ABSTRACT
Background
Hemorrhagic cystitis (HC) is a severe complication of allo-HSCT, characterized by irritative symptoms of the urinary tract and a higher morbidity rate. The risk factors and prognosis of HC are still unclear.
Objective
The objective of this study is to identify risk factors and outcomes to improve treatment in pediatric SAA patients undergoing HSCTs in the Children's Hospital of Soochow University.
Methods
A total of 97 SAA patients as a cohort were enrolled from 2010 to 2019 in the Children's Hospital of Soochow University and a number of factors related to HC and outcomes were analysed. In all transplants (except UCBT), patients received a combination of G-CSF stimulated bone marrow (BM) and peripheral blood stem cell (PBSC). The minimum number of CD34 + cells is 5 × 106 cells/kg.
Results
Mononuclear cells dose (MNC, cut off: 8.53 × 108/kg) and grade II–IV acute graft versus host disease (aGVHD) were identified as independent risk factors for HC. Patients without HC had better overall survival (OS) than with HC (No HC: 98.6%±1.4% vs HC: 87.4% ± 6.8%, p = 0.03).
Conclusion
We concluded that aGVHD and MNC dose in graft might play an important role in the development of HC in pediatric SAA patients undergoing allo-HSCT. HC is also a key complication affecting the prognosis of children with SAA after allo-HSCT.
Introduction
Aplastic anemia (AA) is a rare but potentially fatal disorder characterized by hypocellular bone marrow and pancytopenia [Citation1]. Severe aplastic anemia (SAA) is a life-threatening type which allogeneic hematopoietic stem cell transplantation (allo-HSCT) and immunosuppressive therapy(IST) are the available curative approaches [Citation2,Citation3]. However, IST has obvious shortcomings, approximately one-third of patients did not respond to IST and one-third of responders relapsed after initial therapy [Citation4,Citation5]. HSCT from an HLA-matched related donor (MRD) is the first-line treatment for children with SAA [Citation6]. But due to the limitation of the source of MRD, unrelated cord blood transplantation (UCBT), haplo-identical donor (HID) and matched unrelated donor (MUD) of allo-HSCT are gradually applied in the treatment of SAA [Citation7–10]. The long-term disease-free survival rate of allo-HSCT from different donor types for the treatment of SAA over 85%. Although allo-HSCT for SAA has achieved a high disease-free survival rate, complications related to allo-HSCT are still important factors affecting the quality of life of children. Among them, hemorrhagic cystitis (HC) is a potential life-threatening complication after allo-HSCT [Citation11].
HC is an inflammatory process of the bladder that occurs after allo-HSCT and is a challenging condition to treat [Citation12]. The potential for life-long sequelae from fibrosis and contracture of the bladder is of great consequence in pediatric patients. Clinically, HC is characterized by hematuria, dysuria, irritative symptoms of the urinary tract and even renal failure due to hydronephrosis [Citation13,Citation14]. Early onset HC, often caused by cyclophosphamide (CY), busulfan, and irradiation [Citation15]. And Late onset HC is often associated with a consequence of virus reactivation, most commonly involving BK virus (BKV), but also adenovirus or cytomegalovirus (CMV) [Citation16,Citation17]. Many studies have explored the risk factors for HC after HSCT. Older age, acute Graft versus host disease (aGVHD), HLA matching, T-cell depletion, and severe immunosuppression have been implicated as risk factors to late onset HC [Citation12]. However, to the best of our knowledge, the outcomes and risk factors of HC following allo-HSCT in a pediatric cohort with SAA have not yet been established. Therefore, in the present study, we addressed this question by retrospectively analyzing the possible risk factors (such as age, sex, disease, disease course, donor type, HLA matching, ABO match, CMV seropositive, EBV seropositive, neutrophil engraftment time, platelet engraftment time, MNC, CD34, engraftment syndrome, aGVHD, aGVHD localization) and outcomes (such as cGVHD and overall survival) of HC in 97 pediatric SAA patients after allo-HSCT. We hope that through our research we could identify the most potentially close factors and help in dealing with HC to improve the quality of life of children with SAA after allo-HSCT.
Methods and patients
Patients
This study included 97 pediatric patients (aged 1–18 years), who were diagnosed with AA received allo-HSCT in Children's Hospital of Soochow University, Suzhou, China from 2010 to 2019. Due to the retrospective nature of the study, it was not necessary to request informed consent from the patients, but patient confidentiality was strictly maintained. All patients and stem cell donors signed an informed consent before beginning the transplant process. The criteria for selection of patients were as follows: (i) diagnosed with SAA. Patients with congenital bone marrow disorders (Fanconi anemia, Diamond-Blackfan anemia, and dyskeratosis congenital (DKC)) were excluded. (ii) aged 0–18 years at the time of transplantation; (iii) no prior HSCT.
Transplantation procedure
Conditioning therapy in HID(RIC) and MUD(RIC) consisted of the following: 45 mg/m2/d fludarabine on days -7,-6,-5 and -4; 3.2 mg/kg/d busulfan (BU) on days -7 and -6; 30 mg/kg/d cyclophosphamide (CY) on days-5, -4, -3, and -2; and 2.5 mg/kg/d ATG on days -5, -4, -3, and -2 (Figure S1). Conditioning therapy in MRD (RIC): 40 mg/m2/d fludarabine on days -6,-5,-4 and -3; 40 mg/kg/d CY on days-5, -4, -3, and -2; and 2.5 mg/kg/d ATG on days -5, -4, -3, and -2 (Figure S2). Conditioning therapy in UCB(MAC): 45mg/m2/d fludarabine on days -5,-4,-3 and -2; 3.2 mg/kg/d BU on days -9,-8 and -7; 40 mg/kg/d CY on days -6,-5 and -42.5 mg/kg/d; ATG on days -8, -7, -6, and -5 (Figure S3). In all transplants (except UCBT), patients received a combination of G-CSF stimulated bone marrow (BM) and peripheral blood stem cell (PBSC). The minimum number of CD34+ cells is 5 × 106 cells/kg. All patients received G-CSF treatment from +6 of transplantation until neutrophil count being >1 × 109 cells/L. The aGVHD prophylaxis for patients receiving HID, MUD, and MRD included cyclosporin A (CsA, blood concentration 200–250 ng/ml) plus 15 mg/m2 of oral methotrexate (MTX) on days +1, and MTX 10 mg/m2 on +3, +6, and +11 post-transplantation. Patients who received stem cells from UCB were treated with CsA (blood concentration at 100–150 ng/ml). Mycophenolate mofetil (MMF) was used in all patients (20–30 mg/kg/d) for a period of 30 days and a half dose for 15 more days after allo-HSCT. Corticosteroids (CS) were not included in the prophylaxis. The prevention of uroepithelial damage by CY was mediated by Mesna (40% of the CY dose, q4h × 3/d, 3 days) and hydration (3.5–4 L/m2), from commencement to 24 h after the end of the CY infusion. Heparin and alprostadil were used for preventing hepatic venous occlusive disease (VOD). Ganciclovir, acyclovir, micafungin and meropenem were used for preventing viral, fungal, and bacterial infection, respectively. Treatment of hemorrhagic cystitis was according to standard operating procedures, depending on severity [Citation12].
Immunophenotypic analysis
PB samples were collected using a heparin tube. PB mononuclear cells (PBMCs) were separated within 2 h using Ficoll-Paque (Sigma, San Francisco, CA, USA). T cells were tested using FITC-CD3, PE-CD4, and APC-CD8. B cells were tested using PE-CD19. NK cells were tested using FITC-CD16 and APC-CD56. All antibodies used for immunofluorescence staining were purchased from BD Biosciences (San Jose, CA, USA). Abs staining were performed according to the manufacturer’s instructions. Cells were then washed twice in staining buffer (Phosphate Buffered Saline + 2.5% Fatal Bovine Serum + 0.05% NaN3). Flow cytometry analyses were performed using Flowjo software (BD Biosciences, CA) and Gallios cytometers(Beckman, Los Angeles, CA, USA).
Definitions
Hemorrhagic cystitis was defined as microscopic or macroscopic hematuria and dysuria with a negative bacterial culture in the urine and no other hemorrhagic conditions. The symptoms of HC are classified as follows: grade 1 shows microscopic hematuria; grade 2, macroscopic hematuria; grade 3, macroscopic hematuria with clots, and grade 4, macroscopic hematuria with clots, urinary obstruction, kidney damage, and bladder damage [Citation18]. Overall survival (OS) was considered as time from allo-HSCT to death. Neutrophil engraftment was defined as achieving an absolute neutrophil count ≥0.5 × 109/L for 3 consecutive days. Platelet engraftment is defined as achieving a platelet count ≥20 × 109/L without transfusion support for 7 consecutive days. Myeloablative conditioning regimen, defined as busulfan (BU) >8 mg/kg. The diagnosis and grading of acute GVHD (aGVHD) and chronic GVHD (cGVHD) were assigned by the transplant center using standard criteria [Citation19,Citation20]. Engraftment syndrome (ES) was defined as fever in absence of infection, skin rash, diarrhea, capillary leak syndrome, and pulmonary injury which occur before or during neutrophil engraftment [Citation21].
Statistical analysis
To compare differences between groups, the chi-square test was used for categorical variables and the Mann–Whitney U test for continuous variables. The univariate and multivariate analysis of prognostic factors was performed with a logistic regression model to estimate the odds ratio(OR) effect of variables on HC. Variables with a p < 0.10 in univariate analyses were included in the multivariate model. Receiver operating characteristic (ROC) analyses were performed and areas under the curve (AUC) were calculated with HC as the actual state variable. Analyses of OS, CI of ES, aGVHD, cGVHD were performed using the Kaplan–Meier method, with differences compared by log-rank tests. All statistical analyses were performed using SPSS version 22.0(IBM, Armonk, NY).
Result
Characteristics of patients
A total of 97 patients were eligible for this study. Divide the patients into two groups (HC group vs No HC group) according to the presence or absence of hemorrhagic cystitis. The characteristics of patients and transplantation are presented in and .
Table 1. Patient characteristics.
Table 2. Characteristics of patients with HC.
In No HC group, 38 males and 33 females, with a median age of 7.75 years (range 1–15.75 years). Forty-seven patients (66%) were SAA, and the remaining 24 patients (34%) were VSAA. Forty patients (56%) underwent HSCT with a HID, 19 (27%) with a MRD, 1 (1%) with a UCB, and 11(16%) with a MUD. The median time interval from SAA diagnosis to SCT was 4.3 months (range 1–114.4 months). The median MNC and CD34 +��cell doses were 6.04 × 108/kg (range 1.32–14.89 × 108/kg) and 4.97 × 106/kg (range 0.16–12.50 × 106/kg), respectively.
There are 26 patients in the HC group (14 males and 12 females), with a median age of 5.71 years (range 2.42–11.33 years). The overall cumulative incidence of HC (grade 1–4) was 27% (26 of 97 patients). The median time to HC onset was 21 days (range 1–95 days). Median time to HC duration was 14 days (range 3–95 days). There were 5 patients with grade I HC, 15 patients with grade II HC, and 6 patients with grade III HC, there were no patients with grade IV HC. Eleven patients (42%) were SAA, and 15 patients (58%) were VSAA. Seventeen patients (65%) underwent HSCT with a HID, 3(12%) with a MRD, 4(15%) with a UCB, and 2 (8%) with a MUD. The median time interval from SAA diagnosis to SCT was 3.08 months (range 0.73–76.97months). Median MNC and CD34 + cell doses were 8.71 × 108/kg (range 0.27–17.43 × 108/kg) and 4.75 × 106/kg (range 0.06–10.42 × 106/kg), respectively.
Immune reconstitution and HC
Next, we evaluated whether the immune reconstitution impacts the risk of HC. Medium time to myeloerythroid reconstitution in the no HC group was 11 days (range 8–22 days) for neutrophils and 15 days (range 7–47 days) for platelets. Among the HC-positive patients, this was 12 (neutrophils, range 10–21 days) and 18 (platelet 8–52 days) days. The time of platelet engraftment and neutrophil engraftment in the HC group was later than that in the no HC group, and the difference was statistically significant (, neutrophil: p = 0.02, platelet: p = 0.006). The cumulative incidences of 30-day neutrophil and platelet engraftment were 100% (neutrophil: p = 0.08, (A), platelet: p = 0.03, (B)) in the HC and no HC groups.
Figure 1. Neutrophil and platelet engraftment probability of AA patients after HSCT. Neutrophil and platelet engraftment were compared between HC group and no HC group.(A) Neutrophil engraftment probability of patients after HSCT.(p = 0.08) (B)Platelet engraftment probability of patients after HSCT. (p = 0.03).
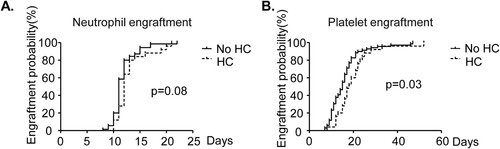
We evaluated whether the frequency and cell count of CD3+ T cells, CD4+T cells, CD8+T cells, CD19+ B, CD16+ CD56+ NK cells and total lymphocyte count at D30 after HSCT impact the risk of HC. There was no statistically significant difference in lymphocyte subsets in patients with and without HC ().
Figure 2. Lymphocyte subsets were not associated with HC. The frequency and counts of CD3+T cells, CD4+T cells, CD8+T cells,CD19+B cells and CD16+CD56+NK cells were detected by flow cytometry at D30 after HSCT. Lymphocyte subsets were compared between HC group and no HC group.(A)The frequency of CD3+T.(B) The counts of CD3+T cells.(C)The frequency of CD4+T cells.(D) The counts of CD4+T cells. (E)The frequency of CD8+T cells.(F)The counts of CD8+T cells.(G)The frequency of CD19+B cells.(H)The counts of CD19+B cells. (I)The frequency of CD16+CD56+ NK cells.(J)The counts of CD16+CD56+ NK cells.(K) The counts of total lymphocyte.
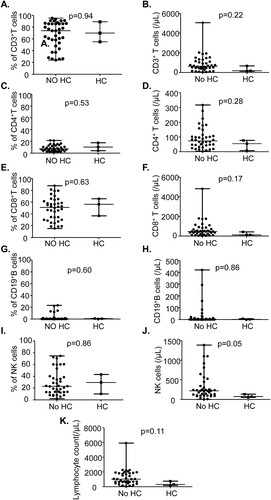
Risk factors for HC
Further, we evaluated a number of other parameters for a possible association with increased risk of HC using univariate analysis, including age, sex, disease, disease course, donor type, HLA matching, ABO match, CMV seropositive, EBV seropositive, neutrophil engraftment time, platelet engraftment time, MNC, CD34, engraftment syndrome, aGVHD, and aGVHD localization (). Disease (SAA vs VSAA, OR:2.67, 95%CI: 1.06–6.07, p = 0.036), donor type(UCB vs others, OR:12.7, 95% CI: .35–119.92, p = 0.026), MNC (OR:1.16, 95%CI: 1.01–1.33, p = 0.04), aGVHD (grade 0–I vs grade II–IV, OR: 6.77, 95%CI: 2.14–21.39, p = 0.001), aGVHD localization (Gut ± others vs others, OR:5.73, 95%CI: 1.79–18.35, p = 0.003) measured as continuous variables, were significantly associated with an increased risk of HC development. Subsequently, we performed a multivariable analysis including disease (SAA vs VSAA), donor type (UCB vs others), neutrophil engraftment time, platelet engraftment time, MNC, aGVHD (grade 0-I vs grade II–IV), and engraftment (). In the multivariable analysis, increasing MNC had an OR of 1.43 for HC (p = 0.002, 95% CI 1.41–1.78), and aGVHD (grade 0–I vs grade II–IV) an OR of 7.75 (p = 0.011, 95% CI 1.59–37.84). MNC and aGVHD were independent prognostic factors for HC. And we performed validation analyses in the MRD, HID and MUD groups. MNC and aGVHD were prognostic factors for HC in the HID group (, MNC: OR: 1.32, 95%CI: 1.07–1.64, p = 0.011; aGVHD: OR: 6.30, 95%CI: 1.53–25.91, p = 0.011). However, MNC and aGVHD were not statistically significant in the MRD group and the MUD group (). We performed ROC analysis to test the specificity and sensitivity of MNC. MNC had a great ability to predict HC (, AUC = 0.65, p = 0.026). When the MNC is above the threshold (8.53×108/kg, sensitivity: 75%, specificity: 58%), the probability of HC occurrence is significantly increased. We further analyzed CI of engraftment and aGVHD. The CI of engraftment in the HC group was higher than that in the no HC group (, 65.4% ± 9.3% vs 46.6% ± 5.9%, p = 0.08). But this difference was not statistically significant (p > 0.05). The CI of aGVHD between HC group and no HC group was significantly different (p < 0.05). Regardless of the CI of grade I-IV aGVHD ((A), HC: 65.4% ± 9.3% vs no HC: 36.6% ± 5.7%, p = 0.018) or grade II-IV aGVHD ((B), HC: 39.5% ± 9.5%% vs no HC: 8.5% ± 3.3%, p < 0.001), the HC group was higher than the no HC group.
Figure 3. Receiver operating characteristic (ROC) curve of MNC for patients with and without HC. The cut off was calculated by the largest Youden's index (74% sensitivity, 58% specificity). The threshold of the MNC level is at 8.53×108/kg.
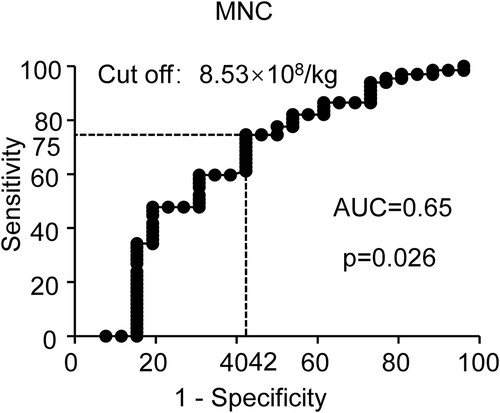
Figure 5. Cumulative incidence of aGVHD and cGVHD in HC and no HC groups. (A) Cumulative incidence of Grade I-IV aGVHD. (B) Cumulative incidence of Grade II-IV aGVHD. (C) Cumulative incidence of cGVHD. (D) Cumulative incidence of moderate and severe cGVHD.
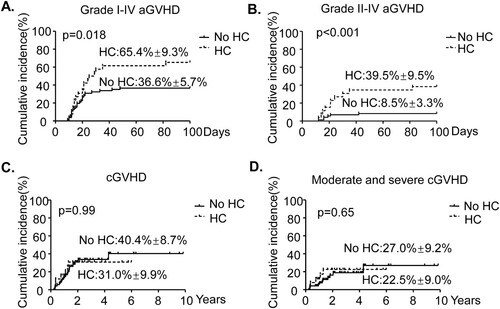
Table 3. Univariate analysis of HC in children with AA receiving HSCT.
Table 4. Multivariate analysis of HC in children with AA receiving HSCT.
Table 5. Validation analysis of HC in children with AA receiving MRD, HID and MUD.
Outcomes and HC
Finally, we explored the relationship between the long-term prognosis after HSCT and HC. All patients did not have disease relapse and late transplantation failure. We compared the difference in the CI of cGVHD ((C), HC: 31.0% ± 9.9% vs No HC 40.4% ± 8.7%, p = 0.99) and CI of moderate and severe cGVHD ((D), HC: 22.5% ± 9.0% vs No HC 27.0% ± 9.2%, p = 0.65) between the HC group and the no HC group. However, HC was not associated with cGVHD. We further compared the survival of the two groups. Overall, 4 patients died, including 3 with HC. In 3/4 cases the death was caused by infections (bacterial, fungal) and aGVHD. In 1/4 cases, the death was caused by encephalitis and bronchiolitis obliterans. Patients without HC had better OS (, No HC: 98.6% ± 1.4% vs HC: 87.4% ± 6.8%, p = 0.03) than with HC.
Discussion
Herein, we reported the incidence of HC after allo-HSCT in children with SAA, and explored the potential risk factors for the development of HC and the prognosis of children with HC. In recent years, more and more children with SAA choose allo-HSCT as a treatment option. Although a good disease-free survival rate has been achieved, multiple complications after allo-HSCT have become an important factor restricting the quality of life. Among these complications, HC is a noteworthy complication particularly in this pediatric population. Therefore, a complete understanding of the risk and outcomes of HC is important. To our knowledge, there are no reports that have analyzed the risk factors of HC after HSCT in children with SAA. In our study, the incidence of clinical signs of HC was 26.8%, which was similar to the incidence of HC as described in the literature [Citation18].
Adequate immune reconstitution post-HSCT is crucial for the success of transplantation, and is associated with many post-HSCT complications [Citation22,Citation23]. Caroline Jepsen et al analyzed 96 patients who underwent haplo-HCT, the medium time to myeloerythroid reconstitution in the whole cohort was 11 days for neutrophils and 14 days for platelets. Among the HC-positive patients, this was 15 (neutrophils) and 14 (platelet) days. The study did not perform a statistical comparison of engraftment time [Citation24]. In our study, we found that the time of neutrophil engraftment and platelet engraftment in children with HC were longer than those without HC (, ). The immune disorder caused by HC may be an important reason for the delay of engraftment. Recovery of NK-, B- and T-lymphocytes is usually slow following HSCT and increases the risk of viral infections [Citation25–27]. And virus infection is an important factor in causing HC [Citation28]. We compared frequency and cell counts of lymphocyte subsets on D30 after HSCT in patients who did and did not develop HC. Somewhat surprisingly, we found no significant difference between these two groups (). In our study, we only analyzed the lymphocyte subsets on D30 after HSCT in the two groups, and the subsequent immune reconstitution was not analyzed. In our cohort, we do not find lymphocyte subsets on D30 playing a major role in developing HC.
Many HC risk factors such as male gender, older age, clinical conditions, and GVHD have been described [Citation29,Citation30]. Hassan et al analyzed 834 patients who underwent HSCT, busulfan-based conditioning, myeloablative conditioning, and CMV infection were found to be risk factors for grades II–IV HC, and bacteremia and severe acute GVHD were the risk factors for severe HC (grades IIIIV) [Citation31]. Barbaros sahin karagun et al. reported patients with a HID, a history of busulfan, and cyclophosphamide treatment or total body irradiation had a higher risk of BK virus-associated HC [Citation32]. In our subject, we identified potential risk factors for HC, and found that VSAA, UCB, MNC, gut aGVHD, and II-IV aGVHD were risk factors in our univariate analysis (). MNC and II-IV aGVHD were significant risk factors in our multivariable analysis (; p = 0.002 and p = 0.011, respectively). Since only UCB used MAC conditioning regimen among all donor types, this may be the reason why UCB is a risk factor for HC. To our knowledge time to VSAA and MNC dose have not been previously reported as risk factors for HC. Transplantation with high MNC dose (, cut off: 8.53 × 108/kg) may further increase the risk of HC after transplantation. Higher numbers of MNCs in grafts may be more likely to cause damage to the bladder epithelium. Therefore, a reasonable dose of MNC is essential for the prevention of HC.
Many studies have shown that there may be a correlation between GVHD and HC or immunosuppressive therapy used to treat GVHD increases the chance of subsequent opportunistic infections of HC [Citation33–35]. However, it still remains not clear whether aGVHD targeting bladder epithelium is manifested as HC or whether immunosuppressive drugs used to treat acute GVHD which could affect viral reactivation. Our study found that regardless of the cumulative incidence of grade I-IV aGVHD or grade II-IV aGVHD, the HC group was significantly higher than the no HC group ((A, B)). However, no significant correlation between HC and the occurrence and severity of cGVHD was found ((C, D)). Regarding overall survival, we found that HC patients were lower than those without HC (, No HC: 98.6%±1.4% vs HC: 87.4%±6.8%, p = 0.03). The patient’s cause of death was GVHD or infection. This may be due to the higher CI of aGVHD in the HC group. The use of stronger immunosuppressive strategies further increased the risk of infection in children [Citation36,Citation37].
Research limitations include its retrospective nature, the small number of samples analyzed, and the small number of HC patients. In the risk factor analyses, we analyzed relatively small patient numbers, higher numbers are needed to achieve a more robust risk analysis. And our center has not tested the BK virus. Prospective multicenter studies are required to firmly establish risk factors for HC development.
In conclusion, we found MNC (cut off: 8.53×108/kg) and II-IV aGVHD were independent risk factors and sufficient for developing HC through univariate and multivariate analysis. And the platelet engraftment in children with HC is late. Children with HC have a worse overall survival than children without HC. Further validation of these findings may help clarify our understanding. Knowledge of the natural history and factors associated with HC is important for the day-to-day management of SAA children undergoing HSCT and the design of clinical trials testing novel therapies.
Supplemental Material
Download TIFF Image (3.7 MB)Supplemental Material
Download TIFF Image (3.8 MB)Supplemental Material
Download TIFF Image (3.8 MB)Disclosure statement
No potential conflict of interest was reported by the author(s).
Correction Statement
This article has been republished with minor changes. These changes do not impact the academic content of the article.
Additional information
Funding
References
- Hartung HD, Olson TS, Bessler M. Acquired aplastic anemia in children. Pediatr Clin North Am. 2013;60:1311–1336.
- Kako S. [Allogeneic hematopoietic stem cell transplantation for patients with aplastic anemia]. Rinsho Ketsueki. 2020;61:1388–1394.
- Korthof ET, Bekassy AN, Hussein AA. Management of acquired aplastic anemia in children. Bone Marrow Transplant. 2013;48:191–195.
- Young NS. Current concepts in the pathophysiology and treatment of aplastic anemia. Hematol Am Soc Hematol Educ Program. 2013;2013:76–81.
- Scheinberg P, Wu CO, Nunez O, et al. Long-term outcome of pediatric patients with severe aplastic anemia treated with antithymocyte globulin and cyclosporine. J Pediatr. 2008;153:814–819.
- Kojima S, Horibe K, Inaba J, et al. Long-term outcome of acquired aplastic anaemia in children: comparison between immunosuppressive therapy and bone marrow transplantation. Br J Haematol. 2000;111:321–328.
- Peslak SA, Olson T, Babushok DV. Diagnosis and treatment of aplastic anemia. Curr Treat Options Oncol. 2017;18:70.
- Esteves I, Bonfim C, Pasquini R, et al. Haploidentical BMT and post-transplant Cy for severe aplastic anemia: a multicenter retrospective study. Bone Marrow Transplant. 2015;50:685–689.
- Kudo K, Muramatsu H, Narita A, et al. Unrelated cord blood transplantation in aplastic anemia: is anti-thymocyte globulin indispensable for conditioning? Bone Marrow Transplant. 2017;52:1659–1661.
- Yagasaki H, Takahashi Y, Hama A, et al. Comparison of matched-sibling donor BMT and unrelated donor BMT in children and adolescent with acquired severe aplastic anemia. Bone Marrow Transplant. 2010;45:1508–1513.
- Leung AY, Mak R, Lie AK, et al. Clinicopathological features and risk factors of clinically overt haemorrhagic cystitis complicating bone marrow transplantation. Bone Marrow Transplant. 2002;29:509–513.
- Harkensee C, Vasdev N, Gennery AR, et al. Prevention and management of BK-virus associated haemorrhagic cystitis in children following haematopoietic stem cell transplantation–a systematic review and evidence-based guidance for clinical management. Br J Haematol. 2008;142:717–731.
- Keles I, Bozkurt MF, Cemek M, et al. Prevention of cyclophosphamide-induced hemorrhagic cystitis by resveratrol: a comparative experimental study with mesna. Int Urol Nephrol. 2014;46:2301–2310.
- Droller MJ, Saral R, Santos G. Prevention of cyclophosphamide-induced hemorrhagic cystitis. Urology. 1982;20:256–258.
- Shepherd JD, Pringle LE, Barnett MJ, et al. Mesna versus hyperhydration for the prevention of cyclophosphamide-induced hemorrhagic cystitis in bone marrow transplantation. J Clin Oncol. 1991;9:2016–2020.
- Fu H, Xu L, Liu D, et al. Late-onset hemorrhagic cystitis after haploidentical hematopoietic stem cell transplantation in patients with advanced leukemia: differences in ATG dosage are key. Int J Hematol. 2013;98:89–95.
- Xu LP, Zhang HY, Huang XJ, et al. Hemorrhagic cystitis following hematopoietic stem cell transplantation: incidence, risk factors and association with CMV reactivation and graft-versus-host disease. Chin Med J (Engl). 2007;120:1666–1671.
- Decker DB, Karam JA, Wilcox DT. Pediatric hemorrhagic cystitis. J Pediatr Urol. 2009;5:254–264.
- Glucksberg H, Storb R, Fefer A, et al. Clinical manifestations of graft-versus-host disease in human recipients of marrow from HL-A-matched sibling donors. Transplantation. 1974;18:295–304.
- Jagasia MH, Greinix HT, Arora M, et al. National institutes of health consensus development project on criteria for clinical trials in chronic graft-versus-host disease: I. The 2014 diagnosis and staging working group report. Biol Blood Marrow Transplant. 2015;21:389–401 e381.
- Edenfield WJ, Moores LK, Goodwin G, et al. An engraftment syndrome in autologous stem cell transplantation related to mononuclear cell dose. Bone Marrow Transplant. 2000;25:405–409.
- Elfeky R, Lazareva A, Qasim W, et al. Immune reconstitution following hematopoietic stem cell transplantation using different stem cell sources. Expert Rev Clin Immunol. 2019;15:735–751.
- Chang YJ, Zhao XY, Huang XJ. Immune reconstitution after haploidentical hematopoietic stem cell transplantation. Biol Blood Marrow Transplant. 2014;20:440–449.
- Jepsen C, Turkiewicz D, Ifversen M, et al. Low incidence of hemorrhagic cystitis following ex vivo T-cell depleted haploidentical hematopoietic cell transplantation in children. Bone Marrow Transplant. 2020;55:207–214.
- Annaloro C, Serpenti F, Saporiti G, et al. Viral infections in HSCT: detection, monitoring, Clinical management, and immunologic implications. Front Immunol. 2020;11:569381.
- Atilla E, Atilla PA, Bozdag SC, et al. A review of infectious complications after haploidentical hematopoietic stem cell transplantations. Infection. 2017;45:403–411.
- Sahin U, Toprak SK, Atilla PA, et al. An overview of infectious complications after allogeneic hematopoietic stem cell transplantation. J Infect Chemother. 2016;22:505–514.
- Dosin G, Aoun F, El Rassy E, et al. Viral-induced hemorrhagic cystitis after allogeneic hematopoietic stem cell transplant. Clin Lymphoma Myeloma Leuk. 2017;17:438–442.
- Lunde LE, Dasaraju S, Cao Q, et al. Hemorrhagic cystitis after allogeneic hematopoietic cell transplantation: risk factors, graft source and survival. Bone Marrow Transplant. 2015;50:1432–1437.
- Lam W, Storek J, Li H, et al. Incidence and risk factor of hemorrhagic cystitis after allogeneic transplantation with fludarabine, busulfan, and anti-thymocyte globulin myeloablative conditioning. Transpl Infect Dis. 2017;19:12677.
- Hassan Z, Remberger M, Svenberg P, et al. Hemorrhagic cystitis: a retrospective single-center survey. Clin Transplant. 2007;21:659–667.
- Karagun BS, Aygunes U, Eken A, et al. Risk assessment for BK virus-associated hemorrhagic cystitis after pediatric hematopoietic stem cell transplant: A single-center retrospective cross-sectional study. Exp Clin Transplant. 2021.
- Ost L, Lonnqvist B, Eriksson L, et al. Hemorrhagic cystitis–a manifestation of graft versus host disease? Bone Marrow Transplant. 1987;2:19–25.
- Echavarria M, Herrera F, Solimano J, et al. Cyclic recovery of adenovirus in a stem cell transplant recipient: an inverse association with graft-versus-host disease. Bone Marrow Transplant. 2003;31:301–303.
- Ruutu T, Ruutu M, Volin L, et al. Severe cystitis as a manifestation of chronic graft-versus-host disease after bone marrow transplantation. Br J Urol. 1988;62:612–613.
- Sawinski D, Goral S. BK virus infection: an update on diagnosis and treatment. Nephrol Dial Transplant. 2015;30:209–217.
- Ambalathingal GR, Francis RS, Smyth MJ, et al. BK polyomavirus: clinical aspects, immune regulation, and emerging therapies. Clin Microbiol Rev. 2017;30:503–528.