Abstract
Hydrophilic monomer methacrylic acid was copolymerized with hydrophobic monomer butylmethacrylate in different molar ratio, using AIBNfree radical initiator. Successful polymerization was confirmed by FTIR and 1H NMR, while DSC and XRD revealed the amorphous nature of the polymers. Copolymers were highly hemocompatible. The polymers showed pH-dependent swelling and erosion which increased with increase in pH. As the amount of methacrylic acid increased, the swelling and erosion also increased. Polymers were used for the microencapsulation of a model anti-inflammatory drug, aceclofenac by an oil-in-oil solvent evaporation method. Microparticles were characterized using FTIR, XRD, DSC, and SEM analysis. The negatively charged particles were almost spherical in shape. The drug release was best explained by zero-order kinetics with anomalous release mechanism. Less than 1% of drug was released at pH 1.2 while the bulk of the drug load was released at pH 6.8 or above, confirming the colon-targeting nature. The microparticles showed significant lowering in myeloperoxidase activity in trinitrobenzene sulfonic acid-induced colitis rat model. There was a significant lowering in the severity of disease symptoms and colon-to-body weight ratio. The histopathological studies also confirmed successful treatment with microparticle formulation.
1. Introduction
Intestinal bowel diseases (IBD) comprise two relapsing and remitting inflammatory disorders, namely ulcerative colitis (UC) and Crohn’s Disease (CD). In CD, there is transmural inflammation of the entire gastrointestinal tract; while in UC, there are ulcers and inflammation, mainly in the mucosa and submucosa of the colon.[Citation1,2] IBD, which are possible risk factor for colon cancer, are increasing rapidly worldwide and account for almost 15% of all deaths.[Citation3–5] The incidence of IBD is increasing mainly in the developing countries and its prevalence in Asia and Middle Eastern countries is 4.9–168.3 per 100,000 persons.[Citation6] The etiology of IBD is not very well understood. However, the known factors which play important role in the etiology are genetic, immunological, environmental, diet, depression, stress, etc.[Citation7] The inflammatory cytokines involved in the inflammation associated with IBD are IL-1β, TNF-α, IL-6, IL-12, etc. Studies have revealed that an increased level of TNF-α caused bleeding and necrosis of intestine in colitis.[Citation8,9] IBD is a lifelong ailment and requires ongoing medication. The current therapies for the treatment of IBD includes amino salicylates, glucocorticoids, and immunosuppressants.[Citation10,11] Treatment for longer periods with high doses of these drugs causes various side effects.[Citation12] Thus, there is a need to target drugs directly to the site of inflammation, which would maximize the local concentration of the drug and minimize the dose required for the treatment, and thereby minimize the side effects.
Different types of approaches have been exploited to target drugs to the colon, like pH-sensitive systems, time-controlled systems, microflora sensitive systems, and pulsatile systems.[Citation13,14] The pH-sensitive drug delivery systems are based on the difference in pH of colon as compared to other sites in gastrointestinal tract and are most frequently used for colon targeting.[Citation13,14] Acrylic and methacrylic polymers are very attractive candidates for such drug delivery systems as their mechanical and chemical properties can be very easily modified by changing the length or chemical nature of the backbone and pendant groups. Moreover, these polymeric materials can sense a change in the surrounding pH and induce structural changes by themselves and release the drug in a desired manner.[Citation15–19] New pH-sensitive polymers have been designed based on the fact that addition of hydrophobic monomers to the hydrophilic backbone modifies the carboxylic acid density, which in turn imparts pH sensitivity.[Citation20,21]
Methacrylic acid and butyl methacrylate are very frequently used monomers in the preparation of stimuli sensitive polymers. However, only few studies have been reported on the copolymerization of methacrylic acid and butyl methacrylate. Kanevskaya et al. have studied the effects of the supermolecular structure of the coating of methacrylic acid and butyl methacrylate copolymers on the thermal-oxidative and photochemical degradation.[Citation22] Konstantinopolskaya et al. have studied the morphology of films of methacrylic acid–butyl methacrylate copolymer using electron microscopy technique.[Citation23] Qui et al. have fabricated butyl methacrylate–methacrylic acid copolymer shell microcapsules containing n-alkane for the storage of thermal energy.[Citation24] Copolymerization of methacrylic acid with butylmethacrylate in water–dioxan mixture was investigated by Bezuglyi et al. [Citation25].
From the literature, no exact evidence was found on the use of methacrylic acid–butyl methacrylate copolymer as drug delivery vehicle. Taking the aforementioned information into account, attempts have been made to synthesize copolymers of methacrylic acid and butyl methacrylate in different molar ratios and study the swelling and erosion of the copolymers in simulated gastrointestinal pH condition followed by characterization of the selected copolymers by FTIR, 1H NMR, PXRD, and DSC. Gel permeation chromatography was used to determine the average molecular weights of copolymers.
Non-steroidal anti-inflammatory drugs (NSAIDs) are an obvious choice for the treatment of disorders caused by inflammation.[Citation26] Aceclofenac, 2-[(2,6-dichlorophenylamino)phenyl] acetoxy acetic acid, acts by preferential selective COX-2 inhibition after conversion into active metabolite. Aceclofenac as such acts by inhibiting the secretion of TNF-α and IL-1.[Citation27,28] Therefore, targeting aceclofenac directly to the inflamed colon may be promising in the treatment of IBD. It has been observed that multiparticulate formulations have shown better results in the treatment of IBD, as compared to conventional single unit formulations.[Citation29,30] Therefore, attempts have also been made to prepare microparticles of aceclofenac, a model NSAID, with the selected copolymers and characterize the microparticles by FTIR, XRD, DSC, and SEM. Drug loading was determined and in vitro release of the entrapped drug was studied. The formulation was also tested for in vivo activity in TNBS-induced colitis rat model and different parameters like disease activity score, colon-to-body weight ratio, and myeloperoxidase levels were determined.
2. Materials and methods
2.1. Materials
Aceclofenac was received from Ranbaxy Research Laboratories (Gurgaon, India) as a gift sample. Methacrylic acid was purchased from SRL, India, and butyl methacrylate was purchased from Merck, India. Both the monomers were purified by vacuum distillation prior to use. The polymerization initiator αα’-azobis-isobutyronitrile (AIBN) was purchased from Loba Chemie Pvt. Ltd, Mumbai, India, and purified by recrystallization before use. Tetrahydrofuran (THF), dichloromethane (DCM), petroleum ether (boiling range 40–60 °C), methanol, acetone, n-hexane, and light liquid paraffin of analytical grade were purchased from Loba Chemie Pvt. Ltd., Mumbai, India and used as received. Colitis inducing agent 2,4,6-trinitrobenzene sulfonic acid was purchased from Sigma-Aldrich, USA.
2.2. Methods
2.2.1. Synthesis of MAA–BMA copolymers
Copolymers of methacrylic acid and butyl methacrylate having different molar feed ratios (3:7, 4:6, 5:5, 6:4, and 7:3) were synthesized in glass tubes sealed with Teflon® film, using THF as solvent and AIBN (0.25% w/v) as free radical initiator as shown in Figure . Appropriate quantities of the monomer, comonomer, THF, and AIBN were taken in a polymerization tube and mixed vigorously. Then, the reaction mixture was flushed with nitrogen gas for 10 min, sealed under nitrogen atmosphere, and immersed in a thermostatic water bath at 65 ± 2 °C. After 16 h, the excess solvent was removed from the polymer under vacuum. Then, the polymer was dissolved in minimum amount of methanol and DCM mixture (5:2) and precipitated in ice-cold petroleum ether. The precipitated polymers were dried under vacuum. Subsequently, the polymers were boiled in distilled water for 5–10 min in order to get rid of unreacted monomers. Finally, the polymers were dried to constant weight.
2.2.2. Copolymer characterization
The selected copolymers were subjected to Fourier transform infrared spectroscopy in the range of 4000–400 cm−1 as KBr pellets. The FTIR spectra of the monomers were taken as neat film (Perkin-Elmer, USA). The chemical structure of the selected copolymers was characterized with 1H NMR using CDCl3 as solvent (Jeol, 400 MHz, Japan). Powder XRD of the selected copolymers was carried out in the range of 5–50° 2θ (X’Pert PRO, PANalytical, Netherlands). Differential scanning calorimetry of the selected copolymers was carried out at a heating rate of 10°/min in the temperature range of 30–200 °C in nitrogen atmosphere (DSC-60 Shimadzu, Japan). An empty aluminum pan was used as reference. Molecular weight of selected copolymers was determined by gel permeation chromatography relative to polystyrene standards using THF as mobile phase (Turbo Matrix-40, Perkin-Elmer, USA).
2.2.3. Estimation of apparent monomer composition in copolymers
The methacrylic acid content of the selected copolymers was analyzed as per the method described for methacrylic acid polymers in the USP27/NF22. Briefly, the dried copolymer was dissolved in 50 ml of acetone–methanol mixture (1:1) and titrated against 0.1 N NaOH using phenolphthalein as an indicator. The butyl methacrylate composition was estimated from the difference between weight of copolymer and the weight of MAA.
2.2.4. Swelling behavior
To demonstrate the swelling and erosion behavior, membranes of all the synthesized copolymers were prepared. A fixed weight of the copolymer was dissolved in 5 ml of methanol and the solution was spread uniformly onto a Teflon® mold. Solution was kept at room temperature for 4 h and then dried overnight. After drying, the membrane was kept in a desiccator under vacuum.
Membrane swelling was measured in four different buffers: 0.1 M HCl (pH 1.2), 0.1 M mixed phosphate buffer (pH 5.5), 0.2 M phosphate buffer (pH 6.8 and pH 7.4) prepared as per Indian Pharmacopoeia 1996. The dry polymer membrane of fixed weight was placed in 10 ml of buffer. The buffer was replaced with a buffer of higher pH (starting with pH 1.2 and then 5.5, 6.8, and 7.4) every 2 h. After fixed intervals, the membrane was removed from buffer and wiped dry and reweighed. The percentage weight gain or percentage swelling was calculated using Equation (Equation1(1) ).
(1)
2.2.5. Erosion behavior
The membrane erosion was measured in the same buffers as used in the swelling study. The membrane was dried to constant weight at 100 °C, weighed, and placed in 10 ml of buffer. The buffer was replaced with a buffer of higher pH (starting with pH 1.2 and then 5.5, 6.8, and 7.4) every 2 h. After fixed intervals, the membrane was removed from buffer, dried to constant weight at 100 °C, and reweighed. The same dried membrane was then put into higher pH buffer. The percentage erosion (weight loss) was calculated using Equation (Equation2(2) ).
(2)
W1 stands for initial weight of the copolymer membrane and W2 stands for the weight of the copolymer membrane after buffer treatment.
2.2.6. Hemolysis assay
The hemolytic effect of the polymers on acid citrate dextrose (ACD) blood was studied according to a previously reported method.[Citation31] ACD blood was prepared by adding 1 ml of ACD solution to 9 ml of fresh blood. Blood testing solution was prepared by diluting 4 ml of fresh ACD blood with 5 ml of saline. The membrane of the selected copolymers MAA:BMA (3:7 and 4:6) was equilibrated in 4 ml saline for 30 min at 37 ± 1 °C. Diluted blood (0.2 ml) was added to each sample and incubated for 60 min at 37 ± 1 °C. Positive and negative controls were also similarly incubated in similar conditions. All solutions were centrifuged for 5 min and the optical density of the supernatant was measured at 545 nm. The percentage hemolysis was calculated using Equation (Equation3(3) ).
(3)
2.2.7. Preparation of microparticles
Aceclofenac was encapsulated in the copolymers MAA:BMA (3:7 and 4:6) by oil-in-oil solvent evaporation technique.[Citation30] Briefly, drug and polymer (1:4 w/w) were dissolved in a mixture of methanol and DCM (2:3 v/v). The resulting solution was added dropwise into liquid paraffin containing Span 80 (0.5% v/v) as emulsifier, under constant stirring at 1300 rpm. The system was kept at constant stirring at room temperature till all the solvent evaporated. The microparticles thus obtained were separated by filtration, washed three times with n-hexane, and dried under vacuum. The microparticles prepared with MAA:BMA (3:7 and 4:6) were designated as M3 and M4, respectively.
2.2.8. Microparticles characterization
Microparticles were characterized for shape by scanning electron microscopy (EVO 40 Zeiss, Germany) and for zeta potential (Nano ZS-90Malvern Instruments, UK). FTIR spectroscopy, X-ray diffraction, and differential scanning calorimetry of the microparticles were done using the same instruments and methods described under copolymer characterization.
2.2.9. Determination of drug loading
A weighed amount of microparticles was crushed and dissolved in 10 ml mixture of phosphate buffer (pH 8.0) and methanol (1:1 v/v). The drug content was estimated by spectrophotometric analysis at 274 nm using UV–visible spectrophotometer. The percentage drug loading and percentage drug loading efficiency were calculated using Equations (Equation4(4) ) and (Equation5
(5) ), respectively.
(4)
(5)
2.2.10. Mucoadhesion testing
Mucoadhesive property of prepared microparticles was evaluated by an in vitro wash-off method.[Citation32] Freshly excised piece of stomach or intestine of goat was cut to expose the mucosal surface and washed with normal saline. The serosal side of the excised stomach or intestine was mounted on a glass slide with cyanoacrylate glue. The microparticles were fixed on the mucosa by gentle pressure and kept for few seconds. Thereafter, the glass slide was hung on the arm of tablet disintegration apparatus (Scientech Instruments, India) with the help of a clamp and thread. The disintegration apparatus was operated to give a regular up and down movement to the tissue in the test fluid (0.1 N HCl for stomach and phosphate buffer of pH 6.8 for intestine) at 37 ± 2 °C. The time required for all the microparticles to get detached from the tissue mucosal surface was considered as mucoadhesion time.
2.2.11. In vitro drug release
The in vitro release of aceclofenac from microparticles was evaluated in a USP type II apparatus (Lab India DS800, India) using 500 ml release medium, at 50 rpm and 37 ± 2 °C. To simulate gastrointestinal pH conditions, the drug release was evaluated in different buffers of pH 1.2, 5.5, 6.8, and 7.4. After every 2 h, the release medium was filtered and the microparticles were replaced in dissolution flask containing buffer of higher pH. The buffers used were same as described under swelling study. Samples were withdrawn at predetermined intervals, filtered, and assayed spectrophotometrically at 274 nm. After each sampling, the volume of the dissolution medium was replenished with fresh buffer in order to maintain the sink condition.
2.2.12. In vivo study
The in vivo studies were approved by the Delhi Institute of Pharmaceutical Sciences and Research animal ethics committee. To study the treatment effects of formulation M3, Wistar rats weighing 225–250 g were divided into different groups (5 animals per group): group I, healthy animals; group II, induced colitis group; group III, formulation treatment group; and group IV, standard treatment group. Animals were kept in a standard environment of 25 ± 2 °C temperature, 40% RH, 12-h light: dark cycle and had free access to the laboratory chow and purified water.
2.2.13. Induction of colitis and treatment
Induction of colitis was done according to a previously reported method by Morris et al. [Citation33]. Briefly, animals were fasted for 24 h, and under light anesthesia, trinitrobenzene sulfonic acid (TNBS) (150 mg/kg) in 50% ethanol was administered intrarectally to the rats through a rubber catheter, inserted 8 cm in the rectum. Rats were held in upside down position for 2 min to avoid any leakage. The induction of colitis was studied over a period of 9 days by assessing the clinical activity scores and colon-to-body weight ratio. The control group (healthy animals) received an equal volume of 50% ethanol. In treatment studies, animals were monitored for 3 days without any treatment, for the development of colitis. Formulation treatment group received the microparticle M3 (equivalent to 5.1 mg/kg of aceclofenac), and standard treatment group received prednisolone (2 mg/kg), daily for 4 days starting from day 3 of the TNBS administration. After 24 h of the last dosing, animals were sacrificed.
2.2.14. Clinical activity scores
The development of colitis was assessed by giving scores to animals for clinical activity based on weight loss, stool consistency, and appearance of blood in stool. The scoring pattern used for weight loss was as follows: no weight loss, 0; 1–5% weight loss, 1; 5–10% weight loss, 2; 10–20% weight loss, 3; and ≥20% weight loss, 4. Scoring pattern for stool consistency was as follows: well-formed pellets, 0; pasty and semisolid stool not sticking to the anus, 2; and liquid stool sticking to the anus, 4. In case of rectal bleeding, the scoring was done in the following pattern: no bleeding, 0; positive finding, 2; and gross bleeding, 4. Average of the above-mentioned scores were calculated for determining the final clinical activity score.
2.2.15. Colon-to-body weight ratio
After sacrificing the animal, the abdomen was opened and the colon was quickly excised. After opening the colon longitudinally, it was washed with normal saline on a chilled glass plate placed over ice. The distal 8 cm section of the colon was taken and weighed. The ratio of wet colon weight to the total body weight was calculated.
2.2.16. Myeloperoxidase estimation
Myeloperoxidase estimation was done according to a previously reported method.[Citation34] Briefly, colon specimens were minced in a beaker containing a fixed volume of iced water for injection, followed by homogenization (three times for 30 s). HTAB buffer (0.5 ml) was mixed with 0.5 ml of tissue homogenate and sonicated for 10 s, followed by centrifugation at 10,000 rpm for 15 min. The supernatant (0.1 ml) was added to 2.9 ml solution containing 0.167 mg/ml o-dianisidine dihydrochloride and 0.0005% hydrogen peroxide. The change in absorbance at 460 nm was observed for 4 min and MPO activity was determined as U/g of wet tissue. One unit of MPO activity was defined as the amount which degraded 1 μmol of hydrogen peroxide per minute at 25 °C.
2.2.17. Histopathological study
The tissue samples were fixed in 10% (v/v) formaldehyde and stained with hematoxylin and eosin. Inflammatory changes like cell infiltration and edema were analyzed using light microscopy.
2.3. Statistical analysis
Data of in vivo activity was tested by one-way ANOVA followed by Dunnett’s test.
3. Results and discussion
3.1. Swelling behavior of copolymers
Swelling behavior of the dried membranes of all the synthesized copolymers is shown in Figure . The copolymers MAA:BMA (7:3 and 6:4) showed an increased weight gain from pH 1.2 (2 h) to pH 5.5 (2 h). The copolymer MAA:BMA (7:3) was dissolved at pH 6.8 in the first hour while copolymer MAA:BMA (6:4) showed further increased weight gain at pH 6.8. However, it also dissolved at pH 7.4 in the first hour of exposure. Similarly, the copolymer MAA:BMA (5:5) showed weight gain of 10.4, 19.4, 26.7, and 42.5% at pH 1.2, 5.5, 6.8, and 7.4 (2 h in each), respectively. But these copolymers showed less weight gain at these pH values as compared to MAA:BMA (7:3 and 6:4). The copolymers MAA:BMA (4:6 and 3:7) also showed continuous weight gain between pH 1.2 and 7.4. The copolymer MAA:BMA (4:6) showed a weight gain of 4.4% at pH 1.2 after 2 h which reached up to a maximum of 19.5% after 2 h at pH 7.4. The least weight gain at all pH values among all the synthesized copolymers was observed for the copolymer MAA:BMA (3:7), which showed 3.1, 8, 11.8, and 17.2% weight gain at pH 1.2, 5.5, 6.8, and 7.4 (2 h in each), respectively. Thus, it was observed that as the methacrylic acid content in the copolymers decreased, the percentage swelling or percentage weight gain at a particular pH value also decreased. Also, the percentage weight gain of a particular copolymer increased with increase in the pH of the buffer.
3.2. Erosion behavior
The study was conducted to ascertain whether the swelling of the membrane was accompanied with erosion (Figure ). The results of the erosion study revealed that erosion of the membranes of all the copolymers occurred at all the pH values. The erosion increased with an increase in the pH value, which means the highest erosion took place at pH 7.4. Also, a correlation between the MAA content in copolymer and percentage erosion was noticed. The percent erosion decreased with a decrease in MAA content. The copolymer MAA:BMA (7:3, 6:4 and 5:5) showed 2.3, 1.8, and 1.7% erosion at pH 1.2 in 2 h. Therefore, it appears that these copolymers would start releasing the drug in the stomach or small intestine. However, copolymers MAA:BMA (3:7 and 4:6) showed very less dissolution at pH 1.2 in 2 h (1.1 and 1.3%, respectively). Corresponding erosion values for these copolymers at pH 5.5 were also less than copolymers MAA:BMA (5:5, 6:4, and 7:3). Therefore, these copolymers, as encapsulating polymers, would protect the drug in the stomach and upper parts of small intestine. The erosion of copolymers MAA:BMA (3:7 and 4:6) was 20.4 and 23.4%, respectively, at pH 6.8, and 27.1 and 32.5%, respectively, at pH 7.4. This suggests that these copolymers would release the drug in distal ileum and/or colon.
3.3. Characterization of copolymers by FTIR
The FTIR spectra of the selected copolymers MAA:BMA (3:7 and 4:6) and the monomers (MAA and BMA) are shown in Figure . The FTIR spectrum of methacrylic acid showed a broad absorption band at 3350–2800 cm−1 which indicated the presence of hydroxyl group of acid, which was also present in the copolymers. The broad absorption band in the copolymers at 1738 cm−1 (MAA:BMA 3:7) and 1732 cm−1 (MAA:BMA 3:7) was due to C=O stretching of the ester group, and weak band at 1475 cm−1 (approx.) is due to the symmetric absorption of carboxylate anion. The C=C stretching at 1636 and 1640 cm−1, and out of plane =C–H bending at 940 and 948 cm−1 observed in the spectra of methacrylic acid and butyl methacrylate were absent in the FTIR spectra of copolymers, suggesting successful polymerization.
3.4. Characterization of copolymers by 1H NMR
The 1H NMR spectra of the monomers methacrylic acid and butyl methacrylate and copolymers MAA:BMA (3:7 and 4:6) are shown in Figure . The spectra of monomers showed the presence of vinyl protons at 5.67 and 6.24 ppm in methacrylic acid and at 5.5 and 6.05 in butyl methacrylate. The absence of these peaks in both the copolymers revealed that all the double bonds present in the monomers have been converted to aliphatic back bone of polymers, indicating successful polymerization.
3.5. Molecular weight determination by GPC
The number, weight, and viscosity-average molecular weights of the selected copolymers MAA:BMA (3:7 and 4:6) were determined by the gel permeation chromatography, and the results are given in Table . Generally, molecular weight of the polymer affects the swelling and degradation of the polymer matrix. Matrix swelling increases with increase in the molecular weight until a limiting threshold level is reached.[Citation35] Compared with MAA:BMA (3:7), MAA:BMA (4:6) had higher molecular weight and swelling. Thus, our results are consistent with the published results.
Table 1. Average molecular weights of copolymers MAA:BMA (3:7 and 4:6).
3.6. Estimation of apparent monomer composition in copolymers
The mole content of methacrylic acid in the copolymers MAA:BMA (3:7 and 4:6) was found to be 1.9 and 3.2, respectively, while that of BMA was 8.1 and 6.8, respectively. Thus, the copolymers became richer in BMA with increase in its amount in the monomer feed. Similar results have been obtained in copolymerization of acrylic acid with methyl methacrylate, where formation of hydrogen bonds between acrylic acid and THF has been implicated for the low reactivity ratio of acrylic acid, and the same could result in a polymer of low acrylic acid content.[Citation36] A similar phenomenon could possibly explain the low methacrylic acid content in MAA:BMA copolymers.
3.7. Hemolysis assay
In general, the acrylate polymers are approved by FDA for oral administration, and they are not approved for the parenteral administration because they are non-biodegradable.[Citation37] Moreover, methacrylic-co-butyl methacrylate copolymer has been used in a previous study, for oral insulin delivery.[Citation38] Both the copolymers were found to be highly hemocompatible as per the ASTM standard for biomaterials (ASTM F 756, standard practice for assessment of hemolytic properties of materials), as the % hemolysis was less than 5%.[Citation39] This suggests that these copolymers can be used as biomaterials for specific purposes.
3.8. Microparticles characterization
The microparticles were anionic, spherical with a slightly rough surface (Figure ). The zeta potential was −25.9 mV for M3 and −29.9 for M4. The aceclofenac loading of M3 and M4 was 5.33 and 6.26%, respectively, while the corresponding entrapment efficiency was 26.65 and 31.33%, respectively. The mucoadhesion time on the stomach mucosa was 57 s and 1 min 7 s for M3 and M4, respectively, while the mucoadhesion time on the intestinal mucosa was 26 and 30 min, respectively. Thus, it was observed that increase in methacrylic acid content increases mucoadhesion (Table ).
Table 2. Microspheres characterization.
3.9. Characterization of microparticles by FTIR
The FTIR spectrum of aceclofenac showed N–H stretching at 3319 cm−1, C=O stretching at 171 cm−1, aromatic stretching at 1589 and 1578 cm−1, and C–H bending for the substituted aromatic ring at 781 and 749 cm−1. The FTIR spectra of the microparticles, M3 and M4 showed the peaks at 3320, 1718, 1452, and 750 cm−1, which were due to the presence of aceclofenac in the microparticles (figure not shown). Thus, successful entrapment of drug was confirmed.
3.10. Characterization of copolymers and microparticles by P-XRD
The powder XRD pattern of aceclofenac, copolymers MAA:BMA (3:7 and 4:6), and microparticles is shown in Figure . The diffractogram indicated an amorphous structure of copolymers while aceclofenac was crystalline. The XRD pattern of both the microparticle formulations revealed their amorphous nature which was devoid of any crystalline peak of aceclofenac. The reason of the absence of drug peaks could be attributed to the dilution effect of the amorphous polymers. It has been observed in a study that when a drug is mixed with a polymer at 1:4 drug: polymer ratio, we get a dispersion of drug in the polymer, and the resulting dispersion does not show any peak for the crystalline drug in the PXRD analysis.[Citation40] In other words, if the drug content is ≤20%, one would not notice any drug peak in the X-ray diffractograms. In our study, the drug content in the microparticles was 5.33 and 6.26%. Accordingly, there should not be any visible peak of the drug in the X-ray diffractograms, and it appears to be because of the dilution effect by the amorphous polymer.
3.11. Characterization of copolymers and microparticles by DSC
The DSC thermogram showed a sharp melting endotherm of aceclofenac at 156 °C. Thermograms of the copolymers revealed amorphous nature of the copolymers. Copolymer MAA:BMA (3:7) showed two broad endotherms at 61.6 and 110.7 °C, while the copolymer MAA:BMA (4:6) showed broad endotherms at 61.1 and 107.4 °C. The thermograms of the microparticle formulation M3 showed broad endotherms at 59.4 and 178.0 °C, while the corresponding endotherms of M4 were at 72.3 and 190.3 °C (Figure ). The endotherms were devoid of any peak corresponding to the drug which could be possible because of the dilution effect, as the drug contents in the microparticles were very low. Similar results have been observed previously where the melting endotherm of the drug disappeared as the drug content in the formulation decreased.[Citation40]
3.12. In vitro drug release
The prepared microparticles showed very less drug release in 2 h at pH 1.2 (Figure ). The formulation M3 and M4 showed approximately 0.8% release within 2 h at pH 1.2, which could be possible because of the presence of unentrapped drug on the surface of the microparticles. The drug release increases slightly at pH 5.5 for 2 h (7% for M3 and 8.5% for M4). The results suggested that the microparticles on oral administration would not release the drug in the acidic environment of stomach (pH 1.0–3.0) or in the duodenum (pH 5.0). As the pH of the dissolution medium was raised to 6.8, the drug release increased sharply. The formulation M3 released 31% drug within 2 h at pH 6.8, and further increase in pH to 7.4 released about 91% drug within 4 h. The microparticle formulation M4 showed 33% drug release at pH 6.8 in 2 h and a cumulative drug release of 93% at pH 7.4 in 4 h. These results show that the microparticle formulations would release the drug at distal ileum (pH 6.8) and colon (pH higher than 7.4).
Figure 9. In vitro drug release profile of microparticle formulations M3 and M4. Each point represents mean ± SD (n = 3).
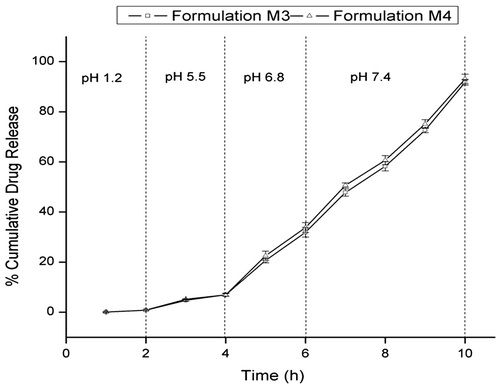
The copolymers MAA:BMA (3:7 and 4:6) contain lesser number of carboxylic groups in the polymer backbone. As a result, the swelling and erosion of the copolymer with increase in pH was less as compared to copolymers MAA:BMA (5:5, 6:4 and 7:3), having higher carboxylic acid content. However, being anionic in nature, the polymers showed pH-dependent swelling and erosion with increase in pH from 1.2 to 7.4. For drug release to occur, the drug will have to diffuse through the polymer. For diffusion to take place, the drug will have to dissolve first in order to give a concentration gradient, as diffusion occurs across a concentration gradient. Aceclofenac, a weakly acidic drug has a pKa of 4.7. Hence, at pH 1.2, the pH is below the pKa of the drug; thus, the drug would be present in the unionized form and will not be dissolved. At pH 5.5, the pH exceeds pKa, and therefore, some drug may be dissolved due to ionization, but the quantity dissolved would be too small to give a sufficient concentration gradient for the diffusion. Increase of pH to 6.8 would promote pH-induced ionization/dissolution of drug, and copolymer would also swell and erode. The dissolved drug would diffuse through the swollen polymer across the concentration gradient. Subsequent increase in pH to 7.4 would promote drug dissolution and polymer swelling/erosion which would facilitate further drug release. This was reflected in the results where almost complete drug load was release at pH 7.4 within 4 h.
Considering the gastrointestinal transit time from mouth to cecum as 4–6 h,[Citation41] it can be stated that the formulations would release more than 60% of the drug load in the colon, in addition to the 35% drug which would be released in the proximity of colon and would also reach the colon in no time. The mucoadhesive nature of the copolymers would also affect the drug release. The microparticles formulation M4 exhibited a mucoadhesion time of 21 s on stomach mucosa indicating that the formulations are not gastroretentive. The mucoadhesion time on intestinal mucosa was 25 min suggesting that the microparticles may be retained in the distal ileum for a short period of time. However, the formulation M3 had a lesser mucoadhesion time of 10 s on stomach mucosa and 15 min on intestinal mucosa.
The drug release kinetics and mechanism was studied by subjecting the in vitro drug release data to different kinetic equations. The results revealed that the best fit was obtained in the zero-order equation which means the drug release from the microparticles was independent of the drug concentration. For both the formulations, the ‘n’ value as per the Korsmeyer–Peppas model was found to be 0.61 and 0.65 (between 0.45 and 0.89). This indicates that the drug release mechanism was anomalous, and swelling, diffusion, and erosion of microparticles were equally responsible for the drug release (Table ).[Citation42,43]
Table 3. Release kinetics of aceclofenac from microparticles using different kinetic equations.
3.13. Induction of colitis
Induction of colitis was studied over a period of 9 days after the administration of TNBS. The successful induction of colitis was confirmed by the significant increase in clinical activity score (Figure (A)) and colon weight-to-body weight ratio (Figure (B)), as compared to the healthy group. The average clinical activity score for colitis group increased gradually from 0.5 ± 0.5 in day 1 to 7.8 ± 0.7 in day 5. The score then started falling gradually from day 5 to day 9. Though there was significant weight loss, and diarrhea, there was no sign of rectal bleeding at any point during the entire study. Similar results were obtained in the colon-to-body weight ratio study. The colon-to-body weight ratio was 0.008511 ± 0.0012 in rats sacrificed on day 3, which increased up to 0.009041 ± 0.0014 in rats sacrificed on day 5. The values then started decreasing. Therefore, day 3 to day 6 was the period of highest inflammation.
Figure 10. Clinical activity scores during induction of colitis (from day 1 to day 9) and colon-to-body weight ratios (day 3, 5, 7 and 9) after TNBS administration. *Statistically significant (p < 0.05) compared with group I (healthy), as determined by one-way ANOVA followed by Dunnett’s test. Each bar represents the mean ± SD (n = 5).
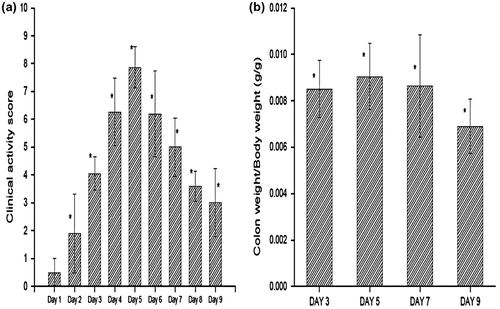
3.14. Clinical activity score
The average clinical activity score for healthy rats was 0.4 ± 0.5 (Figure (A)). The clinical activity score of the microparticles treated group (1.8 ± 1.3) was significantly less as compared to the colitis group (5 ± 1.05). Thus, it was evident that the microparticles were beneficial in the treatment of disease symptoms.
Figure 11. Clinical activity scores, colon-to-body weight ratio, and myeloperoxidase activity of group I (healthy group), group II (colitis group), group III (formulation treatment group), and group IV (standard treatment group). *Statistically significant (p < 0.05) compared with group II (colitis group), as determined by one-way ANOVA followed by Dunnett’s test. Each bar represents the mean ± SD (n = 5).
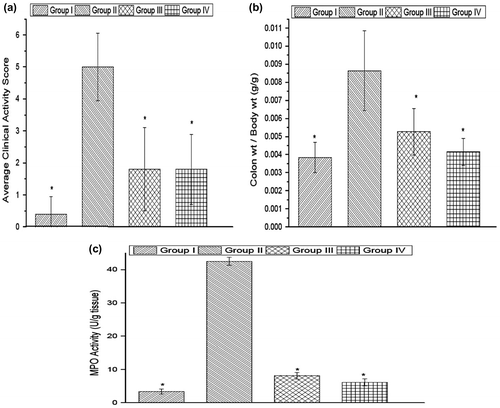
3.15. Colon-to-body weight ratio
The colon weight-to-body weight ratio of normal healthy rats was 0.003849 ± 0.00084 (Figure (B)). Colon-to-body weight ratio is a good indicator of the tissue edema caused due to the inflammation. The microparticles-treated group showed a decreased colon-to-body weight ratio of 0.005262 ± 0.001297 which was significantly less than the corresponding value of colitis group (0.00864 ± 0.002212). The results suggest that the microparticles were successful in lowering the inflammation which resulted in decrease in tissue edema.
3.16. Myeloperoxidase assay
Myeloperoxidase activity is a good indicator of the number of neutrophils infiltrated into the site of inflammation. The myeloperoxidase activity in the colitis group was 42.49 ± 1.19. The corresponding value for the microparticles-treated group was 8.08 ± 0.98, which was significantly lower than the activity present in the colitis group (Figure (C)). The MPO activity in the healthy rats was 3.4 ± 0.7 U/g of wet tissue. This gives a clear indication that microparticle formulation has successfully reduced the inflammation.
3.17. Histopathological analysis
The photomicrograph of healthy rat showed intact mucosa, submucosa, and muscular layers as distinct layers, and the normal colonic histological appearance (Figure ). Rat with untreated colitis showed loss of mucosal layer, with ulceration, edema, and pronounced inflammatory cells infiltration in the submucosal layer. The microparticle-treated rat showed no ulceration or loss of mucosal layer. However, there was a little inflammatory cell infiltration, and the submucosa showed a mild edema. Similarly, the rat treated with standard drug also showed normal submucosal and muscular layers, while a small ulcer was found in the mucosal layer. Therefore, it can be said that the microparticles and the standard treatment caused reduction in the inflammation caused by TNBS administration.
4. Conclusion
The pH-sensitive copolymers with different methacrylic acid and butyl methacrylate molar ratio were successfully synthesized and characterized. The selected copolymers were used for the preparation of colon-targeted microparticle formulations. As these formulations release major portion of the drug at pH ≥ 6.8, these would act as enteric formulation and target the drug to the distal ileum or colon. The in vivo studies were also in accordance with the in vitro release studies, and the microparticle formulation showed significant improvements in the TNBS-induced colitis in rats. These formulations would be helpful in targeting drugs like NSAIDs, glucocorticoids, and anti-cancer drugs directly to the colon, and thereby decreasing the dose required for the treatment.
Acknowledgement
The authors are thankful to the University Grant Commission (UGC), India, for providing fellowship to Mr Amit Arya during the period of research work.
Disclosure statement
The authors report no declaration of interest.
References
- Langholz E. Current trends in inflammatory bowel disease: the natural history. Ther. Adv. Gastroenterol. 2010;3: 77–86.
- Baumgart DC, Sandborn WJ. Inflammatory bowel disease: clinical aspects and established and evolving therapies. Lancet. 2007;369:1641–1657.10.1016/S0140-6736(07)60751-X
- Triantafillidis JK, Nasioulas G, Kosmidis PA. Colorectal cancer and inflammatory bowel disease: epidemiology, risk factors, mechanisms of carcinogenesis and prevention strategies. Anticancer Res. 2009;29:2727–2737.
- Latella G, Papi C. Crucial steps in the natural history of inflammatory bowel disease. World J. Gastroenterol. 2012;18:3790–3799.10.3748/wjg.v18.i29.3790
- Munkholm RL Review article: the incidence and prevalence of colorectal cancer in inflammatory bowel disease. Aliment Pharmacol. Ther. 18( Suppl. 2):1–5.
- Molodecky NA, Soon IS, Rabi DM, et al. Increasing incidence and prevalence of the inflammatory bowel diseases with time, based on systematic review. Gastroenterology. 2012;142:46–54.10.1053/j.gastro.2011.10.001
- Soon IS, Molodecky NA, Rabi DM, et al. The relationship between urban environment and the inflammatory bowel diseases: a systematic review and meta-analysis. BMC Gastroenterol. 2012;12:51.10.1186/1471-230X-12-51
- Li Z, de Zhang K, Yi WQ, et al. NF-κB p65 antisense oligonucleotides may serve as a novel molecular approach for the treatment of patients with ulcerative colitis. Arch. Med. Res. 2008;39:729–734.10.1016/j.arcmed.2008.08.001
- Oslen T, Cui G, Goll R, et al. Infliximab therapy decreases the levels of TNF-alpha and IFN-gamma mRNA in colonic mucosa of ulcerative colitis. Scand. J. Gastroenterol. 2009;44:727–735.
- Carter MJ, Lobo AJ, Travis SPL. Guidelines for the management of inflammatory bowel disease in adults. Gut. 2004;53:1–6.10.1136/gut.2004.043372
- Sandborn WJ. Oral 5-ASA therapy in Ulcerative colitis: what are the implications of the new formulations? J. Clin. Gastroenterol. 2008;42:338–344.10.1097/MCG.0b013e3181595b56
- Sobczak M, Fabisiak A, Murawska N, et al. Current overview of extrinsic and intrinsic factors in etiology and progression of inflammatory bowel diseases. Pharmacol. Rep. 2014;66:766–775.10.1016/j.pharep.2014.04.005
- Jain NK. Advances in controlled and novel drug delivery. 1st ed. New Delhi: CBS Publishers and Distributors; 2001. p. 89–119.
- Jain SK, Jain A. Target-specific drug release to the colon. Expert Opin. Drug Delivery. 2008;5:483–498.10.1517/edd.2008.5.issue-5
- Nemec JW, Bauer W. Acrylic and methacrylic acid polymers. In: Mark HF, Kroschwitz JL, editors. Encyclopedia of polymer science and engineering. 2nd ed. New York (NY): Wiley; 1985.p. 211–234.
- Jones D. Pharmaceutical applications of polymers for drug delivery. In: Rapra review reports. Vol. 15. UK: Rapra Technology Ltd; 2004. p. 3–4.
- Thakral SK, Thakral NK, Majumdar DK. Eudragit®: a technology evaluation. Expert Opin. Drug Delivery. 2013;10:131–149.10.1517/17425247.2013.736962
- Muñoz-Muñoz F, Ruiz JC, Alvarez-Lorenzo C, et al. Novel interpenetrating smart polymer networks grafted onto polypropylene by gamma radiation for loading and delivery of vancomycin. Eur. Polym. J. 2009;45:1859–1867.10.1016/j.eurpolymj.2009.04.023
- Loughlin RG, Tunney MM, Donnelly RF, et al. Modulation of gel formation and drug-release characteristics of lidocaine-loaded poly(vinyl alcohol)-tetraborate hydrogel systems using scavenger polyol sugars. Eur. J. Pharm. Biopharm. 2008;69:1135–1146.10.1016/j.ejpb.2008.01.033
- Vijay S, Sati OP, Majumdar DK. Acrylic acid–methyl methacrylate copolymer for oral prolonged drug release. J. Mater. Sci.: Mater. Med. 2010;21:2583–2592.
- Vijay S, Sati OP, Majumdar DK. Acrylic acid–methyl methacrylate (2.5:7.5/2:8) enteric copolymer for targeted drug delivery. J. Mater. Sci.: Mater. Med. 2010; 22:125–135.
- Kanevskaya YA, Shchepilov AM, Zubov PI. The effect of the supermolecular structure of coatings of a copolymer of butyl methacrylate and methacrylic acid on durability. Polym. Sci. USSR. 1970;12:2201–2206.10.1016/0032-3950(70)90449-1
- Konstantinopolskaya MB, Kanevskaya YA, Karyakina MI, et al. The structure of a copolymer of butyl methacrylate and methacrylic acid. Polym. Sci. USSR. 1965:1102–1105.10.1016/0032-3950(65)90386-2
- Qui X, Song G, Chu X, et al. microencapsulated n-alkane with p (n-butyl methacrylate-co-methacrylic acid) shell as phase change materials for the thermal energy storage. Solar Energy. 2013;91:212–220.
- Bezuglyi VD, Voskresenskaya IB, Alekseyeva TA, et al. Investigation of the copolymerization of methacrylic acid with methyl acrylate and butyl methacrylate in water–dioxan solvent systems. Polym. Sci. USSR. 1975;17:112–116.10.1016/0032-3950(75)90222-1
- Scherrer RA, Whitehouse MW. Anti-inflammatory agents. New York (NY): Academic Press; 1974. p. 33.
- Neil MJ, editor. The Merck index: an encyclopedia of chemicals, drugs and biological. 14th ed. Whitehouse station (NJ). Merck Research Laboratories Division of Merck & Co.; 2006. p. 582.
- Dooley M, Spencer CM, Dunn CJ. Aceclofenac: a reappraisal of its use in the management of pain and rheumatic disease. Drugs. 2001;61:1351–1378.10.2165/00003495-200161090-00012
- Meyer JH, Dressman J, Fink AS, et al. Effect of size and density on gastric emptying of indigestible solids. Gastroenterology. 1985;89:805–813.
- Rodrı́guez M, Vila-Jato JL, Torres D. Design of a new multiparticulate system for potential site specific and controlled drug delivery to the colonic region. J. Controlled Release. 1998;55:67–77.10.1016/S0168-3659(98)00029-7
- Xiang-HuaQu, QWu, GQChen. In vitro study on hemocompatibility and cytocompatibility of poly(3-hydroxybutyrate-co-3-hydroxyhexanoate). J. Biomater. Sci. Polym. Ed. 2006; 17:1107–1121.10.1163/156856206778530704
- Chowdhary KPR, Srinivasa RY. Design and in vitro and in vivo evaluation of mucoadhesive microcapsule of glipizide for oral controlled release: a technical note. AAPS Pharm. Sci. Tech. 2003; 4:320–325.
- Morris GD, Beck PL, Herridg MS, et al. Hapten-induced model of chronic inflammation and ulceration in the rat colon. Gastroenterology. 1989;96:795–803.
- Krawisz JE, Sharon P, Stenson WF. Quantitative assay for acute intestinal inflammation based on myeloperoxidase activity. Assessment of inflammation in the rat and hamster model. Gastroenterology. 1984;87:1344–1350.
- Ju RTC, Nixon PR, Patel MV. Drug release from hydrophilic matrices. 1. New scaling laws for predicting polymer and drug release based on the polymer disentanglement concentration and the diffusion layer. J. Pharm. Sci. 1995;84:1455–1463.10.1002/(ISSN)1520-6017
- Kerbers R, Glamann H. Lösungsmitteleinflüsse auf die copolymerisations parameter des systems methylmethacrylat/acrylsäure [Solvent effects on the reactivity ratios of the system methyl methacrylate / acrylic acid]. Die Makromolekulare Chemie, German. 1967;100:290.10.1002/macp.1967.021000134
- Thakral S, Thakral NK, Majumdar DK. Eudragit®: a technology evaluation. Expert Opin. Drug Delivery. 2013;10:131–149.10.1517/17425247.2013.736962
- Ito Y, Casolaro M, Kono K, et al. An insulin-releasing system that is responsive to glucose. J. Controlled Release. 1989;10:195–203.10.1016/0168-3659(89)90063-1
- Autian J Biological model systems for the testing of the toxicity of biomaterials. Polymers in medicine and surgery. In: Kronethal RL, Oser Z, Martin E, editors. Polymer science and technology. New York (NY): Plenum Press; 1993. p. 181.
- Maghsoodi M. Physicomechanical properties of naproxen-loaded microparticles prepared from Eudragit L100. AAPS PharmSciTech. 2009;10:120–128.10.1208/s12249-009-9186-5
- Shargel L, Yu A Applied biopharmaceutics & pharmacokinetics. London, UK: Printice-Hall International Inc.; 1999. p. 173.
- Peppas NA. Analysis of fickian and non-fickian drug release from polymers. Pharm Acta Helv. 1985;60:110–111.
- Chueh HR, Zia H, Rhodes CP. Optimization of sotalol floating and bioadhesive extended release tablet formulations. Drug Dev. Ind. Pharm. 1995;21:1725–1747.10.3109/03639049509069261