ABSTRACT
Identifying the maximum level of inherent defense against harmful insects in natural variation among wild lineages of crop plants may result in high yield tolerant varieties and reducing use of chemical insecticides. However, knowledge of natural cotton genotypes with high insect‐resistance is still indistinguishable at the biochemical or molecular level. In the present study, different cultivated Gossypium hirsutum varieties were evaluated for their inherent insect-tolerance against two major cottons chewing pests. The insect bio-assay identified two tolerant and one susceptible cotton varieties. The study demonstrates difference in phenolic acids, proanthocyanidin and tannin accumulation in tolerant and susceptible varieties. The post–infestation of chewing pests increases transcript level of the phenylpropanoid pathway genes were detected in tolerant varieties as compared to the susceptible varieties. Altogether, chewing pest-tolerance level in cotton varieties is the cumulative effect of enhanced phenylpropanoid pathway genes expression and secondary metabolite leading to defense responses to conventional host plant.
Introduction
Gossypium hirsutum species (Upland cotton) are largely domesticated as a source of textile fibers, contributing about 90% of the global cotton plantings. However, the cotton crop is susceptible to a wide range of insect pests. About 1300 insect species are reported which infest cotton all over the world.Citation1 Helicoverpa armigera (Hübner) (American bollworm) and Spodoptera litura (Fab.) (Cotton leafworm) are polyphagous lepidopteran pests with over 130 known host plant species including cotton.Citation2 To avoid the yield losses due to insect infestation, several various approaches involving conventional breeding and biotechnological methods are evolving for developing cotton crops to impart pest resistance. For example, the commercial exploitation of δ-endotoxin proteins of Bacillus thuringiensis (Bt), and large-scale implementation of insect resistant GM crops have significantly reduced the chemical insecticidal application, thereby altering the species distribution of insects in agriculture.Citation3–Citation6 Moreover, exploiting the host plant resistance toward the insects will lead to cheap, eco-friendly and one of the most effective methods to control pests and can be a better substitute for the insecticides. In order to cultivate those varieties which are insect-tolerant, the most essential step is to identify, characterize and categorize the effective sources of the inherent resistance in those varieties. Within plant species, there is distinct variation in inherent defense mechanisms that have been formed by alterations in genetic modifications and selection pressure.Citation7–Citation9 However, a few of these natural variations have been used in agricultural practices. Exploring highest level of inherent defense mechanisms in natural variation among wild lineages of cultivated crop plants, or crop plants accessions themselves could lead to more resistant or tolerant varieties.Citation10
Morpho-physical factors, as well as plant secondary compounds, are proximate and ultimate determinants of host-plant range in insect feeders.Citation11 Plant has plentiful intrinsic defensive strategies to perceive and endure with the pathogens’ aggression, including insects and viruses or by wide-range of chemical constituents (allelochemicals) either as part of their growth and development or to biotic stressresponse.Citation12,Citation13 Gossypol is considered one of the most potent insecticidal compound synthesized in cotton (Gossypium sps.) in glandular trichomes of leaves, stems and seeds of cotton and specifically produced in cotton cells glands due to different environmental stress.Citation14,Citation15 However, it is not only the component responsible for cotton host plant tolerance. Phenolics groups of secondary metabolite such as flavonoids, tannins and related phenolic precursors are well reported to have a significant role against fungal pathogens and insect herbivores.Citation16,Citation17 However, the information regarding the impact of chewing pest infestation on cotton phenolics is still not sufficient. Moreover, there has been very limited research related to the impact of phenolics, phenylpropanoid pathway and several other biochemical in different cultivated cotton genotypes against pests.Citation17–Citation20 Understanding the nature of the gene expression and regulation of the plant defensive traits will be helpful in establishing a better approach to design crop plants with enhanced defense properties against the insects.Citation21–Citation25
Therefore, the present study was performed using different G. hirsutum varieties to assess the conventional host plant tolerance in cotton crop against chewing pests. The study includes screening of cultivated cotton varieties based on insect feeding assay against prominent chewing pests of cotton, H. armigera and S. litura. We have also analyzed the gossypol content, accumulation of phenolics, proanthocyanidin and condensed tannins in leaf tissues of tolerant and susceptible cotton varieties. Quantitative gene expression analysis for phenylpropanoid pathway genes was also performed to assess their expression level in response to the chewing pest infestation.
Materials and methods
Plant material and insect culture
Twenty different varieties of cultivated cotton (Gossypium hirsutum) were used in the study, which were procured from UAS-D, Dharwad, JK Agri. Hyderabad and CICR, Nagpur under different programs. Seeds were soaked overnight in distilled water and incubated at 28°C for 1 day. The germinated seeds were planted in soil mix containing peat and vermiculite (1:1) in small plastic bags in a glasshouse and after four-leaf stage were shifted to properly manured soil in three random square plots. Nine biological replicates for each variety were maintained.
Insect reared in the laboratory was further used for all the experiments. Chewing insects, Helicoverpa armigera (cotton bollworm) and Spodoptera litura (cotton leafworm) egg masses and larvae were reared by feeding on artificial diet and fresh castor leaves respectively in the insect growth chamber (25 ± 2°C and relative humidity 60-80%) and the complete life cycle of insects was maintained in the institutional insectary, CSIR-National Botanical Research Institute, Lucknow.
Insect bioassay
The fresh cotton leaves were plucked just before initiating the experiment and washed thoroughly, blotted dry and placed in sample containers (7 cm × 5 cm). Experiments were conducted at 30, 45 & 65 days old different varieties of the cotton plant on the leaf position 2nd, 5th& 7th from the top. Ten neonate larvae of S. litura and H. armigera were carefully released over the detached leaves. The larval population and survivorship were monitored for the next 7 days. Observations for mortality percentage [3 detached leaves of 3 different plants of each variety) x 3 times repeated = 27] were done on alternate days and surviving larvae weights were measured on the 7th day. All experiments were conducted at 25 ± 2° C, 60 ± 10% RH) and three replicates were maintained in each case.
In-planta insect bioassay
In-planta insect bioassay was conducted using the three identified varieties. For this study, six weeks old cotton plants (Bc-68-2, Gcot-16 and Coker-312) were grown from seeds and maintained in a glass-house condition (16:8 h light:dark photoperiod, light intensity 112.3 ± 18.5 μmol m−2 s−1, 23°C, 70 ± 5% relative humidity). The three leaves were used for performing these experiments with three identified varieties of cotton plants in the following conditions: (a) controls without chewing pest infestation; (b) infestation with H. armigera (c) infestation with S. litura. Well-watered healthy potted plants free from incidental pests and diseases having approximately six leaves were used for performing these experiments. Thirty neonate larvae were placed on each cotton varieties with an average of 10 larvae per leaf and covered in insect-proof gauze sleeves/pinched polybag to avoid their escape. Larvae were allowed to feed the plant continuously for 96 h and larvae weight and mortality observed at 0 hr, 48 h, 72 h and 96 h.
Morphological and histochemical studies
Plant leaves were sampled to compare basic morphology at different growth stages. Scanning electron microscopy (SEM) was performed to explore the stomatal count and trichome density in cotton leaf and images were taken under standard conditions (10 kV, 20 mm working distance, and a standard spot size). The computer measuring program Image-J software (Image processing and analysis in Java) was used to measure trichome and stomatal densities and length. Conventional preparative processes involved chemical fixation of fresh leaf fragments in the vapor of 4% solution of osmium tetroxide, followed by dehydration in a graded series of acetone and critical point drying. Specimens were mounted on aluminum stubs with conductive adhesive tape and sputter coated with gold before investigation in a JSM-6490LV SEM. For histochemical analysis, the fresh young and mature leaves were stained using vanillin-HCl for phenolics and 4-dimethylaminocinnamaldehyde (DMACA) for condensed tannins.Citation26
Bio-chemical analysis of gossypol, carbohydrate and protein profiling
All biochemical assays were executed using Lambda 35 UV/Vis spectrometer. Extraction and estimation of gossypol were done according to Verma et al. (2009).Citation27 The carbohydrate content was estimated according to Gerhardt et al. (1994).Citation28 The protein content was estimated according to Lowry (1951).Citation29 Phenolic acid extraction from leaf tissues and its estimation was performed following the proposed methods by Lege et al. (1995).Citation30
Total condensed tannin estimation
Total condensed tannin (proanthocyanidins) was measured according to porter et al. (1986).Citation31 500 µL of the phenolic extract was taken in a new test tube. To the extract, 3 mL butanol-HCl (butanol: HCl, 95:5 v/v) and 100 µL of ferric reagent (ferric ammonium sulfate in 2 N HCl) were added. The sample was properly vortexed followed by boiling for 1 h. Blank formed for each sample without heating the reagent. After cooling to room temperature, OD was measured at ʎ = 550 nm. Condensed tannin as leucocyanidin equivalent was calculated as % CT = (A550 x 78.26 x dilution factor)/(% dry matter) tannic acid (µg).
Measurement of physiological parameters
The photosynthesis rate (PR), transpiration rate (TR), stomatal conductance (SC), and water use efficiency (WUE) were done according to the method described in the Ranjan et al. 2012.Citation32 The leaf gas exchange method was used for measuring net PR, TR and SC with blue/red LED light sources using a portable photosynthesis system (LI-6400, Li-Cor, Lincoln, NE, USA). Measurements were conducted at 400 μmol CO2 mol−1 air, constant vapor pressure deficit (VPD = 2.5 kPa ± 0.2) and leaf temperature (33 ± 2°C) after steady-state photosynthetic rates accomplishment. The ratio of PR to TR was obtained for the intrinsic photosynthetic WUE. The dark respiration (DR) was measured in similar microclimatic environments after 30 min of the leaf dark adaptation. Measurements of relative water content (RWC) and water potential were completed at predawn on single, entirely extended leaves promptly after cutting out. The leaf water potential (Ψ) was calculated with a plant water status console (Soilmoisture, Santa Barbara, CA), while leaf RWC was obtained as 100 × (fresh weight – dry weight)/(turgid weight – dry weight).
Phenyl ammonia-lyase enzyme assay
PAL (EC 4.3.1.24) enzyme activity was measured according to Cahill and McComb (1992). Briefly, leaf sample (500 mg) was homogenized in Tris-HCl buffer (100 mM, pH 8.9) containing β-mercaptoethanol (5 mM) and PVP (0.050 gm) in ice at 4° C. The assay mixture was centrifuged at 4°C for 20 min at 12,000 x g. The supernatant was collected and used for analyzing enzyme activity. Reaction mixture was prepared by mixing enzyme extract (500 µL) with borate buffer (20 mM, pH 8.9) containing 500 µL of L-phenylalanine substrate (10 mM). The reaction was stopped after 24 h by adding 1.5 ml of HCl (2 N). PAL activity was determined by the absorbance change due to the development of trans-cinnamic acid by measuring absorbance at 290 nm. By calculating the relative changes in PAL activity with respect to the initial point of the experiment (zero hour), fold increase or decrease in enzyme activity was calculated separately in each case. Statistical analysis was conducted by using variance analysis (SPSS 6.0). Differences at significant level of p < 0.05 were estimated using LSD.
RNA isolation and real-time PCR
The quantitative RT-PCR was performed according to the method described by Pandey et al 2016.Citation33 Total RNA was isolated using Sigma total RNA isolation kit following manufacturer’s protocol and 2 µg of DNase free total RNA extracted from the control and infested leaf samples was used to synthesize first strand cDNA. The synthesized cDNA was used to perform quantitative RT-PCR (qRT-PCR) for all set of selected genes using SYBR Green. The cotton ubiquitin gene was used as an internal control. The relative expression level of target genes was calculated by ΔΔCt method. The list of selected genes and oligonucleotide primers used for real-time PCR are specified in Supplementary Table S6.
Results
Differential insect tolerance in cultivated cotton varieties against chewing pests
The insect bioassay against H. armigera and S. litura were performed on the leaves for initial screening of the 20 G. hirsutum varieties. The detached leaf insect bioassay revealed that larval weight was lowest in two varieties Bc-68-2 and Gcot-16 and one variety Coker-312 showed the highest larval weight among selected G. hirsutum varieties (; Tables S1-S2). In comparison to the Coker-312, Bc-68-2 and Gcot-16 varieties displayed high mortality during different growth stages (). In planta insect bioassay, Coker-312, Bc-68-2 and Gcot-16 varieties displayed similar effect on H. armigera and S. litura (Supplementary Fig S1). Therefore, these results suggested that Bc-68-2 and Gcot-16 showed comparatively more tolerance for H. armigera and S. litura, while Coker-312 displayed equal susceptibility to both the insects among selected G. hirsutum varieties.
Figure 1. Insect tolerance in cultivated cotton varieties against chewing pests. Upper panel showed detached leaf insect bioassay against post 7 days of feeding chewing insects S. litura (a) and H. armigera (b) on Bc-68-2, Gcot-16 and Coker-312 varieties at 30 days old leaf. Lower panel showed mortality percentage (Line graph) and weight reduction (Bar graph) of S. litura and H. armigera (s) feeding on Bc-68-2, Gcot-16 and Coker-312 varieties at different growth stages of plants (30, 45 and 65 days). Mean ± SE of the three repeated experiments on each of 30, 45 and 65 days old plants. The significant differences for larval mortality percentage with respect to Bc-68-2, P < 0.05, *for 30 days, **for 45 days, # for 65 days. The significant differences for weight reduction with respect to Bc-68-2, * = P < 0.05.
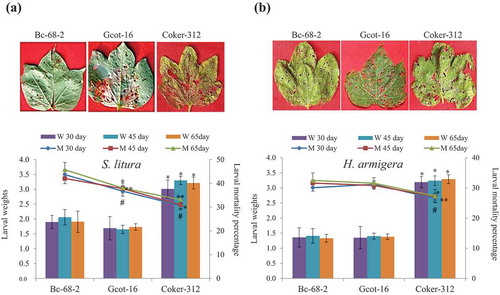
Distinct trichome and stomatal density in cotton varieties potentially correlates with insect tolerance
Morphological assessment of tolerant (Bc-68-2 and Gcot-16) and susceptible (Coker-312) varieties displayed non-significant variation in terms of plant height, leaf count, boll count, seed/boll and canopy cover (Table S3). In both tolerant and susceptible varieties, the photosynthesis rate ranged between 15.80 and 18.16 µmol m−2 s−1, stomatal conductance ranged between 0.29 and 0.38 mol m−2 s−1, transpiration rate ranged between 6.6 and 7.65 mmol m−2 s−1 and water use efficiency ranged between 2.18 and 2.41 (Table S4). The SEM analysis suggested that there was variation in trichome density among the tolerant and susceptible varieties (Fig. S2). In comparison to Coker-312 (297.99 µm) and Bc-68-2 (247.05 µm), Gcot-16 possessed significantly longer trichome (457.74 µm) (Table S5). Additionally, stomata density in Bc-68-2 is comparatively higher than in Gcot-16 and Coker-312 (Table S5).
Primary metabolite, Gossypol and phenolics level in the tolerant and susceptible varieties
Nutritive value of Bc-68-2, Gcot-16 and Coker-312 varieties in terms of the primary metabolite such as carbohydrate and protein content displayed no significant difference even at different plant ages (). The estimations of the carbohydrate and proteins revealed altered levels in pest-infected cotton plant with H. armigera and S. litura as compared to the non-infested plant. Post in-planta pest infestation study revealed a decline in the carbohydrate level while accumulation of proteins increased in both susceptible and tolerant varieties with the duration of infestation (,d).
Figure 2. The changes in carbohydrate and protein metabolism in the cotton varieties due chewing pest infestation. Basal concentration levels of total carbohydrates (a) and total proteins (b) in cotton varieties at different growth stages (30, 45 and 65 days). Values are averages of nine independent replicates ± SD. The change in total carbohydrate (c) total protein (d) level at indicated time points during biting and chewing pest infestation. Significant differences between control and treatment are indicated by * = P < 0.05.
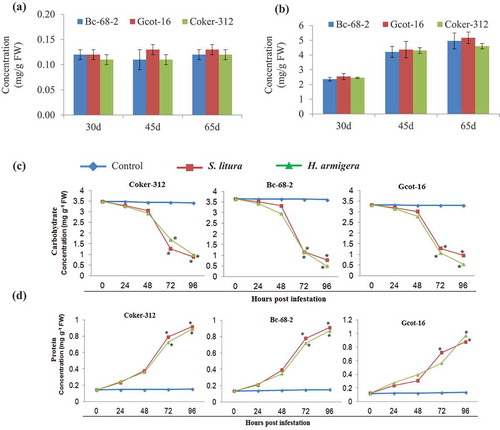
Gossypol content showed almost similar production in both tolerant Bc-68-2 and Gcot-16 and susceptible Coker-312 varieties (). Equivalent gossypol content in the selected varieties switched the investigation toward another class of defensive metabolites. Interestingly, preliminary staining of phenolics and proanthocyanidins in the young and mature leaf tissue displayed a significant variation in the basal accumulation of these compounds in the selected varieties (,c). Mature leaves displayed a higher accumulation of phenolics in the tolerant varieties Bc-68-2 and Gcot-16 as compared to the susceptible Coker-312 (,c). Biochemical analysis of different growth stages of cotton varieties (30 d, 45 d and 65 d) confirmed the above observation of increment in phenolic and condensed tannin content with the age of the plant (). Moreover, quantitative increment in phenolic and condensed tannin content since beginning with maximum level attained at 96 hours post infestation (hpi). Both chewing pests induced the accumulation of these biochemicals in both tolerant as well as the susceptible variety ().
Table 1. Phenolic and condensed tannin concentration in leaf tissue of tolerant and susceptible varieties at different growth stages.
Figure 3. The effect of gossypol, phenolics and condensed tannin in different cotton varieties. (a) Basal concentration of gossypol level in the leaf tissues of cotton varieties at different growth stages (30, 45 and 65 days). Values are averages of nine independent replicates ± SD. (b) Histochemical staining for phenolics after 15 min of staining (Vanillin-HCl) in young & mature leaf of Coker-312, Bc-68-2 and Gcot-16 cotton. (c) Histochemical staining for proanthocyanidin accumulation in young & mature leaf after 30 min of staining (DMACA) of Coker-312, Bc-68-2 and Gcot-16 cotton. (d-e) The effect of chewing pest infestation on phenolics accumulation in leaf tissue of Coker-312, Bc-68-2 and Gcot-16 cotton at 0 h, 24 h, 48 h, 72 h and 96 h post infestation. Significant differences between control and treatment are indicated by * = P < 0.05. (f-g). The effect of chewing pest infestation on condensed tannin in leaf tissue of Coker-312, Bc-68-2 and Gcot-16 cotton at 0 h, 24 h, 48 h, 72 h and 96 h post infestation. Significant differences between control and treatment are indicated by * = P < 0.05.
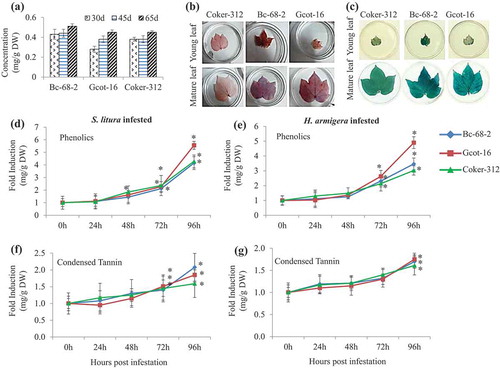
Phenylpropanoid pathway gene expression levels in tolerant and susceptible varieties
The enhanced activity of key enzymes of the phenylpropanoid pathway plays an important function in defense.Citation17,Citation19 We further analyzed the effect of gene expression level of these enzymes (PAL, CHS, CHI, F3H, DFR, ANS and ANR) in tolerant Bc-68-2 and Gcot-16 and susceptible Coker-312 (). Quantitative PCR analysis suggested that gene expression of phenylpropanoid pathway genes was induced in both tolerant and susceptible varieties during infestation by both chewing pests (). Interestingly, the transcript accumulation of PAL and ANS was higher in tolerant varieties as compared to the susceptible one while CHS, CHI, ANR, DFR and F3H transcripts accumulation pattern were almost similar in both tolerant as well as the susceptible variety. A noticeable change in expression of PAL gene began to appear on the infested leaves of Bc-68-2 and Gcot-16 at 24 hpi, whereas in Coker-312, PAL gene showed an increase after 48 hpi, reached the highest values at 96 hpi infested by H. armigera and S. litura. Another gene ANS showed significant accumulation of transcripts in tolerant varieties B-68-2 and Gcot-16 as compared to the susceptible Coker-312 after 48 hpi infested with both H. armigera and S. litura (). These results suggested that susceptible and tolerant varieties showed enhanced phenylpropanoid pathway gene expression during insect attack, however tolerant varieties Bc-68-2 and Gcot-16 showed a high expression pattern of PAL and ANS in comparison of the susceptible variety Coker-312.
Figure 4. Induction of the phenylpropanoid pathway genes in different cotton varieties. (a) General schematic of the phenylpropanoid and flavonoid biosynthetic pathways. (b-c) The effect of chewing pest infestation on the phenylpropanoid pathway genes transcript accumulation in leaf tissue (n = 3) of tolerant and susceptible cotton varieties at 0 h, 24 h, 48 h, 72 h and 96 h post infestation. The genes used in qRT-PCR are: Phenylalanine ammonia lyase (PAL), Chalcone synthase (CHS), Chalcone isomerase (CHI), Flavonoid-3ʹhydroxylase (F3H) Dihydroflavonol reductase (DFR), Anthocyanin synthase (ANS) and Anthocyanin reductase (ANR). Significant differences between control and treatment are indicated by * = P < 0.05.
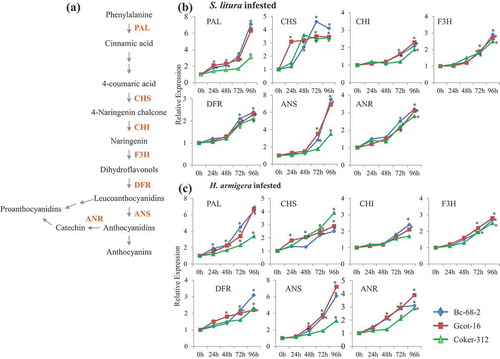
Since, the PAL transcript level showed the highest fold increase postinsect infestation in tolerant as well as susceptible varieties, an increase in PAL activity was examined in the leaves at a different time interval of chewing pest infestation (,b). The activity of PAL in chewing (H. armigera and S. litura) pest-infested plants increased from the start, up to 96 hpi experiments. Similar to PAL gene expression, the enzyme activity was enhanced in S. litura and H. armigera infested Bc-68-2 and Gcot-16 after 48 hpi and attained its highest value at 96 hpi, respectively (,b). While in the case of susceptible Coker-312, the enhancement in the level of PAL activity was lower as compared to the tolerant varieties (). Significant differences in PAL activity were observed in Bc-68-2 and Gcot-16 at 72 hpi and 96 hpi as compared to Coker-312. In the controls, the PAL enzyme activity remained at a low level and slightly changed throughout the experiment. The PAL enzyme assay confirmed that the phenylpropanoid pathway plays a significant role to induce more defense activity in tolerant B-68-2 and Gcot-16 as compared to susceptible variety Coker-312.
Figure 5. The PAL enzyme activity in different cotton varieties. The effect of chewing pest infestation on PAL activity in the leaf tissue (n = 3) of Coker-312, Bc-68-2 and Gcot-16 at 0 h, 24 h, 48 h, 72 h and 96 h post infestation. Significant difference in control and infested plants is represented by * = P ≤ 0.05.
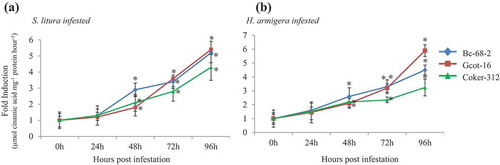
Discussion
The tolerant/resistant cultivars usage is an essential part of an integrated approach to insect pest management.Citation34,Citation35 The insect-resistant cotton variety identification and deciphering its resistance mechanisms will be a significant achievement in the area of cotton cultivation. Through comprehensive screening, we identified two tolerant and one susceptible G. hirsutum variety on the basis of the lowest weight gain and lowest survival rate in the neonate larvae of H. armigera and S. litura. Similarly, previous studies suggest about genetic variations in crop genotypes of same species, provide insect resistance, for example, rice genotypes against brown planthopper (Nilaparvata lugens) and chickpea against fungal pathogen A. rabiei.Citation36,Citation37
Plant morphology and nutrient content play a significant role in providing protection from insect pests.Citation38 Insignificant variation in morphology, as well as nutritive amount (such as carbohydrate or protein content), was observed in both tolerant (Bc-68-2 and Gcot-16) and susceptible (Coker-312) at different growth stages (). From earlier reports, genotype-independent post-infestation decline in carbohydrate content likely helps the plant to maintain a nutritional deficit situation to starve the attacking pests.Citation39 The increase in protein levels might be due to the production of defense-related proteins or compounds at higher concentrations, as a component of host resistance mechanism as also previously reported in Eucalyptus globulus, Manihot utilissima, and Ricinus communis infected by Retithrips syriacus.Citation40
Gossypol and phenolics contribute a major role in defense system of the cotton plant.Citation41,Citation42 However, no significant differences in gossypol level were observed in leaf tissues or during different growth stages of tolerant and susceptible varieties. DAMCA and vanillin-HCl staining results clearly emphasized the possibility of higher flavonoids content in the leaves of tolerant varieties Bc-68-2 and Gcot-16 (). Previous studies on Gossypium spp. also suggested that the flavanol concentration varies considerably with different tissues and the age of the plant.Citation41,Citation43 It is also observed that the old leaves of cotton are more prone to insect attack due to low flavonol or phenolics content but, insect attack leads to marked increment in their concentration.Citation44,Citation45 In our study, there was a decline in larvae weight gain and survival when fed from young to mature leaves, which suggest it may be due to the enhanced flavonol or phenolics content in the leaves by pest infestation.
Several accumulating studies suggested that higher biosynthesis of phenolic acids after insect infestation, for instance, Lamberto cv. seedlings after aphid feeding and in rice plants when attacked by rice leaf folder Cnaphalocrocis medinalis.Citation46,Citation47 It is also reported that condensed tannin content in mature leaves is more than two-fold high as those in the developing leaves.Citation48 In addition, the basal abundance of condensed tannin was also evident in the leaf tissue of tolerant cotton varieties reported here. However, the previous findings also suggests that the total phenolic concentrations declined in contrast to increased condensed tannin concentration from pinhead-square to first-bloom growth stages in some different varieties of G. hirsutum, which give tolerant to spider mites due to high levels of condensed tannins and phenolic acids.Citation30 This contrasting observation indicates there could be some genotype-dependent effect on flavonoids/phenolics accumulation in cotton plants.
The gene expression and post-transcriptional regulation play an important function in the understanding of resistance mechanisms and potent genes identification involved in plant defense mechanism.Citation49–Citation52 Due to the infestation, genomic reprogramming occurs which ultimately controls the proteins synthesis or production of secondary compounds. The phenylpropanoid pathway occupies a central poition in the plant defense pathway. The previous evidence of induced accumulation of phenylpropanoids has been reported in response to insect feeding in wheat tissues as a response to Sitodiplosis mosellana and corn exposed to Sesamia nonagrioides.Citation53,Citation54 In the present study, we have documented a noticeable induction of genes involved in phenylpropanoid pathway (). This has a similarity with previous findings, which suggests that the synthesis of stress metabolites increases the flow of L-phenylalanine toward phenolic synthesis.Citation55 The expression profile of phenylpropanoid pathway was variable in the tolerant and susceptible varieties. Although, the expression of all the seven studied genes showed post-infestation transcript accumulation but, the transcript level of two genes, namely PAL and ANS was considerably higher as compared to the other genes in tolerant Bc-68-2 and Gcot-16 and low accumulation in the susceptible Coker-312 (). Our results fall in parallel with the previous study indicating the gene PAL played a considerable role giving resistance to the resistant Kasalath rice variety in response to small brown plant hopper infestation.Citation56 This is preferred by the previous reports emphasizing that the de novo synthesis and augmented PAL activity are an initial plant defensive mechanism to pest devastation and results in phenolic compounds accumulation in plants that are sequestered in cell vacuole.Citation57,Citation58 But, our results are in contrast with the earlier studies reporting decreased PAL activity in tea plants infested with Helopeltis theivora and wild cotton variety of G. hirsutum infested with S. litura in a leaf-disc assay.Citation59,Citation60 The transgenic plant overexpressing Arabidopsis transcription factor PAP1 enhances the production of the flavonoid and thereby provides resistance from armyworms Spodoptera frugiperda.Citation61 Notably, transcriptome study analysis in the G. hirsutum suggested that transcripts accumulation of phenylpropanoid biosynthesis, flavonoids, and aromatic compounds were observed in the later phase of sap-sucking insect infestation, indicating that plant induces secondary metabolite biosynthesis pathway-associated gene regulation to provide defense from insects.Citation62 These results suggest that the cotton plants infested with chewing pests show up-regulated expression of genes involved in proanthocyanidins biosynthesis, which further suggest that the flavonoid-biosynthesis related genes were considerably activated and displayed coordinate expression patterns during pest invasion.
In conclusion, the study demonstrated that tolerance of cotton variety particularly Gcot-16 for chewing pests (H. armigera and S. litura) possibly due to insecticidal secondary metabolites including phenolics and flavanols. The findings have confirmed that post infestation, differential expression of phenylpropanoids pathway genes is also playing a major role in plant defense and are responsible for the metabolic flux of an individual compound. On the contrary, the susceptibility of Coker-312 is related to the weak and little delayed response with respect to the generation of the disproportionately low level of few metabolites followed by low expression of key regulatory genes of the phenylpropanoid pathway.
Supplemental Material
Download PDF (320.7 KB)Acknowledgments
Authors are grateful to Dr. V.N. Waghmare, CICR, Nagpur for providing seeds of some cotton varieties and GD is thankful to the Department of Science and Technology for DST-INSPIRE - Senior Research Fellowship. The research was supported by SERB, New Delhi, Government of India, project No.GAP-3401.
Institute Manuscript Number is ‘CSIR-NBRI_MS/2020/02/08ʹ
Supplementary material
Supplemental data for this article can be accessed on the publisher’s website.
Additional information
Funding
References
- Matthews GA, Tunstall JP. Insect pests of cotton. Wallingford: CAB International, University Press; 1994.
- Matthews M. Heliothine moths of Australia. A guide to pest bollworms and related noctuid groups. Melbourne: CSIRO Publishing; 1999.
- Chidawanyika F, Mudavanhu P, Nyamukondiwa C. Biologically based methods for pest management in agriculture under changing climates: challenges and future directions. Insects. 2012;3:1–10. doi:10.3390/insects3041171.
- Alphey N, Bonsall MB. Genetics-based methods for agricultural insect pest management. Agric Entomol. 2018;20:131–140. doi:10.1111/afe.12241.
- Lombardo L, Coppola G, Zelasco S. New technologies for insect-resistant and herbicide-tolerant plants. Trends Biotechnol. 2016;34:49–57. doi:10.1016/j.tibtech.2015.10.006.
- Gatehouse JA. Biotechnological prospects for engineering insect-resistant plants. Plant Physiol. 2008;146:881–887. doi:10.1104/pp.107.111096.
- Kant MR, Jonckheere W, Knegt B, Lemos F, Liu J, Schimmel BCJ, Villarroel CA, Ataide LMS, Dermauw W, Glas JJ, et al. Mechanisms and ecological consequences of plant defence induction and suppression in herbivore communities. Ann Bot. 2015;115(7):1015–1051. doi:10.1093/aob/mcv054.
- Chaudhary B. Plant domestication and resistance to herbivory. Int J Plant Genomics. 2013;2013:572784. doi:10.1155/2013/572784.
- Thompson JN. Coevolution: the geographic mosaic of coevolutionary arms races. Curr Biol. 2005;15:R992–4. doi:10.1016/j.cub.2005.11.046.
- Broekgaarden C, Snoeren TA, Dicke M, Vosman B. Exploiting natural variation to identify insect-resistance genes. Plant Biotechnol J. 2011;9:819–825. doi:10.1111/j.1467-7652.2011.00635.x.
- Tosh CR, Powell G, Hardie J. Decision making by generalist and specialist aphids with the same genotype. J Insect Physiol. 2003;49:659–669. doi:10.1016/S0022-1910(03)00066-0.
- Vagiri M, Johansson E, Rumpunen K. Phenolic compounds in black currant leaves – an interaction between the plant and foliar diseases? J Plant Interact. 2017;12:193–199. doi:10.1080/17429145.2017.1316524.
- Lattanzio V, Lattanzio VM, Cardinali A. Role of phenolics in the resistance mechanisms of plants against fungal pathogens and insects. Phytochem. 2006;23–67.
- Bell AA, Stipanovic RD, Howell CR, Fryxell PA. Antimicrobial terpenoids of gossypium: hemigossypol, 6-methoxyhemigossypol and 6-deoxyhemigossypol. Phytochemistry. 1975;14:225–231. doi:10.1016/0031-9422(75)85044-8.
- Dowd MK, Pelitire SM. Isolation of 6-methoxy gossypol and 6,6ʹ-dimethoxy gossypol from Gossypium barbadense Sea Island cotton. J Agric Food Chem. 2006;54:3265–3270. doi:10.1021/jf060027n.
- Treutter D. Significance of flavonoids in plant resistance: a review. Environ Chem Lett. 2006;4:147. doi:10.1007/s10311-006-0068-8.
- Vogt T. Phenylpropanoid biosynthesis. Mol Plant. 2010;3:2–20. doi:10.1093/mp/ssp106.
- Yuan D, Jin L, Xu L, Tu L, Liu L, Zhu L, Long L, Zhang X. Lignin metabolism has a central role in the resistance of cotton to the wilt fungus Verticillium dahliae as revealed by RNA-Seq-dependent transcriptional analysis and histochemistry. J Exp Bot. 2011;62:5607–5621. doi:10.1093/jxb/err245.
- Naoumkina MA, Zhao Q, Gallego-Giraldo L, Dai X, Zhao PX, Dixon RA. Genome-wide analysis of phenylpropanoid defence pathways. Mol Plant Pathol. 2010;11:829–846. doi:10.1111/j.1364-3703.2010.00648.x.
- Dixit G, Praveen A, Tripathi T, Yadav VK, Verma PC. Herbivore-responsive cotton phenolics and their impact on insect performance and biochemistry. J Asia Pac Entomol. 2017;20:341–351. doi:10.1016/j.aspen.2017.02.002.
- Srivastava R, Rai KM, Srivastava M, Kumar V, Pandey B, Singh SP, Bag SK, Singh BD, Tuli R, Sawant SV. Distinct Role of Core Promoter Architecture in Regulation of Light-Mediated Responses in Plant Genes. Mol Plant. 2014;7:626–641. doi:10.1093/mp/sst146.
- Srivastava R, Srivastava R, Singh UM. Understanding the patterns of gene expression during climate change. Climate Change Effect on Crop Productivity. Boca Raton, Florida: CRC Press, Taylor & Francis Group; 2014. p. 279–328. Print ISBN: 978-1-4822-2920-2 eBook ISBN: 978-1-4822-2921-9. doi:10.1201/b17684-14.
- Huot B, Yao J, Montgomery BL, He SY. Growth-defense tradeoffs in plants: a balancing act to optimize fitness. Mol Plant. 2014;7:1267–1287. doi:10.1093/mp/ssu049.
- Fu R, Martin C, Zhang Y. Next-generation plant metabolic engineering, inspired by an ancient Chinese irrigation system. Mol Plant. 2018;11:47–57. doi:10.1016/j.molp.2017.09.002.
- Pandey B, Prakash P, Verma PC, Srivastava R. Regulated gene expression by synthetic modulation of the promoter architecture in plants. Current developments in biotechnology and bioengineering: synthetic biology. Cell Eng Bioprocess Technol. 2019;235–255.
- Abeynayake SW, Panter S, Mouradov A, Spangenberg G. A high-resolution method for the localization of proanthocyanidins in plant tissues. Plant Methods. 2011;7:13. doi:10.1186/1746-4811-7-13.
- Verma PC, Trivedi I, Singh H, Shukla AK, Kumar M, Upadhyay SK, Pandey P, Hans A, Singh P. Efficient production of gossypol from hairy root cultures of cotton (Gossypium hirsutum L.). Curr Pharm Biotechnol. 2009;10:691–700. doi:10.2174/138920109789542048.
- Gerhardt P, Murray R, Wood WA, Krieg NR. Methods for general and molecular bacteriology. Washington DC (United States): American Society for Microbiology; 1994.
- Lowry OH, Rosebrough NJ, Farr AL, Randall RJ. Protein measurement with the Folin phenol reagent. J Biol Chem. 1951;193:265–275.
- Lege KE, Cothren JT, Smith CW. Phenolic acid and condensed tannin concentrations of six cotton genotypes. Environ Exp Bot. 1995;35:241–249. doi:10.1016/0098-8472(94)00051-6.
- Porter LJ, Hrstich LN, Chan BG. The conversion of procyanidins and prodelphinidins to cyanidin and delphinidin. Phytochemistry. 1985;25:223–230. doi:10.1016/S0031-9422(00)94533-3.
- Ranjan A, Nigam D, Asif MH, Singh R, Ranjan S, Mantri S, Pandey N, Trivedi I, Rai K, Jena SN, et al. Genome wide expression profiling of two accession of G. herbaceum L. in response to drought. BMC Genomics. 2012;13:94. doi:10.1186/1471-2164-13-94.
- Pandey V, Srivastava R, Akhtar N, Mishra J, Mishra P, Verma PC. Expression of Withania somnifera steroidal glucosyltransferase gene enhances withanolide content in hairy roots. Plant Mol Bio Rep. 2016;34:681–689. doi:10.1007/s11105-015-0955-x.
- Lewis WJ, van Lenteren JC, Phatak SC, Tumlinson JH 3rd. A total system approach to sustainable pest management. Proc Natl Acad Sci U S A. 1997;94:12243–12248. doi:10.1073/pnas.94.23.12243.
- Trapero C, Wilson IW, Stiller WN, Wilson LJ. Enhancing integrated pest management in GM cotton systems using host plant resistance. Front Plant Sci. 2016;7:500. doi:10.3389/fpls.2016.00500.
- Alagar M, Suresh S, Samiyappan R, Saravanakumar D. Reaction of resistant and susceptible rice genotypes against brown planthopper (Nilaparvata lugens). Phytoparasitica. 2007;35:346. doi:10.1007/BF02980697.
- Weigand F, Koster J, Weltzien HC, Barz W. Accumulation of phytoalexins and isoflavone glucosides in a resistant and a susceptible cultivar of Cicer arietinum during infection with Ascochyta rabiei. J Phytopathol. 1986;115:214–221. doi:10.1111/j.1439-0434.1986.tb00879.x.
- Awmack CS, Leather SR. Host plant quality and fecundity in herbivorous insects. Annu Rev Entomol. 2002;47:817–844. doi:10.1146/annurev.ento.47.091201.145300.
- Lawrence PK, Koundal KR. Plant protease inhibitors in control of phytophagous insects. Electron J Biotechnol. 2002;5:5–6. doi:10.2225/vol5-issue1-fulltext-3.
- Ananthakrishnan TN, Gopichandran R, Gurusubramanian G. Influence of chemical profiles of host plants on the infestation diversity ofRetithrips syriacus. J Biosci. 1992;17:483–489. doi:10.1007/BF02720103.
- Nix A, Paull C, Colgrave M. Flavonoid profile of the cotton plant, gossypium hirsutum: a review. Plants (Basel). 2017;6:E43. doi:10.3390/plants6040043.
- Jenkins JN, Hedin PA, Parrott WL. Relationships of glands, cotton square terpenoid aldehydes, and other allelochemicals to larval growth of heliothis virescens (Lepidoptera: noctuidae). J Econ Entomol. 1992;85:359–364. doi:10.1093/jee/85.2.359.
- Bell AA, Stipanovic RD, O’Brien DH, Fryxell PA. Sesquiterpenoid aldehyde quinones and derivatives in pigment glands of Gossypium. Phytochemistry. 1978;17:1297–1305. doi:10.1016/S0031-9422(00)94578-3.
- Bell AA. Physiology of secondary products. In: JR MAUNEY, JM STEWART, editors. Cotton Physiology- a treatise: section V, phenolic caids. The cotton Foundation. Netherlands: Springer. 1986; 187–205.
- Mace ME, Bell AA, Stipanovic RD. Histochemistry and identification of flavanols in Verticillium wilt-resistant and -susceptible cottons. Physiol Plant Pathol. 1978;13:143–149. doi:10.1016/0048-4059(78)90027-9.
- Chrzanowski G, Leszczyński B. Induced accumulation of phenolic acids in winter triticale (Triticosecale Wittm.) under insects feeding. Herba Polonica. 2008;58:33–40.
- Punithavalli M, Muthukrishnan NM, Rajkuma MB. Defensive responses of rice genotypes for resistance against rice leaffolder Cnaphalocrocis medinalis. Rice Sci. 2013;20:363–370. doi:10.1016/S1672-6308(13)60149-3.
- Donaldson JR, Stevens MT, Barnhill HR, Lindroth RL. Age-related shifts in leaf chemistry of clonal aspen (Populus tremuloides). J Chem Ecol. 2006;32:1415–1429. doi:10.1007/s10886-006-9059-2.
- Andersen EJ, Ali S, Byamukama E, Yen Y, Nepal MP. Disease resistance mechanisms in plants. Genes (Basel). 2018;9(7):339.
- Thomma BP, Penninckx IA, Broekaert WF, Cammue BP. The complexity of disease signaling in Arabidopsis. Curr Opin Immunol. 2001;13:63–68. doi:10.1016/S0952-7915(00)00183-7.
- Kliebenstein DJ. Plant defense compounds: systems approaches to metabolic analysis. Annu Rev Phytopathol. 2012;50:155–173. doi:10.1146/annurev-phyto-081211-172950.
- Li B, Gaudinier A, Tang M, Taylor-Teeples M, Nham NT, Ghaffari C, Benson DS, Steinmann M, Gray JA, Brady SM, et al. Promoter-based integration in plant defense regulation. Plant Physiol. 2014;166:1803–1820. doi:10.1104/pp.114.248716.
- Ding H, Lamb RJ, Ames N. Inducible production of phenolic acids in wheat and antibiotic resistance to sitodiplosis mosellana. J Chem Ecol. 2000;26:969–985. doi:10.1023/A:1005412309735.
- Santiago R, Malvar RA, Baamonde MD, Revilla P, Souto XC. Free phenols in maize pith and their relationship with resistance to Sesamia nonagrioides (Lepidoptera: noctuidae) attack. J Econ Entomol. 2005;98:1349–1356. doi:10.1603/0022-0493-98.4.1349.
- Cramer CL, Edwards K, Dron M, Liang X, Dildine SL, Bolwell GP, Dixon RA, Lamb CJ, Schuch W. Phenylalanine ammonia-lyase gene organization and structure. Plant Mol Biol. 1989;12:367–383. doi:10.1007/BF00017577.
- Duan C, Yu J, Bai J, Zhu Z, Wang X. Induced defense responses in rice plants against small brown planthopper infestation. Crop J. 2014;2:55–62. doi:10.1016/j.cj.2013.12.001.
- Campos-Vargas R, Saltveit ME. Involvement of putative chemical wound signals in the induction of phenolic metabolism in wounded lettuce. Physiol Plant. 2002;114:73–84. doi:10.1034/j.1399-3054.2002.1140111.x.
- Zhao LY, Chen JL, Cheng DF, Sun JR, Liu Y, Tian Z. Biochemical and molecular characterizations of Sitobion avenae-induced wheat defense responses. Crop Prot. 2009;28:435–442. doi:10.1016/j.cropro.2009.01.005.
- Chakraborty U, Chakraborty N. Impact of environmental factors on infestation of tea leaves byHelopeltis theivora, and associated changes in flavonoid flavor components and enzyme activities. Phytoparasitica. 2005;33:88–96. doi:10.1007/BF02980930.
- Usha Rani P, Pratyusha S. Defensive role of Gossypium hirsutum L. anti-oxidative enzymes and phenolic acids in response to Spodoptera litura F. feeding. J Asia Pac Entomol. 2013;16:131–136. doi:10.1016/j.aspen.2013.01.001.
- Johnson ET, Dowd PF. Differentially enhanced insect resistance, at a cost, in Arabidopsis thaliana constitutively expressing a transcription factor of defensive metabolites. J Agric Food Chem. 2004;52:5135–5138. doi:10.1021/jf0308049.
- Dubey NK, Goel R, Ranjan A, Idris A, Singh SK, Bag SK, Chandrashekar K, Pandey KD, Singh PK, Sawant SV, et al. Comparative transcriptome analysis of Gossypium hirsutum L. in response to sap sucking insects: aphid and whitefly. BMC Genomics. 2013;14:241. doi:10.1186/1471-2164-14-241.