732. doi:10.1111/j.1469-8137.2010.03220.x.
- Karban R, Wetzel WC, Shiojiri K, Ishizaki S, Ramirez SR, Blande JD. Deciphering the language of plant communication: volatile chemotypes of sagebrush. J Physiol. 2014a;204:380–385. doi:10.1111/nph.12887.
- Dicke M, van Poecke RMP, de Boer JG. Inducible indirect defence of plants: from mechanisms to ecological functions. Basic Appl Ecol. 2003;4:27–42. doi:10.1078/1439-1791-00131.
- Jassbi AR, Zamanizadehnajari S, Baldwin IT. Phytotoxic volatiles in the roots and shoots of Artemisia tridentata as detected by headspace solid-phase microextraction and gas chromatographic-mass spectrometry analysis. J Chem Ecol. 2010;36:1398–1407. doi:10.1007/s10886-010-9885-0.
- Peñuelas, J., Asensio, D., Tholl, D., Wenke, K., Rosenkranz, M., Piechulla, B., and Schnitzler, J. P. Biogenic volatile emissions from the soil. Plant Cell Environ. 2014;37:1866–1891. doi:10.1111/pce.12340.
- Depuydt S. Arguments for and against self and non-self root recognition in plants. Front Plant Sci. 2014;5:1–7. doi:10.3389/fpls.2014.00614.
- Gruntman M, Novoplansky A. From the cover: physiologically mediated self/non-self discrimination in roots. Proc Natl Acad Sci. 2004;101:3863–3867. doi:10.1073/pnas.0306604101.
- Schmid C, Bauer S, Bartelheimer M. Should I stay or should I go? Roots segregate in response to competition intensity. Plant Soil. 2015;391:283–291. doi:10.1007/s11104-015-2419-3.
- Huang W, Zwimpfer E, Hervé MR, Bont Z, Erb M. Neighbourhood effects determine plant–herbivore interactions below-ground. J Ecol. 2018;106:347–356. doi:10.1111/1365-2745.12805.
- Hu, L., Robert, C. A. M., Cadot, S., Zhang, X., Ye, M., Li, B., Manzo, D., Chervet, N., Steinger, T., Van Der Heijden, M. G., et al. Root exudate metabolites drive plant-soil feedbacks on growth and defense by shaping the rhizosphere microbiota. Nat Commun. 2018;9:1–13. doi:10.1038/s41467-018-05122-7.
- Bever JD, Platt TG, Morton ER. Microbial population and community dynamics on plant roots and their feedbacks on plant communities. Annu Rev Microbiol. 2012;66:265–283. doi:10.1146/annurev-micro-092611-150107.
- Wenke K, Kai M, Piechulla B. Belowground volatiles facilitate interactions between plant roots and soil organisms. Planta. 2010;231:499–506. doi:10.1007/s00425-009-1076-2.
- Junker RR, Tholl D. Volatile organic compound mediated interactions at the plant-microbe interface. J Chem Ecol. 2013;39:810–825. doi:10.1007/s10886-013-0325-9.
- Sharifi R, Lee S, Ryu C. Microbe-induced plant volatiles. New Phytol. 2018;220:684–691. doi:10.1111/nph.14955.
- Tilman D. The resource-ratio hypothesis of plant succession. Am Nat. 1985;125:827–852. doi:10.1086/284382.
- Grime JP. Plant strategies and vegetation processes. Chichester-New York-Brisbane-Toronto: John Wiley & Sons, Ltd. 1979.
- Pettersson J, Ninkovic V, Glinwood R. Plant activation of barley by intercropped conspecifics and weeds: allelobiosis. Bcpc Int Congr Crop Sci Technol. 2003;1(2). Congr. Proc. 2003. p. 1135–1144.
- Ninkovic V, Glinwood R, Pettersson J. Communication between undamaged plants by volatiles: the role of allelobiosis. Commun Plants Neuronal Asp Plant Life. 2006;421–434. doi:10.1007/978-3-540-28516-8_28.
- Tilman D. On the meaning of competition and the mechanisms of competitive superiority. Funct Ecol. 1987;1:304–315. doi:10.2307/2389785.
- Kellner M, Brantestam AK, Åhman I, Ninkovic V. Plant volatile-induced aphid resistance in barley cultivars is related to cultivar age. Theor Appl Genet. 2010;121:1133–1139. doi:10.1007/s00122-010-1377-7.
- Dahlin I, Rubene D, Glinwood R, Ninkovic V. Pest suppression in cultivar mixtures is influenced by neighbor-specific plant-plant communication. Ecol Appl. 2018;28:2187–2196. doi:10.1002/eap.1807.
- Ninkovic V, Dahlin I, Vucetic A, Petrovic-Obradovic O, Glinwood R, Webster B. Volatile exchange between undamaged plants – a new mechanism affecting insect orientation in intercropping. PLoS One. 2013;8:e69431. doi:10.1371/journal.pone.0069431.
- Ninkovic V, Glinwood R, Dahlin I. Weed-barley interactions affect plant acceptance by aphids in laboratory and field experiments. Entomol Exp Appl. 2009;133:38–45. doi:10.1111/j.1570-7458.2009.00900.x.
- Glinwood R, Ninkovic V, Pettersson J, Ahmed E. Barley exposed to aerial allelopathy from thistles (Cirsium spp.) becomes less acceptable to aphids. Ecol Entomol. 2004;29:188–195. doi:10.1111/j.0307-6946.2004.00582.x.
- Vucetic A, Dahlin I, Petrovic-Obradovic O, Glinwood R, Webster B, Ninkovic V. Volatile interaction between undamaged plants affects tritrophic interactions through changed plant volatile emission. Plant Signal Behav. 2014;9:7–12. doi:10.4161/psb.29517.
- Ninkovic V, Pettersson J. Searching behaviour of the sevenspotted ladybird, Coccinella septempunctata– effects of plant-plant odour interaction. Oikos. 2003;100:65–70. doi:10.1034/j.1600-0706.2003.11994.x.
- Ninkovic V, Abassi S, Al Ahmed E, Glinwood R, Pettersson J. Effect of within-species plant genotype mixing on habitat preference of a polyphagous insect predator. Oecologia. 2011;166:391–400. doi:10.1007/s00442-010-1839-2.
- Holopainen JK, Gershenzon J. Multiple stress factors and the emission of plant VOCs. Trends Plant Sci. 2010;15:176–184. doi:10.1016/j.tplants.2010.01.006.
- Loreto F, Barta C, Brilli F, Nogues I. On the induction of volatile organic compound emissions by plants as consequence of wounding or fluctuations of light and temperature. Plant Cell Environ. 2006;29:1820–1828. doi:10.1111/j.1365-3040.2006.01561.x.
- Asai T, Matsukawa T, Kajiyama S. Metabolic changes in citrus leaf volatiles in response to environmental stress. J Biosci Bioeng. 2016;121:235–241. doi:10.1016/j.jbiosc.2015.06.004.
- Unsicker SB, Kunert G, Gershenzon J. Protective perfumes: the role of vegetative volatiles in plant defense against herbivores. Curr Opin Plant Biol. 2009;12:479–485. doi:10.1016/j.pbi.2009.04.001.
- Yamauchi Y, Kunishima M, Mizutani M, Sugimoto Y. Reactive short-chain leaf volatiles act as powerful inducers of abiotic stress-related gene expression. Sci Rep. 2015;5:8030. doi:10.1038/srep08030.
- Danner H, Desurmont GA, Cristescu SM, van Dam NM. Herbivore-induced plant volatiles accurately predict history of coexistence, diet breadth, and feeding mode of herbivores. New Phytol. 2017;220:726–738. doi:10.1111/nph.14428.
- Karban R, Yang LH, Edwards KF. Volatile communication between plants that affects herbivory: a meta-analysis. Ecol Lett. 2014b;17:44–52. doi:10.1111/ele.12205.
- Zakir A, Sadek MM, Bengtsson M, Hansson BS, Witzgall P, Anderson P. Herbivore-induced plant volatiles provide associational resistance against an ovipositing herbivore. J Ecol. 2013;101:410–417. doi:10.1111/1365-2745.12041.
- Dudareva N, Negre F, Nagegowda DA, Orlova I. Plant volatiles: recent advances and future perspectives. CRC Crit Rev Plant Sci. 2006;25:417–440. doi:10.1080/07352680600899973.
- Dicke M, Baldwin IT. The evolutionary context for herbivore-induced plant volatiles: beyond the “cry for help.”. Trends Plant Sci. 2010;15:167–175. doi:10.1016/j.tplants.2009.12.002.
- van Wijk M, de Bruijn PJA, Sabelis MW. Complex odor from plants under attack: herbivore’s enemies react to the whole, not its parts. PLoS One. 2011;6:e21742. doi:10.1371/journal.pone.0021742.
- Kigathi RN, Weisser WW, Reichelt M, Gershenzon J, Unsicker SB. Plant volatile emission depends on the species composition of the neighboring plant community. BMC Plant Biol. 2019;19:1–17. doi:10.1186/s12870-018-1541-9.
- Sharkey TD, Yeh S. Isoprene emission from plants. Annu Rev Plant Physiol Plant Mol Biol. 2001;52:407–436. doi:10.1146/annurev.arplant.52.1.407.
- Navarro, A., Smolander, S., Struthers, H., Zorita, E., Ekman, A. M. L., Kaplan, J. O., Guenther, A., Arneth, A. and Riipinen, I. Global emissions of terpenoid VOCs from terrestrial vegetation in the last millennium. J Geophys Res Atmos. 2014;119:6867–6885. doi:10.1002/2013JD021238.Received.
- Hansen U, Seufert G. Temperature and light dependence of β-caryophyllene emission rates. J Geophys Res Atmos. 2003;108. doi:10.1029/2003JD003853.
- Lee K, Seo PJ. Airborne signals from salt-stressed Arabidopsis plants trigger salinity tolerance in neighboring plants. Plant Signal Behav. 2014;9:3–6. doi:10.4161/psb.28392.
- Caparrotta S, Boni S, Taiti C, Palm E, Mancuso S, Pandolfi C. Induction of priming by salt stress in neighboring plants. Environ Exp Bot. 2018;147:261–270. doi:10.1016/j.envexpbot.2017.12.017.
- Braam J. In touch: plant responses to mechanical stimuli. New Phytol. 2005;165:373–389. doi:10.1111/j.1469-8137.2004.01263.x.
- Markovic D, Glinwood R, Olsson U, Ninkovic V. Plant response to touch affects the behaviour of aphids and ladybirds. Arthropod Plant Interact. 2014;8:171–181. doi:10.1007/s11829-014-9303-6.
- Markovic, D., Colzi, I., Taiti, C., Ray, S., Scalone, R., Gregory Ali, J., Mancuso, S. and Ninkovic, V. Airborne signals synchronize the defenses of neighboring plants in response to touch. J Exp Bot. 2019;70:691–700. doi:10.1093/jxb/ery375.
- Elhakeem A, Markovic D, Broberg A, Anten NPR, Ninkovic V. Aboveground mechanical stimuli affect belowground plant-plant communication. PLoS One. 2018;13:e0195646. doi:10.1371/journal.pone.0195646.