ABSTRACT
Background
: Acute myeloid leukemia (AML) is regarded as a haematological malignancy and seriously threatens the public’s health. Circular RNA (circRNA) is gradually confirmed to be involved in the development of AML. The purpose of this study was to disclose the role of circRNA Potassium Voltage-Gated Channel Subfamily Q Member 5 (circ_KCNQ5) in AML.
Methods
: Quantitative real-time PCR (qPCR) and western blot were used for expression analysis. Colony formation assay, EdU assay and MTT assay were performed to determine cell proliferation. Flow cytometry assay was conducted to determine cell apoptosis. The predicted binding relationship between miR-622 and circ_KCNQ5 or RAS oncogene family member 10 (RAB10) was verified by dual-luciferase reporter assay.
Results
: The expression of circ_KCNQ5 was increased in bone marrow samples of childhood AML patients and AML cell lines. The knockdown of circ_KCNQ5 largely suppressed AML cell proliferation and promoted cell apoptosis. Circ_KCNQ5 directly bound to miR-622 and inhibited miR-622 expression. The cotransfection of miR-622 inhibitor reversed the effects of circ_KCNQ5 knockdown and thus recovered cell proliferation and depleted cell apoptosis. RAB10 was a target of miR-622, and circ_KCNQ5 bound to miR-622 to increase the expression of RAB10. MiR-622 restoration inhibited AML cell proliferation and induced cell apoptosis, while RAB10 overexpression abolished these effects.
Conclusion
: Circ_KCNQ5 high expression was associated with childhood AML malignant development, and circ_KCNQ5 participated in AML progression by regulating the miR-622/RAB10 pathway.
KEYWORDS:
Introduction
Acute myeloid leukemia (AML), the most common acute leukemia, is a highly aggressive hematopoietic stem cell malignancy with high morbidity and mortality [Citation1]. AML is rare in children, accounting for 25% of pediatric leukemia [Citation2]. It is the most common type of acute leukemia in adults, accounting for 80% of all cases [Citation3]. The prognosis of AML remains poor, however, there has been a significant improvement in overall survival over the past 30 years [Citation3]. Genetic disorder, chemotherapy or radiotherapy exposure, myelodysplastic syndrome and carcinogenic chemicals have been considered to be risk factors for AML [Citation4,Citation5]. However, the etiology of AML remains poorly understood, which needs further to be explored from novel insights.
Circular RNAs (circRNAs) are a cluster of non-coding RNA molecules whose function has been widely identified in various human diseases [Citation6]. Different from other linear transcripts, circRNAs are specific for their closed-loop structures, without 3′ and 5′ ends [Citation6]. CircRNAs are thus stable in expression compared to linear molecules, which makes circRNAs more suitable as biomarkers in disease diagnosis and treatment [Citation7]. With the development of RNA sequencing technology, numerous circRNAs that are closely involved in AML leukemogenesis have been discovered [Citation8]. These circRNAs are pivotal in regulating AML cell proliferation, differentiation and apoptosis [Citation8]. Accordingly, circRNAs are promising biomarker and therapeutic targets in AML, with diagnostic and prognostic values [Citation9]. Unfortunately, the function of a large number of circRNAs in AML is still unclear. The data from circRNA expression microarray in a previous study clarified that the expression of circ_0004136 was notably elevated in bone marrow samples from children AML patients [Citation10], suggesting that circ_0004136 was potentially involved in AML development. Potassium Voltage-Gated Channel Subfamily Q Member 5 (KCNQ5) is the parental gene of circ_0004136, and circ_0004136 is also known as circ_KCNQ5. The detailed function of circ_KCNQ5 in AML was still lacking and needs exploring.
CircRNA acts as molecular sponges to bind microRNAs (miRNAs) and thus relieved the inhibition of miRNA on the expression of downstream functional genes, which has been widely noted in accumulating studies [Citation11,Citation12]. More and more miRNAs and target genes are predicted and demonstrated to be involved in circRNA-mediated regulatory networks with the boom of bioinformatics [Citation13]. By analyzing the data from circinteractome and miRDB databases, miR-622 was shown to harbor binding site with circ_KCNQ5, and also bound to the 3′UTR of RAS oncogene family member 10 (RAB10). The implications of miR-622 and RAB10 in AML were partly noted in previous studies [Citation14,Citation15]. We aimed to determine whether miR-622 and RAB10 were located in the circ_KCNQ5-mediated regulatory networks.
Our present study examined the expression of circ_KCNQ5 in childhood AML bone marrow samples and cell lines. Besides, loss-function assays were conducted to determine the function of circ_KCNQ5 on the proliferation and apoptosis of AML cells. In addition, we confirmed the binding relationship between miR-622 and circ_KCNQ5 or RAB10, thus providing a novel regulatory mechanism of circ_KCNQ5 in AML development.
Materials and methods
Samples
Bone marrow samples were collected from childhood patients with AML and normal volunteers enrolled in the Gansu Provincial Maternity and Child-care Hospital. Patients were diagnosed with AML according to the World Health Organization Classification of Tumors of Hematopoietic and Lymphoid Tissues (2008). Bone marrow samples collected from healthy donors or patients with thrombocytopenic purpura or myelofibrosis were used as normal control. A total of 51 pairs of bone marrow samples were used in this study. The use of all samples was approved by patients’ guardian with written informed consent, and this study was permitted by the Ethics Committee of Gansu Provincial Maternity and Child-care Hospital.
Cell lines
Human AML cell lines, including Kasumi-1 and MV4-11, isolated from childhood patients (7-year-old and 10-year-old), were obtained from Procell (Wuhan, China). Human stromal cells, HS-5, were obtained from Yaji Biotech (Shanghai, China). All cells were cultured in RPMI1640 medium containing 10% FBS at a 37°C incubator supplemented with 5% CO2.
Quantitative real-time PCR (qPCR)
Total RNA was isolated from bone marrow samples or cells using RNA isolation reagent (Vazyme, Nanjing, China) and was quantified by a spectrophotometer. Then, total RNA was reversely transcribed into cDNA using the QuantiNova Reverse Transcription Kit (Qiagen). Next, qPCR was performed using SYBR Green PCR Kit (Qiagen), and the results were calculated using the 2−△△Ct formula, with U6 or GADPH as an internal reference. Primers used in qPCR were listed as follows:
circ_KCNQ5, F: 5′-CATTCGAATCTGGTCTGCGG-3′ and R: 5′-TGTGTGCTCAGGGATGGTAG-3′; KCNQ5, F: 5′-AGAACAAGGGGAAGCATCAAG-3′ and R: 5′-TAGCTTCTGACTCTGCTTCCG-3′; miR-622, F: 5′-CGCGACAGTCTGCTGAGGT-3′ and R: 5′-AGTGCAGGGTCCGAGGTATT-3′; RAB10, F: 5′-CGGTTTCCTGGGGCTATGTA-3′ and R: 5′-GAGGACAGTCTCTCTCCCGA-3′; U6, F: 5′-CTCGCTTCGGCAGCACA-3′ and R: 5′-AACGCTTCACGAATTTGCGT-3′; GAPDH, F: 5′-GGAGTCCACTGGCGTCTTCA-3′ and R: 5′-GGTTCACACCCATGACGAAC-3′.
Rnase R digestion and actinomycin D treatment
RNA samples were digested with RNase R (Astral Scientific) for 0.5 h at 37°C, and qPCR was then performed. Kasumi-1 and MV4-11 cells were exposed to Actinomycin D (Amresco, USA) and incubated for 0, 8, 16 or 24 h, and cells were next collected and used for RNA isolation and qPCR.
Subcellular distribution
Nuclear RNA and cytoplasmic RNA from Kasumi-1 and MV4-11 cells were separately isolated by Ribobio (Guangzhou, China). The expression level of circ_KCNQ5 in each fraction was examined by qPCR, with GAPDH or U6 as the internal reference in the cytoplasm or in the nucleus.
Cell transfection
Short hairpin RNA targeting circ_KCNQ5 (sh-circ_KCNQ5) was synthesized by Tsingke (Beijing, China) and packaged into lentiviral vector, using sh-NC as the control. MiR-622 mimic (miR-622), miR-622 inhibitor (anti-miR-622) and their negative controls (miR-NC and anti-miR-NC) were obtained from Ribobio. RAB10 was cloned into pcDNA vector for RAB10 overexpression by Tsingke, using pcDNA vector as the blank control. These oligonucleotides or vectors were introduced into cells using the Lipofectamine 3000 reagent (Invitrogen, Carlsbad, CA, USA) for transient transfection.
Colony formation assay
A total of 200 cells were seeded into 6-well plates in each well, and cells were then cultured at 37°C for 12 days. Colonies were fixed with methanol and stained with crystal violet. After washing with PBS, cell colonies were observed and counted under a microscope (Leica, Wetzlar, Germany).
Edu assay
Cell-Light EdU DNA Cell Proliferation Kit (Ribobio) was applied here for cell proliferation assessment. In brief, cells refreshed into a 96-well plate (5×103 cells/well) were cultured for 48 h. Then, EdU medium was added in each well to culture cells for 2 h. Cells were subsequently collected and stained with Appllo and DAPI. Images of EdU-positive cells were captured using a microscope.
Mtt assay
Kasumi-1 and MV4-11 cells were planted into a 96-well plate, with three replicates for one sample in three wells. Cells were cultured at 37°C and next treated with MTT reagent (Beyotime, Shanghai, China) at 0, 1, 2 or 3 days. After treatment by MTT, cells in each well were further treated with DMSO (Beyotime) to dissolve formazan. Subsequently, the OD value of the absorbance at 570 nm was measured by a microplate reader (BioTek, Winooski, Vermont, USA).
Western blot assay
Total protein was extracted using RIPA lysis reagent (Solarbio) and quantified by BCA assay kit (Solarbio). The equal amount of protein was loaded on 10% SDS-PAGE for separation and next transferred onto the PVDF membrane. After blocking, membranes were incubated with the primary antibodies, including anti-PCNA (ab92552; Abcam, Cambridge, MA, USA), anti-RAB10 (ab237703) and anti-GAPDH (ab9485). Then, the membranes were incubated with the goat anti-rabbit secondary antibody (ab205718). After that, the protein signals were clearly shown using the ECL reagent (Beyotime).
Flow cytometry assay
The apoptotic cells were examined using the Annexin V-FITC Apoptosis Detection Kit (Solarbio, Beijing, China) according to the guidelines of protocol. Cells were sorted and distinguished by a flow cytometer (Beckman Coulter, Miami, FL, USA).
Dual-luciferase reporter assay
The binding site between miR-622 and circ_KCNQ5 or RAB10 3′UTR was predicted by circinteractome (https://circinteractome.nia.nih.gov/) or miRDB (http://mirdb.org/). Then, the wild-type (WT) and mutant-type (MUT) sequence fragments of circ_KCNQ5 or RAB10 were synthesized and cloned into pmirGLO vector by Sangon (Shanghai, China). Dual-luciferase reporter assay was performed in Kasumi-1 and MV4-11 cells by transfecting with miR-622 or miR-NC and reporter plasmid, including circ_KCNQ5-WT, circ_KCNQ5-MUT, RAB10-WT or RAB10-MUT. After 48 h post-transfection, luciferase activity was examined using the Dual-Luciferase Reporter Assay System (Promega, Madison, WI, USA).
Statistical analysis
GraphPad Prism 7 (GraphPad Software Inc., San Diego, CA, USA) was used for data processing. Three independent experiments were performed in this study. Data were expressed as the mean ± standard deviation. The linear correlation between two parameters was analyzed by Pearson correlation analysis. We analyzed the difference in different groups using Student’s t-test or ANOVA. P-value less than 0.05 was defined as statistical significance.
Results
The expression of circ_KCNQ5 was increased in bone marrow samples of AML patients and AML cell lines
The expression and characteristics of circ_KCNQ5 were identified first. The expression of circ_KCNQ5 was notably increased in bone marrow samples from childhood AML patients compared to that from normal controls (A). Besides, the expression of circ_KCNQ5 was also elevated in AML-related cell lines, such as U937, THP-1, Kasumi-1 and MV4-11 cells, compared to HS-5 cells (B and S1A). Considering that circ_KCNQ5 expression was relatively higher in Kasumi-1 and MV4-11 cells, these two cell lines were adopted in our study for functional experiments. Compared to linear KCNQ5, circ_KCNQ5 was effectively resistant to RNase R digestion (C and D). Likewise, the expression of circ_KCNQ5 was rarely depleted by actinomycin D, while the expression of KCNQ5 was largely repressed by actinomycin D (E and F). Additionally, circ_KCNQ5 was mainly distributed in the cytoplasmic fraction of Kasumi-1 and MV4-11 cells but rarely distributed in the nucleus (G and H). The data suggested that circ_KCNQ5 was aberrantly expressed in AML.
Figure 1. Circ_KCNQ5 was overexpressed in AML bone marrow sample and AML cell lines. (A) The expression of circ_KCNQ5 in bone marrow samples from AML patients and normal controls was detected by qPCR. (B) The expression of circ_KCNQ5 in HS-5, Kasumi-1 and MV4-11 cells was detected by qPCR. (C and D) The effect of RNase R on the expression of circ_KCNQ5 and linear KCNQ5 was monitored by qPCR. (E and F) The effect of actinomycin D on the expression of circ_KCNQ5 and linear KCNQ5 was monitored by qPCR. (G and H) The distribution of circ_KCNQ5 in cytoplasmic RNA or nuclear RNA was determined by qPCR. *P < 0.05.
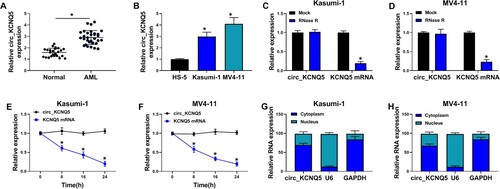
Circ_KCNQ5 knockdown inhibited AML cell proliferation and survival
Kasumi-1 and MV4-11 cells were infected with sh-circ_KCNQ5 to explore the role of circ_KCNQ5. The data showed that circ_KCNQ5 expression was strikingly decreased in Kasumi-1 and MV4-11 cells transfected with sh-circ_KCNQ5, while the expression of KCNQ5 was hardly changed after sh-circ_KCNQ5 transfection (A and B). Functionally, the knockdown of circ_KCNQ5 suppressed the ability of colony formation in Kasumi-1 and MV4-11 cells (C). Besides, EdU assay showed that the number of EdU-positive cells was markedly decreased by circ_KCNQ5 knockdown in Kasumi-1 and MV4-11 cells (D). In addition, MTT assay displayed that cell viability was significantly declined by circ_KCNQ5 knockdown in Kasumi-1 and MV4-11 cells at 1, 2 or 3 days after incubation (E and G). The expression of PCNA protein was also markedly reduced in Kasumi-1 and MV4-11 cells with sh-circ_KCNQ5 transfection (G). Flow cytometry assay displayed that circ_KCNQ5 knockdown enhanced the apoptotic rate of Kasumi-1 and MV4-11 cells (H). These data indicated that circ_KCNQ5 knockdown inhibited the proliferation and induced the apoptosis of AML cells.
Figure 2. Circ_KCNQ5 knockdown suppressed AML cell proliferation and promoted cell apoptosis. (A and B) The expression of circ_KCNQ5 and KCNQ5 in Kasumi-1 and MV4-11 cells transfected with sh-circ_KCNQ5 or sh-NC was detected by qPCR. (C) The effect of circ_KCNQ5 knockdown on cell proliferation was assessed by colony formation assay. (D) The effect of circ_KCNQ5 knockdown on cell proliferation was assessed by EdU assay. (E and F) The effect of circ_KCNQ5 knockdown on cell proliferation was assessed by MTT assay. (G) The protein level of PCNA in Kasumi-1 and MV4-11 cells transfected with sh-circ_KCNQ5 or sh-NC was measured by western blot. (H) The effect of circ_KCNQ5 knockdown on cell apoptosis was assessed by flow cytometry assay. *P < 0.05.
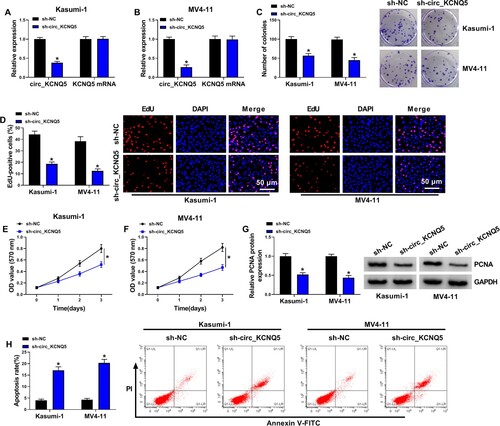
Circ_KCNQ5 bound to miR-622 and inhibited miR-622 expression
The data from circinteractome predicted a special binding site between circ_KCNQ5 sequence and miR-622 sequence (A). Further dual-luciferase reporter assay showed that miR-622 restoration significantly reduced luciferase activity in Kasumi-1 and MV4-11 cells transfected with circ_KCNQ5-WT but not circ_KCNQ5-MUT (B and C). The expression of miR-622 was notably enhanced in Kasumi-1 and MV4-11 cells after circ_KCNQ5 knockdown (D). Naturally, the expression of miR-622 was lower in Kasumi-1 and MV4-11 cells than that in HS-5 cells (E). Besides, the expression of miR-622 was also decreased in bone marrow samples from AML patients compared to normal controls (F). In these AML bone marrow samples, miR-622 expression was negatively correlated with circ_KCNQ5 expression (G). The data revealed that miR-622 was a target of circ_KCNQ5.
Figure 3. MiR-622 was a target of circ_KCNQ5. (A) The binding site between circ_KCNQ5 and miR-622 was predicted by circinteractome. (B and C) The binding between circ_KCNQ5 and miR-622 was verified by dual-luciferase reporter assay. (D) The expression of miR-622 affected by circ_KCNQ5 knockdown was detected by qPCR. (E) The expression of miR-622 in HS-5, Kasumi-1 and MV4-11 cells was detected by qPCR. (F) The expression of miR-622 in bone marrow samples from AML patients and normal subjects was measured by qPCR. (G) The linear relationship between circ_KCNQ5 expression and miR-622 expression in AML bone marrow samples. *P < 0.05.
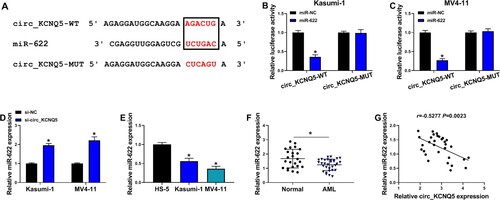
The inhibition of miR-622 partly abolished the effects of circ_KCNQ5 knockdown
Kasumi-1 and MV4-11 cells were transfected with sh-circ_KCNQ5 alone or sh-circ_KCNQ5 + anti-miR-622 to conduct rescue experiments. The data of qPCR exhibited that the expression of miR-622 was notably enhanced in Kasumi-1 and MV4-11 cells transfected with sh-circ_KCNQ5 alone but largely repressed in cells transfected with sh-circ_KCNQ5 + anti-miR-622 (A). The ability of colony formation in sh-circ_KCNQ5 + anti-miR-622 suppressed by sh-circ_KCNQ5 alone was recovered by the cotransfection of sh-circ_KCNQ5 + anti-miR-622 (B). EdU assay presented that the number of EdU-positive cells was inhibited by circ_KCNQ5 knockdown but increased by the addition of anti-miR-622 (C). MTT assay also maintained that the proliferation of Kasumi-1 and MV4-11 cells inhibited by alone circ_KCNQ5 knockdown was largely promoted by combined miR-622 knockdown (D and E). The protein level of PCNA was declined in Kasumi-1 and MV4-11 cells transfected with sh-circ_KCNQ5, while the expression of PCNA was partly restored in cells transfected with sh-circ_KCNQ5 + anti-miR-622 (F). Moreover, circ_KCNQ5 knockdown-induced cell apoptosis of Kasumi-1 and MV4-11 cells was relieved by further miR-622 inhibition (G). The data indicated that circ_KCNQ5 knockdown inhibited the proliferation and survival of AML cells by increasing miR-622 expression.
Figure 4. MiR-622 inhibition reversed the effects of circ_KCNQ5 knockdown. Kasumi-1 and MV4-11 cells were transfected with sh-circ_KCNQ5 alone or sh-circ_KCNQ5 + anti-miR-622. (A) The expression of miR-622 was measured by qPCR. (B-E) Cell proliferation in various cells was determined by colony formation assay, EdU assay and MTT assay. (F) The expression of PCNA in these cells was detected by western blot. (G) Cell apoptosis in these cells was detected by flow cytometry assay. *P < 0.05.
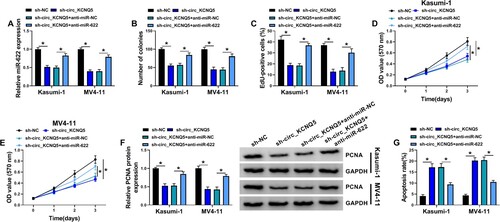
Rab10 was targeted by miR-622, and circ_KCNQ5 regulated RAB10 expression by targeting miR-622
The data from miRDB database predicted that miR-622 bound to RAB10 3′UTR via a special binding site (A). Dual-luciferase reporter assay showed that the cotransfection of miR-622 and RAB10-WT reporter plasmid strikingly reduced luciferase activity in Kasumi-1 and MV4-11 cells (B and C). Kasumi-1 and MV4-11 cells were transfected with miR-622 or anti-miR-622 to induce miR-622 overexpression or downregulation (D). Then, the expression of RAB10 mRNA and protein was markedly decreased in Kasumi-1 and MV4-11 cells after miR-622 overexpression but markedly enhanced after miR-622 downregulation (E and F). The expression of RAB10 mRNA and protein was remarkably enhanced in bone marrow samples from AML patients compared to normal controls (G and H). In addition, RAB10 mRNA expression was negatively correlated with miR-622 expression but positively correlated with circ_KCNQ5 expression in AML bone marrow samples (I and J). The expression of RAB10 mRNA and protein was also elevated in Kasumi-1 and MV4-11 cells compared with that in HS-5 cells (K and L). Furthermore, we found that the expression of RAB10 mRNA and protein was markedly decreased in Kasumi-1 and MV4-11 cells transfected with sh-circ_KCNQ5 but partly recovered in cells transfected with sh-circ_KCNQ5 + anti-miR-622 (M and N). These data indicated that circ_KCNQ5 regulated RAB10 expression by targeting miR-622.
Figure 5. Circ_KCNQ5 regulated RAB10 expression by targeting miR-622. (A) The binding site between miR-622 and RAB10 3′UTR was predicted by miRDB. (B and C) The binding between miR-622 and RAB10 was verified by dual-luciferase reporter assay. (D) MiR-622 mimic or anti-miR-622 was used to mediate miR-622 overexpression or miR-622 downregulation. (E and F) The expression of RAB10 mRNA and protein affected by miR-622 overexpression or miR-622 downregulation was measured by qPCR and western blot. (G and H) The expression of RAB10 mRNA and protein in bone marrow samples from AML patients and normal subjects was detected by qPCR and western blot. (I and J) The linear relationship between RAB10 expression and miR-622 expression or circ_KCNQ5 expression in samples. (K and L) The expression of RAB10 mRNA and protein in HS-5, Kasumi-1 and MV4-11 cells was detected by qPCR and western blot. (M and N) The expression of RAB10 mRNA and protein in Kasumi-1 and MV4-11 cells transfected with sh-circ_KCNQ5 alone or sh-circ_KCNQ5 + anti-miR-622 was measured by qPCR and western blot. *P < 0.05.
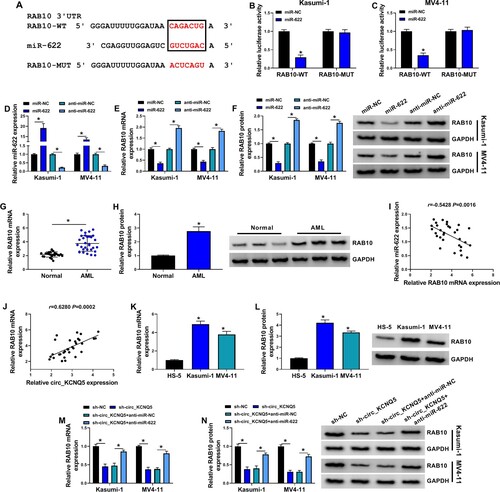
MiR-622 restoration inhibited AML cell proliferation and promoted cell apoptosis by inhibiting RAB10 expression
Kasumi-1 and MV4-11 cells were transfected with miR-622 alone or miR-622 + RAB10 for rescue experiments. The data showed that the expression of RAB10 was remarkably suppressed in Kasumi-1 and MV4-11 cells transfected with miR-622 alone but largely restored in cells transfected with miR-622 + RAB10 (A and B). In function, the ability of colony formation of Kasumi-1 and MV4-11 cells was markedly suppressed by miR-622 restoration but recovered by RAB10 reintroduction (C). EdU assay presented that miR-622 upregulation reduced the number of EdU-positive cells, and further RAB10 reintroduction enhanced the number of EdU-positive cells (D). MTT assay also displayed that cell viability after miR-622 transfection was notably declined, while cell viability after miR-622 + RAB10 transfection was partly enhanced (E and F). The protein level of PCNA was also decreased in Kasumi-1 and MV4-11 cells transfected with miR-622 alone but partly recovered in cells transfected with miR-622 + RAB10 (G). Moreover, miR-622 restoration-induced cell apoptosis was largely suppressed by RAB10 overexpression (H). These data suggested that miR-622 inhibited AML cell proliferation and survival by depleting RAB10.
Figure 6. RAB10 overexpression abolished the effects of miR-622 restoration. Kasumi-1 and MV4-11 cells were transfected with miR-622 alone or miR-622 + RAB10. (A and B) The expression of RAB10 mRNA and protein in these cells was detected by qPCR and western blot. (C-F) Cell proliferation in various cells was determined by colony formation assay, EdU assay and MTT assay. (G) The expression of PCNA in these cells was detected by western blot. (H) Cell apoptosis in these cells was detected by flow cytometry assay. *P < 0.05.
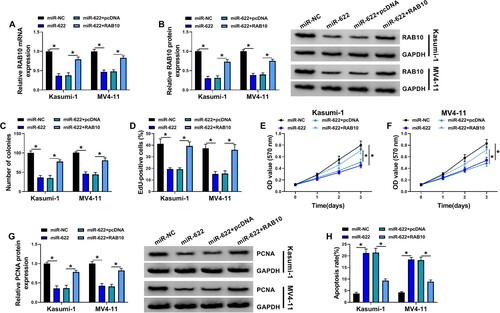
Discussion
Increasing evidence suggests that non-coding RNAs, such as circRNAs and miRNAs, are important regulators in AML. As shown in our study, we mainly found that circ_KCNQ5 expression was increased in bone marrow samples of childhood AML patients and AML cell lines. The downregulation of circ_KCNQ5 suppressed the proliferation and promoted the apoptosis of AML cells. Mechanism analysis found that circ_KCNQ5 served as miR-622 sponge to activate the expression of downstream RAB10, which was validated by rescue experiments. Our findings uncovered the functional role of circ_KCNQ5 in AML cell behaviors and provided a novel functional mechanism of circ_KCNQ5 in AML progression.
The role of numerous circRNAs in AML has been gradually established. For example, circ_0000370 was shown to be involved in FLT3-ITD-positive AML by enhancing AML cell viability and inhibiting cell apoptosis [Citation16]. Besides, circ_0079480 was highly expressed in AML bone marrow samples, and its silencing repressed AML cell growth and stimulated apoptosis [Citation17]. In addition, circ_0004277 expression was restored in AML after chemotherapy, and high circ_0004277 expression was associated with a good prognosis of AML [Citation18]. These findings highlighted the vital roles of circRNA in the development of AML. In terms of circ_KCNQ5, it was previously reported to be upregulated in children AML patients [Citation10]. Besides, this study demonstrated that circ_KCNQ5 overexpression promoted AML cell proliferation [Citation10]. Similarly, a recent study also determined that circ_KCNQ5 knockdown blocked AML cell viability and arrested cell cycle, mainly suggesting that circ_KCNQ5 knockdown inhibited AML cell growth [Citation19]. Consistent with these studies, our study presented that circ_KCNQ5 downregulation impaired cell proliferation and promoted cell apoptosis in AML cells, indicating that the inhibition of circ_KCNQ5 might be a promising strategy to block AML development in practice.
Considering the sponge effect of circ_KCNQ5 on miR-142 and miR-570-3p was demonstrated in previous studies, we speculated that circ_KCNQ5 might serve as a molecular sponge of other target miRNAs. By bioinformatics analysis and dual-luciferase reporter assay, miR-622 was screened as a target of circ_KCNQ5 because the expression pattern of miR-622 in AML was opposite to circ_KCNQ5. MiR-622 was previously recorded to be reduced in AML samples and cells, and its overexpression suppressed myelomonocytic cell proliferation, migration and invasion [Citation14]. The anti-tumor role of miR-622 has been widely addressed in various cancers, such as colon cancer, breast cancer and papillary thyroid carcinoma [Citation20–22], indicating that miR-622 was a well-established tumor suppressor in both hematologic malignancies and solid tumors. Our functional study showed that miR-622 inhibition reversed the effects of circ_KCNQ5 knockdown, leading to recovered proliferation and suppressive apoptosis of AML cells. Not surprisingly, miR-622 restoration largely impaired AML cell proliferation and promoted apoptosis.
Further study discovered that miR-622 bound to RAB10 3′UTR, and their binding was verified by dual-luciferase reporter assay. Interestingly, RAB10 expression in AML bone marrow samples was negatively correlated with miR-622 expression but positively correlated with circ_KCNQ5 expression. Besides, circ_KCNQ5 knockdown reduced RAB10 expression, while additional miR-622 inhibition partly recovered RAB10 expression. RAB10 was previously reported to be involved in the circRNA-100290 regulatory network, and circRNA-100290 promoted AML cell proliferation by upregulating RAB10 via sponging miR-203 [Citation23]. Similarly, long non-dong RNA TUG1 promoted AML cell viability and promoted cell death in AML cells also by increasing RAB10 expression via targeting miR-193a-5p [Citation15]. The data revealed that RAB10 promoted the progression of AML. Our study maintained that RAB10 overexpression abolished the effects of miR-622 restoration, thereby recovering cell proliferation and inhibiting cell apoptosis, which was consistent with previous studies.
Conclusion
Circ_KCNQ5 was highly expressed in childhood AML bone marrow samples and cell lines. Circ_KCNQ5 knockdown inhibited the progression of AML by suppressing AML cell proliferation and induced AML cell apoptosis. Circ_KCNQ5 bound to miR-622 to enhancing the expression of RAB10, thus promoting the progression of AML (). Our study mainly explored the role of circ_KCNQ5 in vitro and provided a new regulatory pathway of circ_KCNQ5 in AML, which strongly supported that circ_KCNQ5 inhibition was a strategy for AML treatment.
Availability of data and materials
Please contact the correspondence author for the data request.
Ethics approval and consent to participate
Written informed consent was obtained from patients with approval by the Institutional Review Board in Gansu Provincial Maternity and Child-care Hospita.
Supplemental Material
Download TIFF Image (209 KB)Disclosure statement
No potential conflict of interest was reported by the author(s).
References
- Desai P, Hassane D, Roboz GJ. Clonal hematopoiesis and risk of acute myeloid leukemia. Best Pract Res Clin Haematol. 2019;32:177–185.
- Taga T, Tomizawa D, Takahashi H, et al. Acute myeloid leukemia in children: current status and future directions. Pediatr Int. 2016;58:71–80.
- Cammarata-Scalisi F, Girardi K, Strocchio L, et al. Oral manifestations and complications in childhood acute myeloid leukemia. Cancers (Basel). 2020;12:1643.
- Fernandes KS, Gallottini M, Castro T, et al. Gingival leukemic infiltration as the first manifestation of acute myeloid leukemia. Spec Care Dentist. 2018;38:160–162.
- Misirlioglu M, Adisen MZ, Yilmaz S. Diagnosis of acute myeloid leukemia in a dental hospital; report of a case with severe gingival hypertrophy. Niger J Clin Pract. 2015;18:573–576.
- Santer L, Bar C, Thum T. Circular RNAs: a novel class of functional RNA molecules with a therapeutic perspective. Mol Ther. 2019;27:1350–1363.
- Meng S, Zhou H, Feng Z, et al. Circrna: functions and properties of a novel potential biomarker for cancer. Mol Cancer. 2017;16:94.
- Liu Y, Cheng Z, Pang Y, et al. Role of microRNAs, circRNAs and long noncoding RNAs in acute myeloid leukemia. J Hematol Oncol. 2019;12:51.
- Ji T, Chen Q, Tao S, et al. The research progress of circular RNAs in hematological malignancies. Hematology. 2019;24:727–731.
- Yuan DM, Ma J, Fang WB. Identification of non-coding RNA regulatory networks in pediatric acute myeloid leukemia reveals circ-0004136 could promote cell proliferation by sponging miR-142. Eur Rev Med Pharmacol Sci. 2019;23:9251–9258.
- Su Q, Lv X. Revealing new landscape of cardiovascular disease through circular RNA-miRNA-mRNA axis. Genomics. 2020;112:1680–1685.
- Zhou J, Qiu C, Fan Z, et al. Circular RNAs in stem cell differentiation: a sponge-like role for miRNAs. Int J Med Sci. 2021;18:2438–2448.
- Dori M, Bicciato S. Integration of bioinformatic predictions and experimental data to identify circRNA-miRNA associations. Genes (Basel). 2019;10:642.
- Alfano D, Gorrasi A, Li Santi A, et al. Urokinase receptor and CXCR4 are regulated by common microRNAs in leukaemia cells. J Cell Mol Med. 2015;19:2262–2272.
- Li Q, Wang J. Lncrna TUG1 Regulates Cell Viability and Death by Regulating miR-193a-5p/Rab10 Axis in Acute Myeloid Leukemia. Onco Targets Ther. 2020;13:1289–1301.
- Zhang L, Bu Z, Shen J, et al. A novel circular RNA (hsa_circ_0000370) increases cell viability and inhibits apoptosis of FLT3-ITD-positive acute myeloid leukemia cells by regulating miR-1299 and S100A7A. Biomed Pharmacother. 2020;122:109619.
- Hu Q, Gu Y, Chen S, et al. Hsa_circ_0079480 promotes tumor progression in acute myeloid leukemia via miR-654-3p/HDGF axis. Aging (Albany NY). 2020;13:1120–1131.
- Li W, Zhong C, Jiao J, et al. Characterization of hsa_circ_0004277 as a new biomarker for acute myeloid leukemia via circular RNA profile and bioinformatics analysis. Int J Mol Sci. 2017;18:597.
- Bi J, Pu Y, Yu X. Exosomal circ_0004136 enhances the progression of pediatric acute myeloid leukemia depending on the regulation of miR-570-3p/TSPAN3 axis. Anticancer Drugs. 2021;32(8):802–811.
- Orlandella FM, Mariniello RM, Mirabelli P, et al. miR-622 is a novel potential biomarker of breast carcinoma and impairs motility of breast cancer cells through targeting NUAK1 kinase. Br J Cancer. 2020;123:426–437.
- Wang R, Ma Q, Ji L, et al. miR-622 suppresses tumor formation by directly targeting VEGFA in papillary thyroid carcinoma. Onco Targets Ther. 2018;11:1501–1509.
- Zhang Y, Li Z, Lan Z. RETRACTED ARTICLE: Silencing UNC5B antisense lncRNA 1 represses growth and metastasis of human Colon cancer cells via raising miR-622. Artif Cells Nanomed Biotechnol. 2020;48:60–67.
- Fan H, Li Y, Liu C, et al. Circular RNA-100290 promotes cell proliferation and inhibits apoptosis in acute myeloid leukemia cells via sponging miR-203. Biochem Biophys Res Commun. 2018;507:178–184.