Abstract
Extant studies have highlighted numerous barriers to carbon reduction but none seem to have been done in the context of construction process in the UK. Hence, this study aims to bridge this gap by investigating the barriers that are critical to the minimization of construction carbon footprint in the UK. A questionnaire was developed based on the carbon reduction barrier variables identified through a comprehensive literature review and pilot study. Then a survey was conducted amongst construction professionals in the UK and the data obtained were analyzed using mean ranking technique and factor analysis. The mean ranking analysis gave rise to 15 critical barriers to carbon reduction and their factor analysis yielded four dissimilar factors which are, resources and prioritization; policy and standards; risk and commitment; and awareness and complexity. The findings imply that these classifications can be used to describe carbon reduction barriers in the UK. This could stimulate a better comprehension of the critical barriers and provide a foundational basis for further research within the sustainable construction domain. Also, the result of this study could facilitate the need for collaboration between construction stakeholders in the UK to proffer pragmatic solutions to the identified challenges of carbon minimization.
Introduction
The construction process carbon emission has been noted to be underestimated and this can be attributed to the complex nature of construction projects (Wu et al. Citation2019). This deflation has led to the notion that the construction phase emission is quite small (Kong et al. Citation2020; Arogundade et al. Citation2021a). Hence, resulting in it being largely ignored in built environment decarbonization efforts (Arogundade et al. Citation2023). However, according to Acquaye and Duffy (Citation2010), about 20% of Ireland’s total carbon emission in 2005 is linked to construction activities. Also, Huang et al. (Citation2018) reported that in 2009, the construction sector in China, India, and the EU-27 (including the UK) contributed 38%, 30%, and 18% respectively to their country’s national emissions. Within the same period in the UK, construction activities related emission was the largest contributor to direct carbon emission (Huang et al. Citation2018). This indicates that the construction process carbon emission is quite substantial (Li et al. Citation2019) and must not be discounted if the industry’s goal of achieving net zero carbon by 2050 is to be attained (World GBC Citation2016).
Having established the significance of construction process carbon emission, the fragmented nature of construction operations (Fang et al. Citation2019) and the chaotic technique which characterizes the construction process (Yang et al. Citation2018) make it difficult to deploy strategies capable of lessening the impact of construction carbon footprint.
These challenges could slow the sector’s decarbonization which is urgently required due to its huge contribution to the global greenhouse gas emission (Giesekam et al. Citation2018). In view of this, this study seeks to investigate the critical barriers inhibiting the construction process carbon emission reduction. Although, given the complexity of built environment projects, various stakeholders such as architects, engineers, material suppliers and even clients are involved in its execution and they all have a role to play in its decarbonization. For example, the designers could substitute a high carbon-intensive material with low carbon intensive ones to lower a project’s total embodied carbon (Victoria and Perera Citation2018) and the client could adopt an early contractor involvement (ECI) contract model approach as a foresight strategy to take advantage of contractors’ carbon reduction experience to maximize the decarbonization potential of the asset to be built (Arogundade et al. Citation2021b). Nevertheless, if contractors who are responsible for utilizing these materials do not adopt them or do not share their experience, then the carbon reduction efforts by coming up with low-carbon materials or involving them from the project concept stage would have been wasted. Therefore, due to contractors’ vital role in bringing construction design to life (Cheung et al. 2012) and being the main stakeholder involved in the construction process stage of a project, they will be the major focus of this study. The findings of this study will arouse the interest of researchers and industry stakeholders as they become aware of specific barriers hindering construction stage decarbonization in the UK. This could then enable construction stakeholders to devise appropriate measures in overcoming the barriers.
Overview of carbon reduction barriers
The construction industry has been noted to be impervious to change (Tierney and Tennant Citation2015) and over time, contractors have been identified to be slow in implementing strategies capable of reducing carbon during construction projects (Wong et al. Citation2013). Some of the concerns highlighted in literature to be responsible for such behavioural tendencies by contractors are organizational and procedural barrier which according to Mohd-Rahim et al. (Citation2017) represents a major obstacle in the development of green building. This lack of support from top management could equally be linked to the implementation cost of low-carbon technologies especially if there are no incentives for going green (Energy Research Partnership Citation2021; Ng Citation2015). This is because most business leaders are concerned with the bottom-line and the need to ensure that the business continues to be in operation. However, if there is clear legislation enforcing the transition to green practices or utilization of low-carbon technologies (Salama and Hana Citation2010), the leaders of industry might consider it worthy to implement.
Upon legislating the adoption of low-carbon practices, the government will need to back such policy up by piloting it through public procurement frameworks to explore its practicability and to understand the financial implication to contractors (Ng Citation2015). This is because Ng (Citation2015) have stated that contractors fear the jeopardisation of project success when looked at within the context of time, cost and quality that may arise as a result of utilizing unfamiliar or novel low-carbon construction equipment and/or materials. But if such risk is absorbed within the government’s tender, then contractors might be willing to infuse low-carbon plans in their bids and take the risk of implementing low-carbon practices during the execution of infrastructure projects. This could also address the situation of the lack of demand for low-carbon projects (Tierney and Tennant Citation2015). Tierney and Tennant (Citation2015) narrated in their position paper exploring the major challenges confronting the delivery of low-carbon homes in Scotland that home buyers do not prioritize energy efficiency and are not willing to pay high prices to purchase such homes (Ng Citation2015; Salama and Hana Citation2010). A possible explanation for the high prices of low-carbon homes is the additional cost to be incurred for changing standard designs to introduce low-carbon technologies, engage the services of those with carbon reduction skills and purchase low-carbon materials (Tierney and Tennant Citation2015). The cost of hiring carbon reduction specialists and acquiring low-carbon materials leading to inflated construction prices could be due to the current insufficient knowledge of carbon minimization within the construction industry and reduced accessibility to low-carbon materials including construction equipment (Ng Citation2015).
Furthermore, Salama and Hana (Citation2010) found in a study seeking to understand sustainable construction awareness levels amongst construction professionals in the United Arab Emirates (UAE) that the construction stakeholders ranked lack of awareness of the benefits of reducing carbon as the key challenge in implementing green building practices. This mirrors the report of the Energy Research Partnership (Citation2021) which stated that adopting sustainable practices seems not to have any apparent competitive advantage for businesses in the UK. Other barriers highlighted by Salama and Hana (Citation2010) include difficulty in quantifying carbon reduction benefits and the complex nature of construction. This could be due to the broader difficulty in identifying emission hotspots vis-à-vis the fragmented nature of the supply chain (Jackson and Kaesehage Citation2020). In line with this, the findings from the case study research conducted by Jackson and Kaesehage (Citation2020) with participants from the UK construction sector revealed that having a procurement process stating the minimum carbon emission requirements as an industry standard can assist the sector with emission reduction. This is similar to the argument of Sanchez et al. (Citation2015).
These barriers curated from different works and listed in have generally been highlighted in the context of how it affects the whole construction industry. Hence, this study will be looking at how these barriers affect construction projects and most especially the ability of contractors to reduce the carbon footprints within their control in the UK.
Table 1. Carbon reduction barriers.
Research method
The methodological model adopted in this study follows that of Chan et al. (Citation2004) with some modifications. It comprises of literature review, questionnaire development, pilot study and empirical research ().
Figure 1. Research framework for the study (source: adapted from Chan Citation2004).
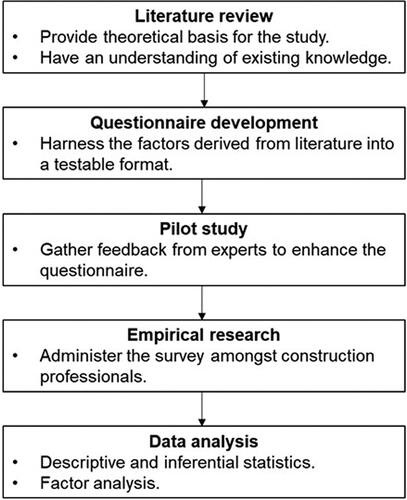
The study began with a comprehensive review of the literature to provide a theoretical basis for the research and extract the initial set of carbon reduction barriers (Darko Citation2019). In extracting the barrier variables, a keyword search was done on the Scopus database. Scopus was chosen as it has been classified by researchers to be the most extensive database in the globe (Oliveros and Vaz-Serra Citation2018; Saad et al. Citation2023). Although, the search initially returned 136 papers but after filtering for relevance to the current study, only five papers were considered eligible to be utilized for developing the barrier variables. This could be as a result of the sparse research in the construction process carbon reduction study area (Arogundade et al. Citation2021b). Furthermore, scholars (Darko and Chan Citation2016; Arogundade et al. Citation2023) have noted that no single study can single-handedly address all the idiosyncratic complexities related to a research keyword when investigating a subject matter. Moreover, the number of papers utilized in this study for obtaining the barrier variables is similar to the number of papers used by Wong et al. (Citation2013) in extracting the carbon reduction variable used for their investigation on contractors response to carbon reduction policies in construction projects. In view of this, the barrier variables obtained from the literature were collated in a questionnaire. For this study, the questionnaire included two other sections. The first section dealt with understanding the respondent’s participation in construction carbon minimization during the delivery of construction projects. This is necessary to ensure responses reflect the sector’s perception as it relates to carbon minimization barriers (Chan et al. Citation2004). The other section captures the profile of the respondents such as industry experience, experience related to carbon reduction, company type and job role. Upon completing the questionnaire development, 10 stakeholders with demonstrable years of experience in construction management were engaged for a pilot study. These stakeholders included two professors, an early career researcher and seven industry experts with knowledge of construction carbon reduction. The sample size of 10 for the pilot study was deemed sufficient since Ajayi (Citation2016) opined that a 10–30 sample size is adequate for a pilot study. The questionnaire was examined for comprehensiveness, vagueness, relevance and suitability of terms used. Based on the valuable comments received from the professionals, the questionnaire was improved and a final list of 16 barrier variables was included in the survey questionnaire (). A five-point Likert scale (1 = not critical, 2 = less critical, 3 = neutral, 4 = critical, and 5 = very critical) was used to obtain respondents’ views on the level of criticality of the barriers hindering the implementation of carbon reduction strategies during the execution of construction projects.
In conducting the survey, an online Google form was employed since it has been used by other scholars and is considered to be easy to use (Ajayi Citation2016). The data was collected by adopting a snowball sampling technique to reach a wider population of the targeted respondents. The technique permits the sharing of the survey with an initial set of respondents as well as through social networks. This approach has been utilized in other studies by Mao et al. (Citation2015). The initial respondents were obtained through the contact list of construction framework association in the UK. This list consisted of both big and small contractors delivering all kinds of construction projects in the UK. The survey link was also shared on LinkedIn. Construction industry associations such as the Institution of Civil Engineers, National Federation of Builders and Civil Engineering Contractors Association were equally approached for data collection. The survey ran for 12 weeks and a total of 48 responses were obtained. Out of the 48, seven responses were removed. One was considered invalid since about 75% of the questionnaire was not completed by the respondent while six were automatically excluded since the key criteria of having participated in a construction project in the UK where reducing carbon was considered or done was not fulfilled. Hence, the study utilized 41 valid responses and the demographic information of the respondents is presented in . The 41 responses were regarded as satisfactory for statistical analysis as the central limit theorem holds true once the sample size is over 30 (Zhao et al. Citation2016). Moreover, Akadiri (Citation2011) argued that the UK construction industry has been noted to be poor in responding to questionnaire surveys and a sample size greater than 30 should be considered as adequate. Furthermore, other researchers within the sustainable construction domain have used a sample size of 30 (Zhao et al. Citation2016) and 32 (Hwang et al. Citation2017). Thus, the sample size for this study can be regarded to be acceptable.
Table 2. Demographic profile of respondents.
Furthermore, the profile of the respondents depicted in implies that the responses reflect the view of the industry.
Data analysis and findings
The data collected were analysed using IBM SPSS version 26. Cronbach’s alpha and Shapiro-Wilk test were first carried out to establish the reliability and normality of the observed data respectively. This is essential to determine the reliability of the questionnaire’s measurement scale (Akintoye et al. Citation2000) and probability distribution of variables in order to select an appropriate statistical method of analysis (Kim Citation2015). The Cronbach’s alpha value for the 16 barrier variables is 0.781 suggesting that the data is reliable for statistical analysis () since according to Bowling (Citation2014), the minimum acceptable threshold is 0.70. As for the Shapiro-Wilk test, the result indicates that the data collected is not normally distributed since all the barriers have p-values lesser than 0.05. Based on this, a non-parametric method was used to conduct further analysis (Hwang et al. Citation2018). The further analysis performed were grouped into two. The first analysis performed was mean analysis and Wilcoxon signed-rank test. This was done to determine the ranking and significance of the variables based on the responses obtained. The second analysis conducted is factor analysis (FA) to explore the intrinsic feature of the carbon reduction barriers. The results of these analyses will be meticulously discussed in successive sections.
Table 3. Ranking of carbon reduction barriers.
Ranking and significance of carbon reduction barriers
The result of the mean analysis of the barriers inhibiting contractors’ ability to minimize construction project carbon footprint is shown in with the barrier codes the same as in . The least ranked barrier ‘lack of demand for low-carbon construction project’ (BR8) has a mean score of 3.20 which is still greater than the median value of 3 when compared with the Likert scale employed for data collection. While this suggests some level of biasness (as ranked by the respondents) towards the barrier being critical to hinder carbon reduction efforts of the contractor, the result of the one-sample Wilcoxon signed rank test implied that the barrier (BR8) is not significant as the p-value is greater than 0.05 (). What this demonstrates is that there is probably demand for low-carbon construction already in the UK and contractors just need to rise to the challenge of delivering such projects. This might not be unexpected since the biggest infrastructure project (high-speed rail line, HS2) in the UK (and indeed all of Europe) at the moment has a carbon reduction target of 50% by 2030 (UK Government Citation2016; HS2 Citation2022). The UK government have equally noted that the project will engage both tier 1 and 2 contractors throughout the construction execution phase (UK Government Citation2016). Even though Tierney and Tennant (Citation2015) noted that there is likely to be a demand issue for low-carbon home construction in the UK. However, the findings of this study seem to negate this as construction professionals in the UK believe there is a demand for such environmental-friendly construction.
‘Lack of demand for low-carbon construction project’ (BR8) is the only insignificant barrier amongst the 16 barriers to construction carbon reduction as per the Wilcoxon signed rank test result. Hence, the remaining 15 barriers were found to be critically significant with mean scores ranging from 3.37 to 4.39 (). The critical barriers with similar mean values were ranked based on their SD figure and the barrier with the lowest SD is given the highest rank. The top five barriers ranked as the most critical by the respondents are: ‘lack of support from top management’; ‘increase in construction project cost due to carbon reduction efforts’; ‘lack of collaboration between clients, their consultants and contractors’; ‘non-involvement during the design and specification stage of the project’; and ‘lack of clear carbon reduction regulation by the government’; in that order. The ranking of these five barriers as the most critical that could impede contractors in achieving construction project decarbonization in the UK is somewhat expected. This is because Tierney and Tennant (Citation2015) opined that low-carbon construction could lead to more costs which clients might not want to bear and studies have shown that the profit margin of contractors is quite low (Moffat Citation2020). Therefore, contractors might not want to absorb the cost increase that may arise from the implementation of low-carbon practices during the delivery of infrastructure projects. Also, ECI and increased collaboration between all construction stakeholders have been touted to improve both the financial and environmental output of a project. For instance, in a road construction project in Australia, Sanchez et al. (Citation2015) pointed out that the utilization of the ECI strategy resulted in the avoidance of hauling about 30% of material thereby saving fuel and its associated carbon emission. A similar strategy was reported to have saved tonnes of virgin materials and concrete resulting in 21% CO2e savings on a road project in the UK (Manidaki et al. Citation2016a). This indicates that if such an opportunity for early collaboration was lacking, there will not be an avenue to suggest and implement measures leading to this sort of emission reduction. Furthermore, as found out in this study that lack of top management support is the topmost critical barrier to construction carbon reduction, several studies (Rais et al. Citation2018; Khaderi et al. Citation2022) done in both developed and developing countries also support these findings. Lastly, the lack of clear regulation on construction carbon reduction being ranked as one of the top five critical barriers by UK construction professionals is in tandem with the observation of the UK’s House of Commons Environmental Audit Committee. The committee noted that the absence of such a policy is responsible for the lack of progress in reducing embodied carbon of built environment projects because operational emissions seem to have largely been minimized due to policy targeted at it (Environmental Audit Committee Citation2022). Hence, the government needs to urgently put forward clear regulations and guidelines directed towards embodied carbon if the UK is to meet its net zero target by 2050 (Environmental Audit Committee Citation2022).
Factor analysis of carbon reduction barriers
The FA was performed on barriers of construction carbon reduction to establish their latent variables. Out of the 16 barriers, one barrier (BR8) was excluded since it was discovered to be insignificant based on the result of the Wilcoxon signed rank test (). Hence, the FA was carried out on the remaining 15 barriers that were considered critical and significant. In conducting the FA, principal component analysis and varimax rotation were used with minimum factor loading set to 0.50. This approach was utilized because it has been widely used in construction management studies (Wong et al. Citation2016) and it is capable of generating an easily interpretable rotated component matrix (Akintoye Citation2000; Cheung et al. Citation2011). Also, to determine the factors to retain, Kaiser’s criterion which states that the eigenvalue of factors should be above 1 was adopted (Pallant Citation2020. p. 184).
Before proceeding with the FA, the suitability of the data was inspected. KMO and Bartlett’s test of sphericity was utilized for this purpose. The KMO value was 0.561. Albeit small, it is greater than the allowable threshold of 0.50 and according to Field (Citation2005, p. 647), it is a value lower than 0.50 that should warrant the omission of some variables or the collection of more data. Thus, based on this study’s KMO value, the factor analysis to be conducted is expected to yield reliable and dissimilar factors (Field Citation2018, p. 798). For the Bartlett’s test, a chi-square value of 256.768 at a significant level of 0.000 was returned implying that the correlation matrix is not an identity matrix (Field Citation2005, p. 660). The outcome of the KMO and Bartlett’s test indicates that FA is appropriate. The application of factor analysis revealed four factors whose eigenvalues were higher than 1. These four clusters accounted for 66.316% of the total variance and which is greater than the minimum value of 60% (Hair et al. Citation2014, p. 109; Chan Citation2019). But one barrier ‘difficulty in quantifying carbon reduction benefits’ (BR11) was loading on two factors. Therefore, it was taken out and the analysis was repeated. The new analysis result is presented in with four clusters still retained and a slight improvement in the cumulative variance (66.890%) was noticed. The remaining 14 critical barriers all have factor loadings above 0.50 and one variable has a negative factor loading of −0.628. According to (Field Citation2018, p. 823), the negative sign does not matter, and the factor is as important as those with a positive sign since the factor loading value is greater than 0.50 which is the most important criterion (Chan et al. Citation2010). The four clusters are then labelled based on the characteristics of their underlying variables and they are:
Table 4. Factor analysis for the carbon reduction barriers.
Cluster 1: Resources and prioritization.
Cluster 2: Policy and standards.
Cluster 3: Risk and commitment.
Cluster 4: Awareness and complexity.
Discussion of factor analysis results
Resources and prioritization
This group pinpoints the essential purpose of human, material and knowledge resources in delivering a low-carbon construction project by contractors. Given the interrelationship that exists between these resources and their key contribution to the success of any built environment projects (Ng Citation2015), it is not surprising that this group accounts for the highest variance with a value of 25.397% (). As seen in , this cluster has five underlying critical barriers.
Even though lack of support from top management had a low factor loading in the group, it is the most exigent barrier in the group based on the findings of this study ().
The lack of backing from senior management could be attributed to various factors such as a likely increase in project cost if low-carbon materials are adopted, minimal knowledge and awareness of decarbonization practices, no incentive to reduce the carbon impact of projects, no demand for low-carbon construction, to mention but a few. The factors highlighted are related to or could impact the overall profitability of an organization. Therefore, since the major goal of top management is to maximize and prioritize resources while improving a company’s financial well-being (Galbraith et al. Citation1975; Jensen Citation2010), they might not be willing to engage in practices that could jeopardize this. Nonetheless, the collocation of the barriers on carbon reduction knowledge and awareness of decarbonization technologies with top management support in this cluster highlights the interdependence of these critical barriers. According to Olawumi and Chan (Citation2020), the execution of sustainability practices within the construction sector requires the continuous training of all stakeholders due to the constant emergence and development of new emission abatement technologies and practices. Most times, approval of senior management is often required to participate in such training due to the resources (time and/or financial) required. Hitherto, if the aforesaid consent is lacking, then the capacity building of workers will be affected, thereby hindering the depletion of carbon during construction. Meanwhile, as regards the possibility of limited or no demand for low-carbon projects influencing top management decisions to support the adoption of low-carbon practices, currently in the UK, this does not seem likely as the result of this study negates such perception (see section “Ranking and significance of carbon reduction barriers”).
Additionally, the failure to engage contractors during the design and specification stage has the least factor loading () in this cluster but is ranked amongst the top five critical barriers as per the result of this study (see ). Thus, its importance in impeding the minimization of construction carbon by contractors cannot be overlooked. This aligns with the observation of previous studies. Arogundade et al. (Citation2021b) and Kadefors et al. (Citation2021) argued that ECI has the potential to lead to a considerable decrease in construction carbon. Also, Pomponi et al. (Citation2020) accentuated that construction carbon mitigation is best attained early on in a project lifecycle. To achieve this, stakeholders have emphasized the need for collaboration throughout the lifecycle of a project (Manidaki et al. Citation2016a) which is the feature of the ECI strategy. Again, this is crucial because once the design and specification of materials for a project are completed, its alteration to imbibe alternative material would be difficult for a contractor. Thereby limiting the scope of whatever low-carbon initiative to be implemented by the contractor (Ng Citation2015).
Another critical barrier which has a high factor loading (0.819) in this group is the limited availability of materials and equipment with high carbon efficiency. As found in the result of this study with UK contractors that this barrier is crucial to their carbon minimization endeavour (), Ng (Citation2015) equally reported similar findings in Hong Kong. But a report by the UK’s House of Common Environment Audit Committee stated otherwise. The committee believe that there is availability of low-carbon product and that what is lacking is an incentive by the government to promote the adoption of such products (Environmental Audit Committee Citation2022). Perhaps, this could be because some of these net zero carbon technologies are rather expensive and still have low market penetration (Gillingham and Sweeney Citation2012; Preston and Lehne Citation2018). Thus, requiring the support of the government to achieve economies of scale.
Policy and standards
This cluster explains 17.282% of the total variance and comprises policy- and standard-related barriers at both organizational and government level. It is the second most vital cluster after the resources and prioritization group based on the result of the factor analysis (). This appears to support the assumption that policies have a link to resource allocation (Andrews and Cingano Citation2014). The policy and standard group consist of four critical barriers. Out of the four critical barriers contained in this group, two of them are amongst the top five barriers identified as most critical based on the respondents’ ranking (). Whilst the lack of collaboration between construction project stakeholders has the least factor loading (0.541), it remains the most significant barrier in this cluster as per the result of this study followed by the absence of explicit government regulation on construction carbon reduction (). The criticality of collaboration in achieving low-carbon construction cannot be overemphasized. Sopp (Citation2018) mentioned that collaborative working can aid innovation, enhance risk management and improve project performance. Within the construction sector, the British Standards Institute equally stressed the need for stakeholders to collaborate in managing the carbon impact of infrastructure projects (Manidaki et al. Citation2016b). This call was supported with the publication of a standard termed PAS 2080 with corresponding guidance by the Construction Leadership Council. This elucidates the importance given to collaborative working and Ey et al. (Citation2014) argued that the absence of it could result in disputes and poor project performance.
In the UK, the barrier, lack of clear carbon reduction regulation by the government has been ranked rather high in comparison to the result of the study by Salama and Hana (Citation2010) in the UAE. This suggests that UK contractors are more concerned about the policy direction of the government in influencing their behaviour as it relates to the minimization of the carbon footprint of built environment projects. This is not unexpected because contracting organizations like most businesses would require some level of assurance by the government as regards the mandatory procurement of low-carbon infrastructure before investments in new carbon-efficient technologies can be made (Office of Fair Trading Citation2009). Albeit counterintuitive since this study has established that lack of demand for low-carbon projects is not a critical barrier in the UK (section 4.1), yet if it is not supported by a government policy, the pace of low-carbon infrastructure development will be slow (Environmental Audit Committee Citation2022). Once this happens, contractors will be left with no choice but to institute an organizational policy on carbon reduction as well as develop a carbon management plan for construction projects which will tally with their organization’s policy. In addendum, it has been noted that carbon reduction regulation can strongly influence a behavioural change in construction stakeholders to reduce the carbon footprints of infrastructure projects (Wong et al. Citation2015). Therefore, without such a policy from the government, contractors might not work on lowering construction project carbon footprint.
Risk and commitment
This group consist of three critical barriers underscoring the role of project success in the dimensions of cost, time, and quality. The group accounts for 14.205% of the total variance ().
Again, as per the findings of this study, the barrier ‘increase in construction project cost due to carbon reduction efforts’ is the most significant in this risk and commitment group due to its high rank (second) by the respondents () even though it has the lowest factor loading (0.692) in the cluster. It is quite interesting to discover in this study that this barrier is still very relevant in mitigating the carbon emission of construction projects in the UK. This is so because, in the study conducted by Sorrell in 2003, an increase in project cost was identified as one of the major barriers impeding the construction of low-carbon buildings in the UK (Sorrell Citation2003). The author further argued that as of then, the reforms happening within the UK construction industry championed by the government were not linked to the UK climate policy even though other barriers identified by the author were addressed by the reform (Sorrell Citation2003). While project cost increase being one of the most critical barriers to decreasing construction carbon is not synonymous to the UK alone as similar findings were reported in the UAE (Salama and Hana Citation2010) and Hong Kong (Ng Citation2015). The government (UK in this case) might need to lead in this aspect by instituting incentive schemes to overcome this barrier as suggested by Ng (Citation2015). Construction clients especially those procuring public infrastructure projects for the government might equally look at initiating such incentive schemes to abate the risk associated with project success if carbon reduction measures implemented by contractors have an undesirable outcome. Even though project success means different things to various individuals (Chan Citation2001; Chan and Chan Citation2004) and it is dependent on the client criteria, the success of a construction project remains the most paramount factor to contractors (Love et al. Citation1998). Thus, if the condition to lessen construction carbon emission is part of a client’s requirement, initiatives to support this obligation should be included in the tendering process for a procurement framework to ensure that contractors become willing to take the risk.
Awareness and complexity
The last grouping revealed by the FA performed on the critical barriers explained just about 10% of the total variance and they are barriers linked with the value of carbon reduction and project complexity ().
According to the findings of this study, the complexity of construction projects has been cited by contractors as one of the obstacles to reducing the carbon impact of a project in the UK. This finding agrees with the observation of Bentil et al. (Citation2017) who mentioned that the complex nature of construction projects hinders project success. This can be exacerbated by the construction industry’s fragmented supply chain which can fetter innovation (Willan Citation2019). This is so because the execution of most built environment projects involves employing a multitude of suppliers and subcontractors. For instance, Ko (Citation2010) stated that supply chain fragmentation could hamper a construction project’s early connection to the grid which is one of the strategies that could be adopted to reduce carbon. This is due to the coordination required amongst them before the grid connection can be done which could result in delay if proper coordination is lacking (Ko Citation2010). Consequently, it is imperative to consider processes that could aid in the easy management of the suppliers being engaged. This view is buttressed by Burbridge (Citation2012) who noted that efficient management and monitoring of construction supply chain activities could assist in project decarbonization. Since most carbon associated with construction processes is emitted by the actions of the supply chain. Therefore, if a supplier can manage its carbon footprint, this would have an impact on the carbon target of the project which the supplier is involved in. The management of carbon emissions by a supplier or subcontractor is contingent on their awareness of the benefit of doing so. The same applies to the main contractor and indeed all the stakeholders taking part in a construction project. Maybe that is why both barriers are collocated in this group. In line with this, contractors are urged to constantly seek for ways in updating their knowledge to improve project outcomes. This call is necessary because based on the result of this study, about 85% of the respondent have more than five years of experience working within the construction industry while only 37% have upward of five years’ experience related to carbon reduction in construction projects.
Theoretical and practical contributions of the study
The outcome of the study illustrates that construction carbon reduction barriers can be categorized into four distinct clusters. This engenders a better comprehension of the critical barriers impeding the minimization of carbon during the delivery of construction projects. This classification could provide a foundational basis for further research within the sustainable construction domain and deepen the knowledge of researchers in this research area.
Furthermore, the findings of the study would be beneficial to construction clients and policymakers in strengthening their understanding of the carbon reduction challenges which contractors experience while executing projects. Thus, it could facilitate the need for collaboration between these construction stakeholders in order to proffer pragmatic solutions to the identified challenges. For instance, Sanchez et al. (Citation2014) suggested that the development and implementation of consistent systems – carbon management systems in the case of this study – could enable the translation of carbon reduction goals into practice.
Conclusion and limitations
This study has elucidated significant critical barriers likely to hinder contractors to minimize the carbon impact of construction projects in the UK. The findings of this study conducted amongst 41 construction professionals revealed that of the 16 barriers presented to the respondents, 15 of them were found to be significant according to the result of the Wilcoxon signed-rank test. FA was then carried out to establish the underlying factors of these 15 critical barriers. The result of the FA returned four barrier clusters namely: resources and prioritization; policy and standards; risk and commitment; and awareness and complexity. Construction stakeholders in the UK will need to pay attention to these four barrier categories and implement policies and strategies that would assist in overcoming these identified challenges. Such strategies could include the provision of training support by construction clients while also incentivizing the uptake of innovative approaches by contractors in reducing construction carbon emissions. In addition, the government can institute policies targeted at construction process carbon reduction just as it has for operational emissions.
Even though the study objective has been realized, the research has few limitations. Firstly, although the sample size was considered adequate for the study, it is appreciated to be small. This is probably due to the requirement that respondents must have worked on a project where carbon reduction was implemented. Given the rise in client requirements to reduce the carbon footprint of construction projects, more contractors are likely to become skilled in carbon reduction, therefore, have experience in construction carbon minimization. Hence, future studies can be conducted, and more respondents from contracting organizations could be involved. Secondly, the research was conducted amongst UK construction professionals and the findings were interpreted accordingly within this context. Hence, the generalization of the study outcome beyond the UK should be done with caution because of the peculiarities of the construction process in other geographies. Future research related to this study could be replicated within the UK or elsewhere while involving other construction stakeholders and the findings juxtaposed with the result of this study.
Acknowledgements
This study forms part of a much larger doctoral study on construction carbon reduction. The authors wish to acknowledge the School of Built Environment, Engineering and Computing, Leeds Beckett University and YORhub for their partial funding support for this study.
Disclosure statement
No potential conflict of interest was reported by the author(s).
References
- Acquaye AA, Duffy AP. 2010. Input-output analysis of Irish construction sector greenhouse gas emissions. Build Environ. 45(3):784–791. doi: 10.1016/j.buildenv.2009.08.022.
- Ajayi SO. 2016. Design, procurement and construction strategies for minimizing waste in construction projects. Bristol: University of the West of England.
- Akadiri OP. 2011. Development of a multi-criteria approach for the selection of sustainable materials for building projects [PhD Thesis]. University of Wolverhampton, pp. 1–437.
- Akintoye A. 2000. Analysis of factors influencing project cost estimating practice. Constr Manag Econ. 18(1):77–89. doi: 10.1080/014461900370979.
- Akintoye A, McIntosh G, Fitzgerald E. 2000. A survey of supply chain collaboration and management in the UK construction industry. Euro J Purch Supply Manag. 6(3–4):159–168. doi: 10.1016/S0969-7012(00)00012-5.
- Andrews D, Cingano F. 2014. Public policy and resource allocation: evidence from firms in OECD Countries. Econ Policy. 29(78):253–296. doi: 10.1111/1468-0327.12028.
- Arogundade S, Dulaimi M, Ajayi S. 2021a. Carbon reduction during building construction projects – trend mapping from construction journals. In: Dulaimi, M. Elhag, T. editor., CIB International Conference on Smart Built Environment, 2021. CIB.
- Arogundade S, Dulaimi M, Ajayi S. 2021b. The role of contractors in reducing carbon during construction – a preliminary study. In: Gorse, C., Dickinson, I. & Drotleff, B. editor. The 7th International Sustainable Ecological Engineering Design for Society Conference, 2021. p. 702.
- Arogundade S, Dulaimi M, Ajayi S. 2023. Holistic review of construction process carbon-reduction measures: a systematic literature review approach. Buildings. 13(7):1780. doi: 10.3390/buildings13071780.
- Bentil E, Nana-Addy E, Asare EK, Fokuo-Kusi A. 2017. The level of existence and impact of cost and time overruns of building construction projects in Ghana. Reserch Gate. 9(1):36–46.
- Bowling A. 2014. Research methods in health: investigating health and health services. New York: McGraw Hill.
- Burbridge D. 2012. Building a lower-carbon construction industry [Online]. Carbon Trust; [accessed 2023 Jan 2]. https://www.carbontrust.com/news-and-insights/insights/building-a-lower-carbon-construction-industry.
- Chan A. 2001. Framework for measuring success of construction projects value alignment process for project delivery framework for measuring success of construction projects. Pp. 1–21.
- Chan APC, Chan APL. 2004. Key performance indicators for measuring construction success. Benchmarking. 11(2):203–221. doi: 10.1108/14635770410532624.
- Chan APC, Chan DWM, Chiang YH, Tang BS, Chan EHW, Ho KSK. 2004. Exploring critical success factors for partnering in construction projects. J Constr Eng Manage. 130(2):188–198. doi: 10.1061/(ASCE)0733-9364(2004)130:2(188).
- Chan APC, Lam PT, Chan DW, Cheung E, Ke Y. 2010. Critical success factors for PPPs in infrastructure developments: Chinese perspective. J Constr Eng Manage. 136(5):484–494. doi: 10.1061/(asce)co.1943-7862.0000152.
- Chan DWM. 2019. Sustainable building maintenance for safer and healthier cities: effective strategies for implementing the Mandatory Building Inspection Scheme (MBIS) in Hong Kong. J Build Eng. 24:100737. doi: 10.1016/j.jobe.2019.100737.
- Cheun SO, Wong PSP, Lam AL. 2012. An investigation of the relationship between organizational culture and the performance of construction organizations. J Bus Econ Manag. 13(4):688–704. doi: 10.3846/16111699.2011.620157.
- Cheung SO, Wong PSP, Wu AWY. 2011. Towards an organizational culture framework in construction. Int J Project Manage. 29(1):33–44. doi: 10.1016/j.ijproman.2010.01.014.
- Darko A. 2019. Adoption of green building technologies in Ghana: development of a model of green building technologies and issues influencing their adoption. Ghana: The Hong Kong Polytechnic University.
- Darko A, Chan APC. 2016. Critical analysis of green building research trend in construction journals. Habitat Int. 57:53–63. doi: 10.1016/j.habitatint.2016.07.001.
- Energy Research Partnership. 2021. How behaviour change will unlock net zero.
- Environmental Audit Committee. 2022. Building to Net Zero : costing carbon in construction. (May).
- Ey W, Zuo J, Han S. 2014. Barriers and challenges of collaborative procurements: an exploratory study. Int J Constr Manag. 14(3):148–155. doi: 10.1080/15623599.2014.922725.
- Fang Y, Lu X, Zhang Y. 2019. Carbon emissions analysis for construction process. In: ICCREM 2019, 2019. American Society of Civil Engineers, pp. 648–654. doi: 10.1061/9780784482308.075.
- Field A. 2018. Discovering statistics using IBM SPSS statistics, 5th ed. Thousand Oaks: SAGE Publications, Inc.
- Field AP. 2005. Discovering statistics using SPSS. Thousand Oaks: SAGE Publications, Inc.
- Galbraith JK, Weidenbaum ML, Hession CH, Deckard B, Sherman H, Thompson CC. 1975. Economics and the Public Purpose. J Econ Issues. 9(1):87–100. (doi: 10.1080/00213624.1975.11503256.
- Giesekam J, Tingley DD, Cotton I. 2018. Aligning carbon targets for construction with (inter)national climate change mitigation commitments. Energy Build. 165:106–117. doi: 10.1016/j.enbuild.2018.01.023.
- Gillingham K, Sweeney J. 2012. Barriers to implementing low carbon technologies paper prepared for the stanford-RFF Climate Policy Conference Kenneth Gillingham. (Eia 2010), pp. 1–26.
- Hair JF, Black WC, Babin BJ, Anderson RE. 2014. Multivariate data analysis, 7th ed. Essex: Pearson.
- HS2. 2022. Net Zero Carbon Plan: a Cleaner, Greener Future.
- Huang L, Krigsvoll G, Johansen F, Liu Y, Zhang X. 2018. Carbon emission of global construction sector. Renewable Sustainable Energy Rev. 81:1906–1916. doi: 10.1016/j.rser.2017.06.001.
- Hwang B-G, Zhu L, Ming JTT. 2017. Factors affecting productivity in green building construction projects: the case of Singapore. J Manage Eng. 33(3):e0000499. doi: 10.1061/(ASCE)ME.1943-5479.0000499.
- Hwang BG, Shan M, Looi KY. 2018. Key constraints and mitigation strategies for prefabricated prefinished volumetric construction. J Cleaner Prod. 183:183–193. doi: 10.1016/j.jclepro.2018.02.136.
- Jackson DJ, Kaesehage K. 2020. Addressing the challenges of integrating carbon calculation tools in the construction industry. Bus Strat Env. 29(8):2973–2983. doi: 10.1002/bse.2551.
- Jensen MC. 2010. Value maximization, stakeholder theory, and the corporate objective function. J Appl Corp Finance. 22(1):32–42. doi: 10.1111/j.1745-6622.2010.00259.x.
- Kadefors A, Lingegård S, Uppenberg S, Alkan-Olsson J, Balian D. 2021. Designing and implementing procurement requirements for carbon reduction in infrastructure construction–international overview and experiences. J Environ Plann Manage. 64(4):611–634. doi: 10.1080/09640568.2020.1778453.
- Khaderi SS, Yub Y, Bakri AS, Abd Shukor AS. 2022. Green procurement implementation in construction industry: analysis of developer’s challenges. IOP Conf Ser: Earth Environ Sci. 1067(1):012052.
- Kim TK. 2015. T Test as a parametric statistic. Korean J Anesthesiol. 68(6):540–546. doi: 10.4097/kjae.2015.68.6.540.
- Ko J. 2010. Carbon: reducing the Footprint of the Construction Process. p. 64.
- Kong A, Kang H, He S, Li N, Wang W. 2020. Study on the carbon emissions in the whole construction process of prefabricated floor slab. Appl Sci 10(7):2326. doi: 10.3390/app10072326.
- Li YL, Han MY, Liu SY, Chen GQ. 2019. Energy consumption and greenhouse gas emissions by buildings: a multi-scale perspective. Build Environ. 151(January):240–250. doi: 10.1016/j.buildenv.2018.11.003.
- Love PED, Skitmore M, Earl G. 1998. Selecting a suitable procurement method for a building project. Constr Manag Econ. 16(2):221–233. doi: 10.1080/014461998372501.
- Manidaki M, Depala P, Ellis T, Steele K, Roe D. 2016a. Guidance Document for PAS 2080 20 February 2021.
- Manidaki M, Depala P, Ellis T, Steele K, Roe D. 2016b. PAS 2080:2016 – Carbon Management in Infrastructure.
- Mao C, Shen Q, Pan W, Ye K. 2015. Major barriers to off-site construction: the developer’s perspective in China. J Manage Eng. 31(3):e000246. doi: 10.1061/(ASCE)ME.1943-5479.0000246.
- Moffat O. 2020. The profit problem in construction. Gement; [accessed 2022 August 2] https://gement.co.uk/the-profit-problem-in-construction/.
- Mohd-Rahim FA, Pirotti A, Keshavarzsaleh A, Zainon N, Zakaria N. 2017. Green construction project: a critical review of retrofitting awarded green buildings in Malaysia. J Design Built Environ. 17:11–26. doi: 10.22452/jdbe.sp2017no1.2.
- Ng ST. 2015. Reducing the embodied carbon of construction projects through a carbon emission encompassed tender. WIT Transactions on Ecology and the Environment, 195, pp. 403–413. doi: 10.2495/ESUS150341.
- Office of Fair Trading. 2009. Government in markets. Oxford: Regulatory Policy Institute, p. 1–12.
- Olawumi TO, Chan DWM. 2020. Key drivers for smart and sustainable practices in the built environment. Eng Const Arch Mang. 27(6):1257–1281. doi: 10.1108/ECAM-06-2019-0305.
- Oliveros J, Vaz-Serra P. 2018. Construction project manager skills: a systematic literature review. In: Rajagopalan, P. & Andamon, M. editor. Engaging Architectural Science: Meeting the Challenges of Higher Density: 52nd International Conference of the Architectural Science Association, 2018. p. 185–192.
- Pallant J. 2020. SPSS Survival manual: a step by step guide to data analysis using SPSS. 4th ed. Australia: Allen & Unwin.
- Pomponi F, Giesekam J, Hart J, D’Amico B. 2020. Embodied carbon status quo and suggested roadmap. Report to Zero Waste Scotland. (March).
- Preston F, Lehne J. 2018. Making concrete change innovation in low-carbon cement and concrete. Chatham House Report pp. 1–122.
- Rais SLA, Bidin ZA, Bohari AAM, Saferi MM. 2018. The possible challenges of green procurement implementation. IOP Conf Ser: Mater Sci Eng. 429(1):012023. doi: 10.1088/1757-899X/429/1/012023.
- Saad AM, Dulaimi M, Zulu SL. 2023. A systematic review of the business contingencies influencing broader adoption: modern Methods of Construction (MMC). Buildings. 13(4):878. doi: 10.3390/buildings13040878.
- Salama M, Hana AR. 2010. Green buildings and sustainable construction in the United Arab Emirates. Association of Researchers in Construction Management. ARCOM 2010 – Proceedings of the 26th Annual Conference (September 2010), p. 1397–1405.
- Sanchez AX, Lehtiranta L, Hampson KD, Kenley R. 2014. Evaluation framework for green procurement in road construction. Smart Sust Built Environ. 3(2):153–169. doi: 10.1108/SASBE-05-2013-0028.
- Sanchez AX, Lehtiranta LM, Hampson KD. 2015. Use of contract models to improve environmental outcomes in transport infrastructure construction. J Environ Plann Manage. 58(11):1923–1943. doi: 10.1080/09640568.2014.969832.
- Sopp M. 2018. Collaborative Working and ISO 44001 [Online]. Croner-i; [accessed 2023 Jan 1] https://app.croneri.co.uk/feature-articles/collaborative-working-and-iso-44001#po-heading-id_oXDcgd7BRUGZM3GVYJmA0w.
- Sorrell S. 2003. Making the link: climate policy and the reform of the UK construction industry. Energy Policy. 31(9):865–878. doi: 10.1016/S0301-4215(02)00130-1.
- Tierney G, Tennant S. 2015. House building in Scotland: the sustainability performance gap. Proceedings of the 31st Annual Association of Researchers in Construction Management Conference, ARCOM 2015 (September), p. 317–326.
- UK Government. 2016. HS2 supply chain opportunities.
- Victoria MF, Perera S. 2018. Managing embodied carbon in buildings: a Pareto approach. Built Environ Project Asset Mang. 8(5):504–514. doi: 10.1108/BEPAM-10-2017-0095.
- Willan CM. 2019. Life in the gap: how does a construction company respond to the challenge of targets for energy and carbon in-use? London: University College London.
- Wong JKW, Chan JKS, Wadu MJ. 2016. Facilitating effective green procurement in construction projects: an empirical study of the enablers. J Cleaner Prod. 135:859–871. doi: 10.1016/j.jclepro.2016.07.001.
- Wong PSP, Ng STT, Shahidi M. 2013. Towards understanding the contractor’s response to carbon reduction policies in the construction projects. Int J Project Manage. 31(7):1042–1056. doi: 10.1016/j.ijproman.2012.11.004.
- Wong PSP, Zapantis J, Owczarek A, Spinozzi J, Kefalianos Z, Murison M. 2015. The drivers and strategies of carbon reduction in projects: perceptions of the Australian Construction Practitioners. Int J Pro Organ Manag. 7(4):307–326. doi: 10.1504/IJPOM.2015.073142.
- World GBC. 2016. World Green Building Council: Annual Report 2015/2016. pp. 1–19.
- Wu W, Sun P, Zhou H. 2019. The case study of carbon emission in building construction process. IOP Conf Ser: Earth Environ Sci. 371(2):022011. doi: 10.1088/1755-1315/371/2/022011.
- Yang X, Hu M, Wu J, Zhao B. 2018. Building-information-modeling enabled life cycle assessment, a case study on carbon footprint accounting for a residential building in China. J Cleaner Prod. 183:729–743. doi: 10.1016/j.jclepro.2018.02.070.
- Zhao X, Hwang BG, Lee HN. 2016. Identifying critical leadership styles of project managers for green building projects. Int J Const Manag. 16(2):150–160. doi: 10.1080/15623599.2015.1130602.