ABSTRACT
Electrical signaling, similar to chemical signalings such as calcium (Ca2+), reactive oxygen species (ROS, mainly hydrogen peroxide: H2O2), nitric oxide (NO), and hydrogen sulfide (H2S), regulates many physiological processes. However, the effect of electrical stimulation on seed germination, seedling growth, and thermotolerance improvement in maize was little known. In this study, using maize as materials, the effect of electrical stimulation on seed germination, seedling growth, and thermotolerance improvement in maize was explored. The results suggested that electrical stimulation with optimal intensity boosted germination rate and seedling growth (as indicated in the increase in the length of shoots and roots, as well as fresh weight) under normal germination conditions. In addition, electrical stimulation augmented the survival rate of maize seedlings, mitigated the decrease in the tissue vitality, and reduced the peroxidation of membrane lipids under heat stress. These data suggested that electrical stimulation could boost seed germination, seedling growth, and thermotolerance improvement in maize.
Electrical signaling widely exists in plant and animal body, which can be classified into three types according to different spatial patterns, that is, action potential, variation potential, and system potential.Citation1–Citation5 Electrical signaling endogenously generated or exogenously applied, similar to chemical signalings such as calcium (Ca2+), reactive oxygen species (ROS, mainly hydrogen peroxide: H2O2), nitric oxide (NO), and hydrogen sulfide (H2S), regulates many biological processes at molecular, cellular, physiological, and biochemical levels.Citation1–Citation5 Endogenous electrical signaling can be triggered by environmental stress, such as heat, cold, touching, pricking, burning, and crushing.Citation1–Citation6 Afterward, electrical signaling regulates physiological (stem growth, leaf movement, respiration, transpiration, and stomatal opening), biochemical [synthesis of proteins, hormones (abscisic acid: ABA; jasmonic acid: JA), protein kinase inhibitors (pin2), and secondary metabolites], and molecular (gene expression of enzymes related to carbon metabolism and ribosome proteins) processes.Citation1–Citation6
Exogenous electrical stimulation is an efficient and environmentally friendly technique, which regulates plant growth and development by modifying cellular metabolism via interaction with hydraulic, chemical (Ca2+, ROS), and hormone signaling (ABA and JA) in plants.Citation1–Citation4,Citation7 In many plant species (Triticum aestivum, Solanum lycopersicum, Raphanus sativus, and Hordeum vulgare, Avena sativa, and Hordeum vulgare), the positive and negative effects of electrical stimulation on seed germination, crop yield, and fruit quality have been observed.Citation1–Citation4,Citation8 In addition, more interestingly, electrical stimulation also can improve plant tolerance to salt and freezing stress in wheat and Cucurbita pepo, respectively.Citation2,Citation9 However, it is little known that whether electrical stimulation could boost seed germination, seedling growth, and the production of thermotolerance in maize. Therefore, in this study, the food, feed, and energy crop maize (Zea mays L., Xuanheyu No 2) as materials, the effect of electrical stimulation on seed germination, seedling growth, and thermotolerance improvement was studied. The aim was to interpret the motivating effect of electrical stimulation on seed germination, seedling growth, and the development of thermotolerance in maize.
The uniform and healthy maize seeds (purchased from Yiliang Seed Company, China) were sterilized with 5% (w/v) sodium hypochlorite solution for 15 min and then rinsed with distilled water. The rinsed seeds were immersed in distilled water at 26°C for 12 h. Afterward, the watered seeds were sown in trays (22 cm × 15 cm, 200 seeds per tray) with eight-layer filter paper, and then moistened with 100 mL of 1/2 MS nutrient solution. To carry out electrical stimulation, an end of the wire was linked with the anode of electrophoresis apparatus, and the other end was inserted into the narrow end of trays to touch the moistened filter paper; the two ends of another wire were linked with the cathode of apparatus and the another narrow end of the trays, respectively. Afterward, the voltage of apparatus was adjusted to 22 V per tray (namely 1 V/cm, 3 mA per tray. The appropriate voltage was screened from preliminary experiments with 11, 22, and 33 V). Electrical stimulation was performed at 22 V for 0, 5, 10, and 15 min (once per 20-h), and then germinated in a climate chamber at 26 °C in dark for 60 h (namely 2.5 d). On the 2.5 d, the germination rate, the length of shoots and roots, and the fresh weight were measured according to the methods of Wang.Citation10 The experiments were at least repeated threetimes and two parallel replications in each time. The results showed that, compared with the control without electrical stimulation, electrical stimulation increased the germination rate of maize seeds, and this increase was enhanced with increasing time of electrical stimulation, reaching the significant level at 22 V for 10 min (P ˂ .05, )). However, a higher intensity of electrical stimulation (22 V for 15 min) reduced the germination rate and exhibited a significant difference compared to the control (P ˂ .05, )). Similar results have been observed in wheat, barley, radish, and tomato.Citation4 These data indicated that electrical stimulation could improve seed germination. For seedling growth, similar to the change in germination rate ()), compared with the control, the length of shoots and roots, as well as the fresh weight, were also elevated by electrical stimulation. The elevation was strengthened along with the duration of time of electrical stimulation, indicating a significant facilitating effect at 22 V for 10 min (P ˂ .05, )). By contrast, seedling growth, that is, the length of shoots and roots, as well as the fresh weight, were decreased by a higher strength of electrical stimulation (22 V for 15 min), and exhibiting a significant inhibiting effect compared with the control (P ˂ .05), ()). The inhibitive effect of electrical stimulation might be related to the cell membrane disruption ()) and more accumulation of secondary metabolites.Citation4 Further experiments confirmed that electrical stimulation could trigger Ca2+ and ROS signaling by activating ion channels, which in turn increased the activities of peroxidase, catalase, and enzymes related to carbon metabolism, as well as the uptake of nutrients such as potassium, calcium, and magnesium.Citation4,Citation11 These might be reasons for electrical stimulation-boosted seed germination and seedling growth. In addition, seed germination and seedling growth also are closely associated with the enzymes related to reserve substances such as starches, proteins, and lipids.Citation12
Figure 1. Effect of electrical stimulation on seed germination (a, b), shoot length (c), root length (d), and fresh weight (e) under normal germination conditions, as well as survival rate (f, g), tissue vitality (h), and malondialdehyde (MDA) content (i) of maize seedlings under heat stress. To determine seed germination and seedling growth, the watered maize seeds were treated with electrical stimulation at 22 V for 0, 5, 10, and 15 min, respectively, once per 20 h, and then germinated at 26°C for 60 h. After germination, germination rate, shoot length, root length, and fresh weight were measured. To evaluate thermotolerance, after a 60-h germination, the 60-h-old maize seedlings treated with electrical stimulation above were exposed to heat stress at 47°C for 16 h. After heat stress, survival rate, tissue vitality, and MDA content were determined. The data in figure are the mean ± standard error (SE) (n = 6), significance was tested with Least Significant Difference (LSD), asterisk (*) and double asterisk (**) indicate significant difference (P˂ .05) and very significant difference (P ˂ 0.01) compared to the control without electrical stimulation, respectively.
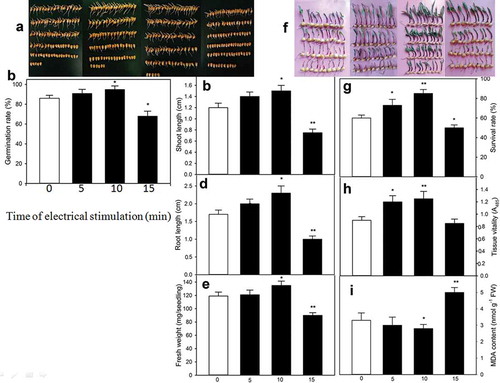
In order to further study the effect of electrical stimulation on the thermotolerance of maize seedlings, after electrical stimulation mentioned above, the 60-h-old seedlings were subjected to heat stress at 47°C for 16 h (the stress strength was screened from the preliminary experiments, which made the survival rate of maize seedlings reach 50%, namely half-lethal strength). After heat stress, the survival rate, tissue vitality, and the content of malondialdehyde were determined as per Wang.Citation10 The experiments were at least repeated threetimes and two parallel replications in each time. As shown in ), analog to the change in seed germination ()), compared with the control, the survival rate of maize seedlings was improved by electrical stimulation under heat stress. Also, the survival rate was further enhanced with the elongation of time of electrical stimulation within a given range, showing significant difference and very significant difference at 5 (P ˂ .05) and 10 min (P ˂ .01) of electrical stimulation at 22 V. In addition, for tissue vitality, similar to the trends of the change in survival rate, compared to the control, the decline in the tissue vitality also was relieved by electrical stimulation under heat stress. The relief was further enhanced with the duration of time of electrical stimulation within a certain range, reaching significant and very significant levels at 5 (P ˂ .05) and 10 min (P ˂ .01) of electrical stimulation at 22 V ()). However, compared with the control, a significant difference in tissue vitality was not observed in maize seedlings treated with a higher intensity of electrical stimulation at 22 V for 15 min under heat stress ()). For the extent of peroxidation of membrane lipids, that is, the accumulation of malondialdehyde was also alleviated by electrical stimulation under heat stress, similar to the change in tissue vigor ()). As expected, the degree of peroxidation alleviation was strengthened along with the increasing time of electrical stimulation, exhibiting a significant mitigating effect at 22 V for 10 min (P ˂ .05, )). Unfortunately, the degree of membrane lipid peroxidation, namely the accumulation of malondialdehyde was deteriorated by a higher intensity of electrical stimulation (22 V for 15 min, )), indicating a negative effect. In pea (Pisum Sativum), electrical signals can stimulate the thermotolerance of photosystem I by decreasing CO2 assimilation and quantum yields.Citation13,Citation14 In addition, electrical signal-stimulated thermotolerance may associate with the activation of non-photochemical quenching and cyclic electron flow;Citation15 an increase of ATP content;Citation16,Citation17 changes in transpiration and leaf heating,Citation18 and the state transition in photosynthetic machinery.Citation19 Also, the development of thermotolerance induced by an electrical signal is involved in the crosstalk of signaling molecules such as Ca2+, reactive oxygen species, and plant hormones; expression of genes (calmodulin, proteinase inhibitors, Rubisco, and enzymes related to ABA and JA); as well as changes in phloem mass-flow.Citation1,Citation14,Citation20,Citation21
In summary, electrical stimulation boosted the percentage of seed germination, the length of shoots and roots, and the fresh weight of maize seedlings within a given range under normal germination conditions. In addition, electrical stimulation also boosted the percentage of survival of maize seedlings under heat stress, remitted the decline in the vigor of tissue, and weakened the degree of the peroxidation of membrane lipids in an intensity-dependent fashion. These results exhibited that electrical stimulation boosted the seed germination, seedling growth, and thermotolerance improvement in maize. Nevertheless, the exact physiological, biochemical, and molecular mechanism of electrical stimulation-boosted seed germination, seedling growth, and thermotolerance production in maize was waiting for further expounding in the future.
Disclosure of Potential Conflicts of Interest
No potential conflicts of interest were disclosed.
Additional information
Funding
References
- Sukhov V, Sukhova E, Vodeneev V. Long-distance electrical signals as a link between the local action of stressors and the systemic physiological responses in higher plants. Prog Biophys Mol Biol. 2019;146:1–4. doi:10.1016/j.pbiomolbio.2018.11.009.
- Sukhov V. Electrical signals as mechanism of photosynthesis regulation in plants. Photosynth Res. 2016;130:373–387. doi:10.1007/s11120-016-0270-x.
- Mikami M, Mori D, Masumura Y, Aoki Y, Suzuki S. Electrical stimulation: an abiotic stress generator for enhancing anthocyanin and resveratrol accumulation in grape berry. Sci Hort. 2017;226:285–292. doi:10.1016/j.scienta.2017.09.005.
- Dannehl D. Effects of electricity on plant responses. Sci Hort. 2018;234:382–392. doi:10.1016/j.scienta.2018.02.007.
- Stanković B, Davies E. Intercellular communication in plants: electrical stimulation of proteinase inhibitor gene expression in tomato. Planta. 1997;202:402–406. doi:10.1007/s004250050143.
- Pena-Cortes H, Fisahn J, Willmitzer L. Signals involved in wound-induced proteinase inhibitor II gene expression in tomato and potato plants. PNAS. 1995;92:4106–4113. doi:10.1073/pnas.92.10.4106.
- Yudaev I, Mashkov S, Vasilyev S, Syrkin V, Shevchenko S, Sirakov K. Improvement of technology of electrical and magnetic stimulation of seeds and crop plants. In: Kharchenko V, Vasant P, editors. Handbook of research on energy-saving technologies for environmentally-friendly agricultural development. Chicago (US): IGI Global; 2020. p. 365–396.
- Black JD, Forsyth FR, Fensom DS, Ross RB. Electrical stimulation and its effects on growth and ion accumulation in tomato plants. Canad J Bot. 1971;49:809–1815.
- Wang ZY, Qin XH, Li JH, Fan LF, Zhou Q, Wang YQ, Zhao X, Xie CJ, Wang ZY, Huang L. Highly reproducible periodic electrical potential changes associated with salt tolerance in wheat plants. Environ Exp Bot. 2019;160:120–130. doi:10.1016/j.envexpbot.2019.01.014.
- Wang Y, Ye XY, Qiu XM, Li ZG. Methylglyoxal triggers the heat tolerance in maize seedlings by driving AsA-GSH cycle and reactive oxygen species-/methylglyoxal-scavenging system. Plant Physiol Biochem. 2019;138:91–99. doi:10.1016/j.plaphy.2019.02.027.
- Li X, Liu X, Wan B, Li X, Li M, Zhu H, Hua H. Effects of continuous exposure to power frequency electric fields on soybean Glycine max. J Environ Rad. 2019;204:35–41. doi:10.1016/j.jenvrad.2019.03.026.
- Rajjou L, Duval M, Gallardo K, Catusse J, Bally J, Job C, Job D. Seed germination and vigor. Ann Rev Plant Biol. 2012;63:507–533. doi:10.1146/annurev-arplant-042811-105550.
- Sukhov V, Surova L, Sherstneva O, Vodeneev V. Influence of variation potential on resistance of the photosynthetic machinery to heating in pea. Physiol Plant. 2014;152:773–783. doi:10.1111/ppl.12208.
- Sukhov V, Gaspirovich V, Mysyagin S, Vodeneev V. High-temperature tolerance of photosynthesis can be linked to local electrical responses in leaves of pea. Front Physiol. 2017;8:763. doi:10.3389/fphys.2017.00763.
- Sukhov V, Surova L, Morozova E, Sherstneva O, Vodeneev V. Changes in Hþ-ATP synthase activity, proton electrochemical gradient, and pH in pea chloroplast can be connected with variation potential. Front Plant Sci. 2016;7:1092. doi:10.3389/fpls.2016.01092.
- Surova L, Sherstneva O, Vodeneev V, Sukhov V. Variation potential propagation decreases heat-related damage of pea photosystem I by 2 different pathways. Plant Signal Behav. 2016;11:e1145334. doi:10.1080/15592324.2016.1145334.
- Surova L, Sherstneva O, Vodeneev V, Katicheva L, Semina M, Sukhov V. Variation potential-induced photosynthetic and respiratory changes increase ATP content in pea leaves. J Plant Physiol. 2016;202:57–64. doi:10.1016/j.jplph.2016.05.024.
- Sukhov V, Surova L, Sherstneva O, Bushueva A, Vodeneev V. Variation potential induces decreased PSI damage and increased PSII damage under high external temperatures in pea. Funct Plant Biol. 2015;42:727–736. doi:10.1071/FP15052.
- Sukhova E, Mudrilov M, Vodeneev V, Sukhov V. Influence of the variation potential on photosynthetic flows of light energy and electrons in pea. Photosynth Res. 2018;136:215–228. doi:10.1007/s11120-017-0460-1.
- Szechynska-Hebda M, Lewandowska M, Karpinski S. Electrical signaling, photosynthesis and systemic acquired acclimation. Front Physiol. 2017;8:684. doi:10.3389/fphys.2017.00684.
- Choi WG, Miller G, Wallace I, Harper J, Mittler R, Gilroy S. Orchestrating rapid long-distance signaling in plants with Ca2+, ROS and electrical signals. Plant J. 2017;90:698–707. doi:10.1111/tpj.13492.