Abstract
The European ELOISE (European Land Ocean Interaction Studies) cluster (>60 projects) is the world's largest research initiative on land – ocean interactions. Beside its scientific goals, ELOISE is also meant to contribute directly to coastal zone management and thus to European coastal policy. Most of the ELOISE results have been gathered in digests that are made available on the Internet to all potential end-users (see http://www.eloisegroup.org/themes/).
This paper highlights the contribution of the scientific work within ELOISE to the understanding of the ecological functioning of coastal ecosystems under eutrophication stress and what the implications are for the management of these systems.
Our main objectives were (i) to identify the cross-cutting concepts emerging from the scientific studies, (ii) to point out how these concepts changed our view of the intrinsic characteristics of ecosystems and of their responses to man-made disturbance, and (iii) to relate them to issues in ecosystem management. One important conclusion is the crucial issue of spatial and temporal scale for most of the processes controlling the fluxes and impacts of nutrients in water systems. Beside the availability of the appropriate models and databases, necessary tools to bridge the gaps between scales, the main challenge for water systems managers is how to organize a system where appropriate and coordinated actions can be taken at all scales, from local communities to the European level. This study points out some tremendous advances in our understanding of essential processes in nutrient cycling that are triggered by progresses in fundamental scientific knowledge. It follows that continuing efforts at deepening our insight into ecosystem functioning are required to support a sound management for coastal ecosystems.
1. Introduction
Since the premonitory ‘Avant que nature meure’ by Jean Dorst (Citation1965) the realization that human activities are altering the functioning of the Earth system has become an incontestable fact (Vitousek Citation1994; Vitousek et al. Citation1997). Cloern (Citation2001) stressed that one of the prominent modes of human disturbance has resulted from activities that mobilize the nutrient elements nitrogen and phosphorus through land clearing, production and applications of fertilizer, discharge of human waste, animal production, and combustion of fossil fuels. These activities have resulted in elevated nitrogen and phosphorus concentrations on land and in the acceleration of the fluxes of these elements to coastal waters, disrupting the balance between the production and metabolism of organic matter in the coastal zone (Cloern Citation2001). There is a large and continuously increasing amount of publications dealing with these processes (Vidal et al. Citation1999) and we have now a rather well defined view on the effects of eutrophication on coastal ecosystems (Elliott & de Jonge Citation2002). The term ‘eutrophication’ is generally used to describe the process of enrichment itself and of its effects on the environment. This issue is addressed in several EU policies and an ongoing effort aims at a pan-European definition for the concept of eutrophication. The following definition was proposed during the 2004 meeting of the Monitoring and Assessment Group from the Helsinki Commission in Tallinn:
‘Eutrophication means the enrichment of water by nutrients, especially compounds of nitrogen and/or phosphorus, causing an accelerated growth of algae and higher forms of plant life to produce an undesirable disturbance to the balance of organisms present in the water and to the quality of the water concerned, and therefore refers to the undesirable effects resulting from anthropogenic enrichment by nutrients’.
Cloern (Citation2001) describes how conceptual models for eutrophication effects have evolved over time. The first conceptual model that was originally (1960s – 1970s) developed by limnologists described unequivocal linkages between simple bulk quantities such as nutrient loading and chlorophyll standing stock (Vollenweider Citation1976; Schindler Citation1977). During the 1980s and 1990s it became obvious that fundamental differences exist in the system-level responses to nutrient enrichment in estuarine – coastal ecosystems compared to lakes (Oviatt et al. Citation1995; Borum Citation1996). This shift in the perception of the eutrophication processes in coastal areas served as a foundation for our contemporary conceptual model. This view supports that, among other factors, physical constraints, nutrient ratio, sediment buffers and food-web structure are actively shaping the responses of ecosystem to both nutrient enrichments and associated sanitation measures.
Despite the tremendous improvements in our representation of the ecosystem processes, we acknowledge, with Cloern (Citation2001), that the question of how anthropogenic nutrient enrichment causes change in coastal ecosystems still exceeds the boundaries of our contemporary conceptual understanding. Further progress in research and a sustained effort of dissemination of knowledge to society are challenging us since the effectiveness of strategies for ecosystem protection or restoration will be directly proportional to the accuracy and completeness of our representation of the ecosystem responses to anthropogenic stressors.
The aim of this paper is to describe how scientific work within the European ELOISE (European Land Ocean Interaction Studies) cluster of projects has contributed to a better understanding of the ecological functioning of coastal ecosystems under eutrophication stress and to the development of quantitative tools. We make no attempt at completeness, but use examples to illustrate some of our conclusions. Operating since 1996, with more than 60 projects, the European ELOISE initiative has now entered a phase where scientific results are being disseminated to all potential end-users. With respect to nutrient-related problems, a synthesis article (Herman et al. Citation2005) and web-published digest and presentation (Escaravage et al. Citation2004) were elaborated to disseminate the major findings of these projects to a broader audience.
As a continuation of the task of dissemination after the completion of the digest, we attempt here to summarize the ELOISE projects at a higher level of integration. Our objective is to highlight common concepts that were described and worked out within the different projects, to point out how these concepts changed our view of the intrinsic characteristics of ecosystems and of their responses to man-made disturbance, and to relate them to concepts of ecosystem management. For the latter purpose, we relate the cross-cutting concepts emerging from the scientific studies to the DPSIR (Driving forces, Pressures, States, Impacts, Responses) framework. DPSIR is the management approach supported by the European Environment Agency for defining the appropriate measures to mitigate environmental issues. It consists of the identification of causal relationships between the Driving forces, Pressures, States of the environment, Impacts on society and the adequate Responses for mitigation.
Results from 27 ELOISE projects were used in the present study ( ). The projects were selected according to their contribution to the selected topics and only peer-reviewed published results were used as a guarantee of their scientific quality. Some projects were still delivering their main products at the time of writing, and may be under-represented in the overview.
Table I. List of ELOISE projects (C for completed projects) and their matching with the three topics selected in the digest by Escaravage et al. (Citation2004): 1, nutrient pathways from source to target; 2, nutrient processing in estuaries and coastal areas; 3, effects on estuarine food webs functioning
2 Common concepts to the investigated ELOISE issues
Four fields of research were mainly addressed in the ELOISE digest (Escaravage et al. Citation2004): atmospheric deposition; rivers and groundwater inputs; nutrient transformations; and food-web impacts. Four concepts were found to be common to these four fields of research from a subsequent study carried out on the digest:
-
nutrient pathways and effects are scale-dependent in space and time;
-
nutrient transformations and effects exhibit non-linear dynamics;
-
present knowledge on nutrient transformations is not yet sufficient; and
-
integrated models are needed to combine all aspects above.
Table II. Features observed within the different fields of research (atmospheric deposition, rivers and groundwater inputs, nutrient transformations, food-web impacts) listed against the corresponding common concepts (in rows) that have been identified from the digest on nutrient dynamics
3 Integration of ELOISE-derived concepts to DPSIR trajectories
As an attempt to integrate the ELOISE results to a DPSIR analytical approach, we propose tracking the journey of the nutrients between their land-based sources to their potential targets located in the coastal area. Our aim is to identify the consequences of the common concepts evidenced from the ELOISE data ( ) on the causal links between the stressors and their effects. For the present exercise, the terms of the DPSIR analytical approach are defined as follows:
The Driving forces are human activities leading to increased nutrient emissions either to the atmosphere or to the watershed. We will not discuss these activities here. The environmental Pressures are the nutrient emissions to the coastal area. The ecological State of the ecosystem is represented by the variables that define the structure and functioning of the coastal ecosystem. We distinguish increased nutrient concentrations, and ecological variables (biomass, production, grazing) that are changed following the increase of emissions. It is clear, however, that both are linked, since the biological system ultimately determines concentrations at least as much as emission does. The environmental Impacts represent the consequences of the changes in ecological state for the functions supported by the coastal ecosystem such as carrying capacity or any other function that could be related to the sustainability of human use. Eventually the policy Responses are the management measures that are taken at one or more levels of the nutrient pathway for the remediation of the ecological impacts due to the excessive input of nutrient to the coastal system ( ).
Figure 1. The framework DPSIR for integrated assessment of the effects of the response of policy and measures on the driving forces of environmental pressure and on its changes in the state of the environment and its ecological impact (Oenema Citation2004).
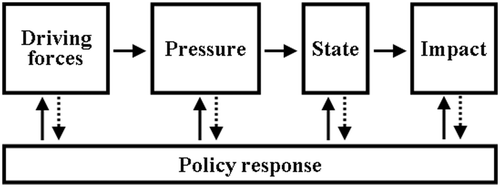
The ELOISE projects have mostly focused on the Pressure – State part of this scheme, with some evaluations of the State – Impact relations, and only a few projects have dealt with either drivers or policy responses.
3.1 Defining the environmental pressures: from emission rates to increased ambient concentrations and/or influxes
The ELOISE results allow identifying a chain of transport/transformation processes that either temper or amplify the relation between the levels of nutrient emission and the levels of nutrient availability in the coastal areas.
3.1.1 A very broad range of spatio-temporal scales are relevant for the nutrient pathways
One of the problems encountered in managing nutrient inputs into the coastal zone is the mix of spatial and temporal scales at which important processes occur. Typical scales for (mostly bacterial) gross turnover of inorganic nitrogen in estuaries may be as short as 1 hour (Middelburg & Nieuwenhuize 2001). However, many of these turnover processes occur in fast and (almost) closed loops. Therefore this timescale is not representative for the timescale of processes relevant for input – output dynamics, such as the net removal of nitrogen from the system. In estuaries the timescale of net nitrogen removal is in the order of months (approximately 30% net removal per month, Dettmann Citation2001). Atmospheric nutrient emissions that are generated from land-based sources at scales of hundreds of kilometres are deposited by precipitation events occurring on the timescale of hours and spatial scales of kilometres (Hertel et al. Citation2002; de Leeuw et al. Citation2003). The input of nutrients to the coastal zone by rivers and estuaries is a function of nutrient transfer from soils and groundwater to surface water in the total watershed. Within the watershed, small-scale processes, e.g. nutrient uptake by aquatic plants in streams (Jarvie et al. Citation2002), have a significant influence on the transfer. Models used to predict the relationship between emissions and nutrient inputs into the coastal zone face the difficulty of bridging these scale gaps. Finding appropriate proxy functions to generalize, at a European scale, the transfer and loss rates in a spatially explicit way without dependence on complete coverage of the surface with data-intensive surveys remains an important challenge (e.g. Forsman et al. Citation2003).
For management of nutrient inputs, this multitude of scales may have implications at the organizational level. There is no single appropriate scale at which solutions can be implemented. Although the watershed approach, which is used, for example, in the Water Framework Directive, has considerable advantages, trans-watershed phenomena such as atmospheric deposition should also receive due attention and, moreover, within the watershed more local units should probably exist to work on solutions at the smaller scale.
3.1.2 The non-linearity of processes complicates the nutrient pathways
The delay of response of river nutrient concentrations after considerable reductions in agricultural nitrogen input as observed for the Elbe and Eastern European rivers results from the long residence time of groundwater reservoirs and from the large amount of organic nitrogen that has accumulated in soils during periods of higher application rates (Grimvall et al. Citation2000). This study demonstrated that nutrient loadings (1) rapidly react to increased input from point and diffuse sources but (2) have a very long lag time after a reduction of the input. As a result, reduction in river nutrient concentrations will not follow source reductions on a short timescale. Although the principle of this delay mechanism can be demonstrated using simple box models with different reaction times (Grimvall et al. Citation2000), applying it in practice to a particular watershed is very difficult due to the often incomplete knowledge of the circulation pathways and dynamics. The detection of the phenomenon has required the large-scale ‘experiment’ of economic change in Eastern Europe, but in order to perform scenarios on watershed where similar breaks in activity have not occurred, fairly detailed groundwater modelling studies (including nutrient and organic carbon reactions in the groundwater) will probably be needed.
For management, this phenomenon is most important at a conceptual level. Simple input – reaction thinking may be very wrong, and this should be taken into consideration when predicting the time frame of changes in the coastal systems as a consequence of management options.
3.1.3 Knowledge on processes regulating the nutrient pathways is not exhaustive
Whereas impressive progress has been realized during the last decade in modelling the atmospheric deposition of nutrients and assessing the emission sources, the processes at the sea – air interface itself remain still not completely understood (de Leeuw et al. Citation2001). For example, little is known about the relative importance of the competing effects of dilution and entrainment, deposition, chemical reactions and re-emission from the sea surface.
When compared with their fresh, stagnant or marine counterparts, nutrient in-stream transformations are rather badly documented. For example, in a recent version of the INCA (Integrated Nitrogen in Catchments) nitrogen model, nutrient in-stream transformations are limited to nitrification and denitrification fluxes that are ad hoc adjusted to match the model output with the measured concentrations (Wade et al. Citation2002). Additional knowledge on this issue is necessary for an exhaustive representation, because streams should be considered as full ecosystems, including their biological compartments.
Continuing efforts in research, both fundamental and applied, is required for adaptive management systems to be established. Even though the implementation of existing knowledge in appropriate tools for management remains a priority, it is illusive to consider that this existing knowledge is sufficient to solve and anticipate all possible problems and solutions.
3.2 From environmental pressures to changes in ecological status
The second step of the DPSIR analytical approach consists of the evaluation of the changes induced in the ecological status of the coastal ecosystem resulting from the changes in nutrient availability and/or concentration.
3.2.1 Spatio-temporal scales for the changes in ecological status
The effects of nutrient input on ecosystem functioning differs according to the regions where it occurs. This is true at a large (European) scale, where several ELOISE projects have documented very different responses between Atlantic and Mediterranean systems, but it is also true at much smaller scales, where physical characteristics of estuaries or lagoons (e.g. strength of stratification, residence time) determine the local vulnerability of the system to nutrient perturbation (Tett et al. Citation2003). Stratification is related to vertical mixing: a stratified water column has denser water near the bottom, and lighter water near the surface. It takes much energy (from tides or wind) to mix the water column. In the absence of vertical mixing, oxygen flux to the lower waters is limited, and it does not take a large organic enrichment to increase oxygen consumption to a level where anoxic conditions occur. Residence time is the average time a water parcel stays in the estuary before it is exchanged with the coastal sea. Estuaries with short residence times are frequently flushed, and any enrichment is easily diluted.
At a European scale, the very different response to atmospheric nitrogen deposition in Mediterranean and Atlantic coastal waters can serve as an example: whereas the Atlantic coastal systems are highly reactive to nitrogen enrichment (de Leeuw et al. Citation2003), in the eastern Mediterranean where abundant cyanobacteria (i.e. photosynthetic bacteria) are actively fixing the atmospheric N2, phosphorus deposition is an important limiting factor for primary production (Migon et al. Citation2001). Nitrogen fixation is the process by which some organisms can transform N2 gas (which is abundantly present in the atmosphere, and also in water) into biologically useful forms such as ammonium and nitrate. Organisms capable of fixing nitrogen have a large advantage in nitrogen-limited waters, but the process is constrained by many biochemical limitations. It occurs frequently in warm seawater, but in colder climates is limited to fresh and brackish waters.
Another example is given by the response of an ecosystem to nutrient inputs. Nutrient additions in experimental systems containing a natural mix of organisms induced biomass explosion of large phytoplankton species (diatoms) when Atlantic waters were fertilized whereas the identical experimental set-up applied to Mediterranean waters induced a very low biomass increase of small phytoplankton species (nanoplankton) (Olsen et al. Citation2001). The differential response that was observed is due to differences in the structure of the food web of Atlantic and Mediterranean systems. The Mediterranean small-sized phytoplankton species are tightly controlled by efficient grazers (microzooplankton ciliates) whereas in the Atlantic, larger-sized (diatom) phytoplankton suffers no severe grazing losses from the relatively slow-growing, relatively large grazers (copepods) that dominate the mesozooplankton.
At a smaller spatial scale, nutrients that are deposited at the sea surface have variable effects on the ecosystem according to the local nutrient status. In well illuminated stratified areas (summer in the North Sea) the surface water layer that is depleted in nutrients reacts to nitrogen deposition events with intense phytoplankton blooms (de Leeuw et al. Citation2003). In such cases, the atmosphere may represent the dominant source of nitrogen to surface waters (Spokes et al. Citation2000; Galloway et al. Citation2003).
At a European scale, the difference in vulnerability (magnitude of the marginal effect of nutrient additions to the systems) between different coastal ecosystems is probably one of the biggest challenges to coastal zone management. Recently, there has been a shift in political emphasis from general norms and standards (e.g. in the nitrogen directive, aiming at maximum concentrations) to result-oriented norms (e.g. in the Water Framework Directive, which requires good ecological status for all waters, thus incorporating differential response of different waters to similar input). Although from an ecological perspective result-oriented approaches are much more meaningful, they create regional and local differences in norms that may be at odds with economic and social policies. Designing flexible management strategies to solve this problem not only requires social research, it also remains an important problem for ecological research. Since responses may be qualitatively different from one system to another, it is not easy to quantify how these qualitatively different responses can be compared on a scale of ‘ecosystem health’ or ecosystem services.
3.2.2 Non-linearity in the relation between pressures and impacts
Critical organic loading levels of sediments have been identified that induce a switch in the sediment chemistry from oxic to anoxic processes of mineralization (Wijsman et al. Citation2002). This transition is predicted to occur abruptly and show hysteresis. Whereas increasing enrichment causes the system to switch from oxic to anoxic state at a certain threshold enrichment, say T i, return from anoxic to oxic state by decreasing the enrichment will only occur after reduction to a different enrichment T r which is well below T i. This phenomenon is well-known from studies in lakes (e.g. Scheffer & Carpenter Citation2003), where it has been the basis for entirely new approaches to biological control. Studies on sediment chemistry in combination with aquatic plants (mostly seagrasses) in the ROBUST project have suggested similar mechanisms for the transition from a seagrass to a macroalgal community: buffer capacities in the sediment can withhold the transition over a certain range of nutrient enrichment, but once beyond this threshold the rapid cycling of nutrients through a macroalgal community can reinforce the eutrophication effects (De Wit et al. Citation2001).
The existence of non-linear behaviour in coastal sediment systems is still speculative, but potentially important in predicting the response of certain systems to enrichment. This is a topic of active research that is, as yet, difficult to implement in scenario studies. Further development of models should be encouraged, since the existence of threshold effects and hysteresis may need incorporation into emission regulation scenarios and may constitute powerful indicators for change in ecosystems.
3.2.3 Gaps in knowledge on nutrient transformations
The fraction of the nutrient input that will be eventually incorporated in the system depends on a complex network of transformations that is far from completely understood. Until recently, denitrification was recognized as the only important process removing nitrogen from the natural environments (denitrification can be considered as the reverse of nitrogen fixation: it transforms nitrate into N2 gas – the process is performed by anoxic bacteria, which use nitrate instead of oxygen to mineralize organic matter). Denitrification is a well-known process that has been extensively studied in coastal systems. However, during the past years, research within ELOISE projects (Welsh et al. Citation2001; Dalsgaard & Thamdrup Citation2002; Thamdrup & Dalsgaard Citation2002) has evidenced two new and unexpected pathways that remove biologically available nitrogen compounds from coastal ecosystems. These pathways, known as ANAMOX (anaerobic ammonium oxidation) and DNRA (Dissimilatory Nitrate Reduction to Ammonium) have now been established as potentially important nitrogen removal mechanisms from natural ecosystems. As they were not measured in previous estimations of nitrogen removal processes, it is clear that additional study is required for further assessment. This illustrates the importance of continued fundamental research, even on relatively well studied problems as the environmental nitrogen cycle.
Gaps in knowledge and development of new insights, even in fundamental processes affecting nutrient transformations in coastal systems, are difficult to take into account in coastal management schemes. Yet the ability to incorporate advancing knowledge may well be one of the key characteristics of adaptive management.
3.3 From changes in ecological status to environmental impacts
The penultimate step in the DPSIR analytical approach deals with the environmental impacts that are induced by the changed ecological status of the ecosystem. This class of impacts concerns the functionality of the coastal system that, in the framework of management issues, mostly addresses the sustainability of human use.
3.3.1 Relevant spatio-temporal scales for the environmental impacts
Natural variability in ecological phenomena may affect human use and, in general, the ‘goods and services’ produced by coastal systems. This natural variability is beyond the influence of management measures, but may interact with anthropogenic influences. As an example we cite the study by Reigstad et al. (Citation2000) on the flux of organic matter to fjord sediments. This flux is important, because it determines how much oxygen is consumed by bacteria in the sediment that mineralize this organic matter. As the water of fjords is badly mixed, the bottom waters become anoxic if too much organic matter is deposited on the sediment. Reigstad et al. (Citation2000) show that the flux to the sediment is controlled by large-scale variations in meteorology, which determine large-scale water currents. In particular, it is important to know if zooplankton organisms (which move with the water masses) flow from shelf waters into adjacent fjords. If there is sufficient zooplankton, the grazing by these organisms on the phytoplankton allows the incorporation of the phytoplankton into the food web and its transfer to fish, and further to birds and sea mammals. Conversely, in the absence of zooplankton grazing, phytoplankton sinks to the bottom and may cause anoxic events associated with mass-mortality events of the benthic fauna.
The amount of phytoplankton that is produced in a coastal water body such as a fjord is determined by nutrient input and anthropogenic stress. Generalizing from this study, it can be stated that the natural conditions leading to a maximal benthic deposition should be taken into account to calculate the maximum oxygen problem possible and its probability of occurrence. Thus, the required nutrient regulation may go well beyond the level needed for ‘good’ years.
The example illustrates two important problems for coastal zone management. First, management that takes into account potential natural variability should aim at targets not ‘normally’ encountered, and therefore easily perceived as too severe. Second, as the occurrence of phenomena such as oxygen deficiency is a chance event, even rational management may be faced with an exceptional event that leads to an apparent ‘failure’ of the policy. Handling this variability in the face of different human pressures is probably one of the major challenges for adaptive integrated management.
3.3.2 Non-linear processes may control environmental impacts
Increased levels of nutrient input to coastal systems leads, in general, to elevated levels of phytoplankton production. The organic matter thus produced may further overload the ecosystem's capacity. However, it is too simplistic to assume that the effects of elevated production of phytoplankton are directly (or linearly) proportional to the increased nutrient input. We already discussed the impact of physical conditions in the previous section. However, the chemical nature of the nutrient input may also play a role. An illustrative case is the importance of stoichiometry (nutrient ratios) in the nutrient input to the Black Sea. Phytoplankton species require nutrients in characteristic proportions that are close to the value observed in their own biomass (the so-called Redfield ratio). Well-balanced N:P:Si nutrient input, i.e. nutrient input that adds nutrients according to the Redfield ratio, is easy to use for the phytoplankton. Growth is not limited by a single nutrient, as the nutrients will be exhausted more or less simultaneously. Relatively large, fast-growing phytoplankton species such as diatoms, can take full advantage of well-balanced nutrient input. This phytoplankton is the basis of the so-called linear food chain, i.e. the transfer from diatoms to copepods to fish and higher trophic levels.
However, when the nutrients are added in imbalance, with either nitrogen or phosphorus being added in much greater amount than the other nutrients (compared to the Redfield ratio), then a shortage of one nutrient will occur while the other nutrients are still largely available. Specialist, usually very small phytoplankton species, typically take advantage of these conditions and out compete diatoms. They are mostly consumed in the bacteria – microzooplankton dominated food web (Lancelot et al. Citation2002).
Whereas with balanced nutrient inputs the transfer of nutrients to higher trophic levels (fish) is more efficient, unbalanced inputs lead to nutrient regeneration in the microbial food web and to extension of the duration of the phytoplankton bloom. This clearly shows that the effect of nutrient inputs on the marine environment is not just a function of the concentrations but also depends on the nutrient composition.
The management problem illustrated by this example is important and difficult to handle. When, based on ecological considerations, it is decided to reduce nutrient inputs into the coastal zone, but success is much easier to obtain with one nutrient than with another, should one then go for maximal reduction of the easiest nutrient (‘do what you can’) or postpone action on this nutrient because it is upheld by the other (‘only do what you can do well’)? Experience has shown that phosphorus reduction has been much easier in Europe than nitrogen reduction, because phosphorus is associated with exchangeable products such as washing powder, and can also be treated relatively easily in point sources such as sewage treatment plants. This has had demonstrated effects on coastal phytoplankton species composition (Philippart et al. Citation2000) and probably also on higher trophic levels (Rousseau et al. Citation2000). A very thorough understanding of nutrient cycles and food webs is needed in order to make predictions on the likely outcome of the present unbalanced nutrient inputs, and on the need to change these in the long term.
3.3.3 Gaps in knowledge on the determinism of environmental impacts
The many studies conducted on the effects of nutrient enrichments on biological communities are mostly focused on the alterations following the environmental changes that accompany the increase in eutrophication. Less, however, is known about the direct effects of nutrient enrichment on the food web considered as the whole set of trophic interactions between the organisms or (functional) groups of organisms. Recent technological advances, e.g. in the use of stable isotopes for tracing food webs, are rapidly increasing our knowledge, especially of benthic systems (e.g. Herman et al. 2000). It remains a formidable scientific challenge, though, to relate changes in the functioning of coastal communities (such as those following nutrient additions) to structural changes and biodiversity. We believe that this point, both for benthic and pelagic communities, will be an important focus of further studies.
Management criteria that are based on structural characteristics and ‘ecological integrity’ of coastal waters, such as those being implemented in the Water Framework Directive, are directly confronted with the lack of fundamental understanding of function – structure relationships. It can be anticipated that concepts, criteria and scenarios for these management options will have to change with increasing scientific understanding.
3.4 From environmental impacts to policy response
The last step in the DPSIR approach consists of the critical phase when customized policy responses have to be defined as a remediation for the deleterious environmental effects observed in the coastal environment. This implies the whole chain of causal links and constraining factors between the nutrient sources and the potential targets to be taken into account. This can only be achieved by using integrated models aimed at reproducing the journeys of nutrients on their way between the emission sources and the potential targets in the coastal area.
Beside advanced research on individual processes, much effort has also been devoted within the ELOISE pool to the development of modelling tools required to track nutrients during their transport and transformation processes. There is a clear parallel between this modelling effort and the structure of the DPSIR approach that is articulated around the cascade of causal relations between the man-made driving forces and their ecological impacts. As such, the modelling tools that have been developed and/or their conceptual basis are well suited to support the definition of adequate remediation responses to the ecological impacts of elevated nutrient inputs to the coastal areas.
3.4.1 Atmospheric deposition
Modelling efforts deployed within the ANICE (Atmospheric Nitrogen Inputs into the Coastal Ecosystem) project (de Leeuw et al. Citation2001) succeeded in simulating/quantifying the marine deposition of atmospheric inputs of nitrogen compounds at an unequalled spatio-temporal resolution (de Leeuw et al. Citation2003). Emission sources were clearly identified and quantified (Hertel et al. Citation2002) as 40% from agricultural activity and 60% due to combustion sources (mainly traffic, industry and power production). The resolution scales of the monitoring effort required for a consistent assessment should match the scales of the processes taken into account in the model (≈day, ≤30 × 30 km grid) and be conducted (at least) at the European scale. State-of-the-art modelling of atmospheric processes, combined with a representative monitoring effort, are indispensable ingredients for the formulation of efficient policy measures related to atmospheric emission/deposition.
3.4.2 Rivers and groundwater
Full deterministic models such as INCA (Integrated Nitrogen in Catchments) have shown their suitability for management purposes such as the assessment of the various nutrient sources in catchments and/or the simulation of management scenarios (Jarvie et al. Citation2002). The application of this class of model is, however, limited to areas with a high density of data. A promising alternative is proposed with models applying a combination of deterministic and statistical approaches (Forsman & Grimvall Citation2003) where, for example, the nutrient flux is derived from the number of inhabitants in catchments. The latter approach was successfully applied to scenario studies (Forsman & Grimvall Citation2003) and implemented in a decision support system (Forsman et al. Citation2003). However, further validation and development of statistical models, as well as further integration of statistical and deterministic modelling approaches may be needed.
3.4.3 Nutrient transformations
A fully coupled biogeochemical model, linking the fate of different nutrients (N, P, Si) to that of organic matter in river systems, has been applied in the EROS project (RIVERSTRAHLER model, Garnier et al. Citation2002). This type of modelling is much needed, as it is very clear that rivers, lakes and reservoirs cannot be considered as passive pathways for nutrients to the coastal seas. Further development of this type of models, as well as interfacing them with estuarine and coastal models, is an important priority for research. Support for management systems, especially at the watershed scale, can be obtained if these models are made operational for many European watersheds. In view of the importance of nutrient ratios in the coastal zone (see above), it is a prerequisite that the models simulate the fate of different nutrients (nitrogen, phosphorus, silicate) and of organic matter in a coupled way. This will require further process studies, both in rivers and estuaries.
The bulk of the nutrient transformations are supported by biogeochemical cycles in surface sediments and rapid developments in the integrated modelling of these processes have been witnessed during the last decade. A promising attempt has been made within the ELOISE pool to develop user-friendly and flexible modelling environments (MEDIA) integrating the present know-how (Meysman et al. Citation2003a, b). Benthic biogeochemical processes fuelled by deposition of material from the water column and affected by the overlying bottom water are however essentially pelagically driven. As a result, coupled physical – pelagic – benthic biogeochemical models are required to account for this interaction (Soetaert et al. Citation2000). Along the broad complexity scale of models, from fully coupled to ‘bottomless’ models, Soetaert et al. (Citation2000) acknowledge that the most complex models may not always be the most suitable and recommend optimizing the matching between the ecological issues and the modelling tool to be used. In the same vein, Tett et al. (Citation2003) also highlight the use of simple as well as complex models in helping to understand eutrophication.
3.4.4 Food-web impacts
Coupled models are required to reproduce and predict the flux of nutrients through the complex and dynamic trophic network within food webs. As a successful example, the BIOGEN ecosystem model (Lancelot et al. Citation2002) showed its ability to stress the role played by overfishing and eutrophication in destabilizing the Black Sea system. The orientation of management criteria towards structural aspects of the biological community will require further development and application of this type of model with a high biological resolution.
4 Conclusion
The four issues described here have critical implications for our ability to detect the effects of man-made disturbance and for the choice of adapted mitigation measures. In discussing these issues, a number of problems for management have been pointed out.
We have stressed the important issues of spatial and temporal scale. Apart from the general problem of scale mismatch between human organizational systems (e.g. countries, provinces) and the scales of natural processes, the wide range of relevant scales within the natural processes poses a critical problem. Appropriate models are an essential tool to bridge the gap between the smallest and largest scales. From the management perspective, the biggest problem is how to organize a system where appropriate and coordinated action can be taken at all scales, from local communities to the European level.
In several places, we have stressed the need for environmental data, or suitable proxies, in order to run the models that are needed for realistic nutrient scenarios. Coordination of efforts and compilation of high-quality databases at the European scale seems a prerequisite for advances in integrated coastal zone management at this political scale.
The technical tools such as databases and good models applicable to different regions within Europe, are all the more needed because it becomes increasingly clear that (a) management objectives are phrased in terms of resulting ecological structure and (b) the cause – effect relations are very different between (sub)regions in Europe. In order to preserve equity and to combine political and environmental goals, coherence in this management structure at the European scale is needed. This is an important challenge for the European Commission.
All through this text, we have pointed out that the fundamental scientific knowledge of essential processes in nutrient cycling is subject to important change. Continuing efforts to deepen our insight are needed if management is to obtain its ambitious goals. Especially when structural variables, related to biodiversity, are formulated as the management goals, and this evolution seems inevitable as many ‘goods and services’ of coastal systems are directly related to these structural elements, much more knowledge of the function – structure relation is needed.
Acknowledgements
This study was funded by the Commission of the European Union in the framework of the European Land Ocean Interaction Studies (ELOISE) programme. We thank Dr Tom Ysebaert for the initial assembling of the bibliographic references used in the present article. We also thank Dr Keith Weston and Professor Dr Paul Tett for their comments on an earlier version of the manuscript. This is contribution no. 3791 of the Netherlands Institute of Ecology (NIOO-KNAW).
References
- Blackford , J C , Allen , J I and Gilbert , F J . 2004 . Ecosystem dynamics at six contrasting sites: A generic modelling study . J Mar Syst , 52 : 191 – 215 .
- Borum , J . 1996 . “ Shallow waters and land/sea boundaries ” . In Eutrophication in coastal marine ecosystems , Edited by: J⊘rgensen , B B and Richardson , K . 179 – 203 . Washington, DC : American Geophysical Union .
- Cloern , J E . 2001 . Our evolving conceptual model of the coastal eutrophication problem . Mar Ecol Prog Ser , 210 : 223 – 253 .
- Dalsgaard , T and Thamdrup , B . 2002 . Factors controlling anaerobic ammonium oxidation with nitrite in marine sediments . Appl Environ Microbiol , 68 : 3802 – 3808 .
- de Leeuw , G , Cohen , L , Frohn , L M , Geernaert , G , Hertel , O , Jensen , B , Jickells , T , Klein , L , Kunz , G J Lund , S . 2001 . Atmospheric input of nitrogen into the North Sea: ANICE project overview . Cont Shelf Res , 21 : 2073 – 2094 .
- de Leeuw , G , Skjoyh , C A , Hertel , O , Jickells , T , Spokes , L , Vignati , E , Frohn , L , Frydendall , J , Schulz , M Tamm , S . 2003 . Deposition of nitrogen into the North Sea . Atmos Environ , 37 : 145 – 165 .
- de Leeuw , G , Spokes , L , Jickells , T , Skjoth , C A , Hertel , O , Vignati , E , Tamm , S , Schulz , M , Sorensen , L L Pedersen , B . 2003 . Atmospheric nitrogen inputs into the North Sea: effect on productivity . Cont Shelf Res , 23 : 1743 – 1755 .
- de Wit , R , Stal , L J , Lomstein , B A , Herbert , R A , van Gemerden , H , Viaroli , P , Cecherelli , V U , Rodriguez-Valera , F , Bartoli , M Giordani , G . 2001 . ROBUST: The ROle of BUffering capacities in STabilising coastal lagoon ecosystems . Cont Shelf Res , 21 : 2021 – 2041 .
- Dettmann , E H . 2001 . Effect of water residence time on annual export and denitrification of nitrogen in estuaries: A model analysis . Estuaries , 24 : 481 – 490 .
- Dorst , J . 1965 . Avant que nature meure , Neuchâtel and Paris : Delachaux et Niestlé .
- Elliott , M and de Jonge , V N . 2002 . The management of nutrients and potential eutrophication in estuaries and other restricted water bodies . Hydrobiologia , 475 : 513 – 524 .
- Escaravage , V , Herman , P MJ and Heip , C HR . 2004 . Nutrient dynamics in European water systems (a digest from the Eloise results) Available: http://www.eloisegroup.org/themes/nutrients/contents.htm
- Forsman , A and Grimvall , A . 2003 . Reduced models for efficient simulation of spatially integrated outputs of one-dimensional substance transport models . Environ Model Softw , 18 : 319 – 327 .
- Forsman , A , Grimvall , A , Scholtes , J and Wittgren , H B . 2003 . Generic structures of decision support systems for evaluation of policy measures to reduce catchment-scale nitrogen fluxes . Phys Chem Earth , 28 : 589 – 598 .
- Galloway , J N , Aber , J D , Erisman , J W , Seitzinger , S P , Howarth , R W , Cowling , E B and Cosby , B J . 2003 . The nitrogen cascade . Bioscience , 53 : 341 – 356 .
- Garnier , J , Billen , G , Hannon , E , Fonbonne , S , Videnina , Y and Soulie , M . 2002 . Modelling the transfer and retention of nutrients in the drainage network of the Danube River . Estuar Coast Shelf Sci , 54 : 285 – 308 .
- Grimvall , A , Stalnacke , P and Tonderski , A . 2000 . Time scales of nutrient losses from land to sea – a European perspective . Ecol Eng , 14 : 363 – 371 .
- Herman , P MJ , Ysebaert , T and Heip , C HR . 2005 . “ Land – ocean fluxes and coastal ecosystems – a guided tour of ELOISE results ” . In Managing European coasts – past, present and future , Edited by: Vermaat , J , Bouwer , L , Turner , K and Salomons , W . 21 – 58 . Berlin : Springer .
- Hertel , O , Skjoth , C A , Frohn , L M , Vignati , E , Frydendall , J , de Leeuw , G , Schwarz , U and Reis , S . 2002 . Assessment of the atmospheric nitrogen and sulphur inputs into the North Sea using a Lagrangian model . Phys Chem Earth , 27 : 1507 – 1515 .
- Jarvie , H P , Wade , A J , Butterfield , D , Whitehead , P G , Tindall , C I , Virtue , W A , Dryburgh , W and McGraw , A . 2002 . Modelling nitrogen dynamics and distributions in the River Tweed, Scotland: an application of the INCA model . Hydrol Earth Syst Sci , 6 : 433 – 453 .
- Lancelot , C , Staneva , J , Van Eeckhout , D , Beckers , J M and Stanev , E . 2002 . Modelling the Danube-influenced north-western continental shelf of the Black Sea. II: Ecosystem response to changes in nutrient delivery by the Danube River after its damming in 1972 . Estuar Coast Shelf Sci , 54 : 473 – 499 .
- Meysman , F JR , Middelburg , J J , Herman , P MJ and Heip , C HR . 2003a . Reactive transport in surface sediments. I. Model complexity and software quality . Comput Geosci , 29 : 291 – 300 .
- Meysman , F JR , Middelburg , J J , Herman , P MJ and Heip , C HR . 2003b . Reactive transport in surface sediments. II. Media: an object-oriented problem-solving environment for early diagenesis . Comput Geosci , 29 : 301 – 318 .
- Middelburg , J J and Nieuwenhuize , J . 2000 . Nitrogen uptake by heterotrophic bacteria and phytoplankton in the nitrate-rich Thames estuary . Mar Ecol Prog Series , 203 : 13 – 21 .
- Migon , C , Sandroni , V and Bethoux , J P . 2001 . Atmospheric input of anthropogenic phosphorus to the northwest Mediterranean under oligotrophic conditions . Mar Environ Res , 52 : 413 – 426 .
- Oenema , O . 2004 . Governmental policies and measures regulating nitrogen and phosphorus from animal manure in European agriculture . J Anim Sci , 82 : 196 – 206 .
- Olsen , Y , Reinertsen , H , Vadstein , O , Andersen , T , Gismervik , I , Duarte , C , Agusti , S , Stibor , H , Sommer , U Lignell , R . 2001 . Comparative analysis of food webs based on flow networks: Effects of nutrient supply on structure and function of coastal plankton communities . Cont Shelf Res , 21 : 2043 – 2053 .
- Oviatt , C , Doering , P , Nowicki , B , Reed , L , Cole , J and Frithsen , J . 1995 . An ecosystem level experiment on nutrient limitation in temperate coastal marine environments . Mar Ecol Prog Ser , 116 : 171 – 179 .
- Philippart , C JM , Cadee , G C , van Raaphorst , W and Riegman , R . 2000 . Long-term phytoplankton – nutrient interactions in a shallow coastal sea: Algal community structure, nutrient budgets, and denitrification potential . Limnol Oceanogr , 45 : 131 – 144 .
- Reigstad , M , Wassmann , P , Ratkova , T , Arashkevich , E , Pasternak , A and Oygarden , S . 2000 . Comparison of the springtime vertical export of biogenic matter in three northern Norwegian fjords . Mar Ecol Prog Ser , 201 : 73 – 89 .
- Rousseau , V , Becquevort , S , Parent , J Y , Gasparini , S , Daro , M H , Tackx , M and Lancelot , C . 2000 . Trophic efficiency of the planktonic food web in a coastal ecosystem dominated by Phaeocystis colonies . J Sea Res , 43 : 357 – 372 .
- Scheffer , M and Carpenter , S R . 2003 . Catastrophic regime shifts in ecosystems: linking theory to observation . Trends Ecol Evol , 18 : 648 – 656 .
- Schindler , D W . 1977 . Evolution of phosphorus limitation in lakes . Science , 195 : 260 – 262 .
- Soetaert , K , Middelburg , J J , Herman , P MJ and Buis , K . 2000 . On the coupling of benthic and pelagic biogeochemical models . Earth Sci Rev , 51 : 173 – 201 .
- Spokes , L J , Yeatman , S G , Cornell , S E and Jickells , T D . 2000 . Nitrogen deposition to the eastern Atlantic Ocean. The importance of south-easterly flow . Tellus Ser B Chem Phys Meteorol , 52 : 37 – 49 .
- Tett , P , Gilpin , L , Svendsen , H , Erlandsson , C P , Larsson , U , Kratzer , S , Fouilland , E , Janzen , C , Lee , J Y Grenz , C . 2003 . Eutrophication and some European waters of restricted exchange . Cont Shelf Res , 23 : 1635 – 1671 .
- Thamdrup , B and Dalsgaard , T . 2002 . Production of N-2 through anaerobic ammonium oxidation coupled to nitrate reduction in marine sediments . Appl Environ Microbiol , 68 : 1312 – 1318 .
- Vidal , M , Duarte , C M and Sanchez , M C . 1999 . Coastal eutrophication research in Europe: Progress and imbalances . Mar Pollut Bull , 38 : 851 – 854 .
- Vitousek , P M . 1994 . Beyond global warming – ecology and global change . Ecology , 75 : 1861 – 1876 .
- Vitousek , P M , Mooney , H A , Lubchenco , J and Melillo , J M . 1997 . Human domination of Earth's ecosystems . Science , 277 : 494 – 499 .
- Vollenweider , R A . 1976 . Advances in defining critical loading levels of phosphorus in lake eutrophication . Mem Ist Ital Idrobiol , 33 : 53 – 83 .
- Wade , A J , Durand , P , Beaujouan , V , Wessel , W W , Raat , K J , Whitehead , P G , Butterfield , D , Rankinen , K and Lepisto , A . 2002 . A nitrogen model for European catchments: INCA, new model structure and equations . Hydrol Earth Syst Sci , 6 : 559 – 582 .
- Welsh , D T , Castadelli , G , Bartoli , M , Poli , D , Careri , M , de Wit , R and Viaroli , P . 2001 . Denitrification in an intertidal seagrass meadow, a comparison of N-15-isotope and acetylene-block techniques: dissimilatory nitrate reduction to ammonia as a source of N2O? . Mar Biol , 139 : 1029 – 1036 .
- Wijsman , J WM , Herman , P MJ , Middelburg , J J and Soetaert , K . 2002 . A model for early diagenetic processes in sediments of the continental shelf of the Black Sea . Estuar Coast Shelf Sci , 54 : 403 – 421 .