ABSTRACT
Background
Besides the traditional roles of HBA1 and HBB, recent findings suggest that hemoglobin genes may have roles in other contexts.
Objective
In the present study, we aim to investigate a possible tumor-suppressor role of HBA1 and HBB in acute myeloid leukemia.
Methods
Quantitative real-time PCR (RT-qPCR) was performed to detect the expression levels of HBA1 and HBB in acute myeloid leukemia patients and AML cell lines. The transfected cells were analyzed for Cell Counting Kit-8 (CCK-8), apoptosis, and cell cycle assay.
Results
HBA1 and HBB genes were significantly decreased in acute myeloid leukemia patients and AML cell lines. Furthermore, in vitro approaches showed that overexpression of HBA1 and HBB inhibited proliferation, induced cell apoptosis, and blocked cell cycle process at the G2/M phase in K562 cells.
Conclusion
Our data indicated that HBA1 and HBB genes may be potential tumor-suppressor genes in acute myeloid leukemia.
Introduction
The development of multicellular organisms in adulthood are achieved by complex control of basic cellular processes including maintenance of pluripotent stem cells, cell fate decision, differentiation, proliferation, and cell death [Citation1]. From germ cells, stem cells, until terminal differentiation, there is a gradual increase in differentiation degree and decrease in proliferation capacity. Abnormality of this developmental pattern may lead to the occurrence of tumors. Tumor cells are biologically characterized by stagnation of differentiation and infinite proliferation. There is a negative correlation between differentiation degree and proliferation ability.
Erythropoiesis is a process that hematopoietic stem cells (HSCs) differentiate into erythrocytes, including early erythropoiesis and terminal erythroid differentiation [Citation2]. Primitive erythroblasts enucleate to become reticulocytes, which subsequently mature into red blood cells [Citation3,Citation4]. Adult red blood cells, as the terminal differential cells, with no nucleus and rather simple structure, have ceased dividing and have no proliferation capacity [Citation5]. The main feature of red blood cells is a high level of adult hemoglobin, with hemoglobin A (HbA) accounting for 97% of its weight, which indicates that high expression of HbA may be accompanied by the cessation of cell proliferation. Human erythroleukemia K562 cells can proliferate indefinitely, but don’t express HbA. Does this mean that no expression of HbA may be accompanied by unlimited proliferation? HBB and HBA1 are genes that encode the normal adult hemoglobin tetramer (Hb) [Citation6]. Adult hemoglobin (HbA) is the most popular form of hemoglobin including two β-globin molecules and two α-globin molecules [Citation7]. Therefore, we hypothesized that HBB and HBA1 may be associated with differentiation degree and proliferation ability of blood cells.
Human HBB gene is mapped to chromosome region11p15.5 and human HBA1 gene is located at chromosome region16p13.3 [Citation8]. Interestingly, both chromosome region 11p15.5 and 16p13.3 have a high incidence of loss of heterozygosity (LOH) [Citation9,Citation10]. Nonrandom chromosome deletion and allele loss often indicate inactivation of tumor-suppressor genes (TSGs) residing in a particular chromosomal region [Citation11]. Thus, we speculate that whether HBA1 as well as HBB have tumor-suppressive function during the growth and development of blood cells. Here, we aim to evaluate the expression levels of HBB and HBA1 in acute myeloid leukemia (AML) and then to explore the effects of HBB and HBA1 on the proliferation ability of K562 cells.
Materials and methods
Patients and samples
A total of 78 (51 men and 27 women, median age 62, 16–85) whole peripheral blood samples from new diagnosed AML patients were collected from January 2020 and June 2021 at Zhongnan Hospital of Wuhan University. The simultaneous 80 healthy peripheral blood samples (48 males and 32 females, median age 60, 14–91) were collected for the control group. All of the diagnoses were made according to the WHO 2016 classification. All AML patients were analyzed at diagnosis before any treatment.
RNA extraction and real-time polymerase chain reaction
Total RNA was extracted from blood mononuclear cells with Trizol reagent (Takara, Japan) according to the manufacturer’s protocol. The cDNAs from total RNA were synthesized using PrimeScript RT reagent Kit (Takara, Japan). Real-Time PCR was performed on ABI 7700 (Applied Biosystems, Foster City, CA, U.S.A.) using qPCR Mastermix for SYBR Green I (Eurogenetec, Seraing, Belgium) following manufacturer’s protocol. The reaction was carried out in a volume of 25 μL containing 2 μL cDNA, 12.5 μL SYBR Green mix, 2 μL gene-specific forward and reverse primers (0.1 μM), and 8.5 μL nuclease-free water. GAPDH was applied as the internal control housekeeping gene for normalization. The primers for the reaction were as follows: HAA sense: 5′-CGACAAGACCAACGTCAAGG-3′ and antisense: 5′-GTGGGGAAGGACAGGAACAT-3′; HBB sense: 5′-CACGTGGATCCTGAGAACTTCAG-3′ and antisense: 5′-GGTGAATTCTTTGCCAAAGTGAT-3′; GAPDH sense: 5′-GAAGGTGAAGGTCGGAGTC-3′ and antisense: 5′-GAAGATGGTGATGGGATTTC-3′. The amplifications started at 95°C for 5 min followed by 35 cycles, each consisting of denaturing for 30 s at 95°C, annealing for 30 s at 60°C, and elongation for 30 s at 72°C. All reactions were amplified in duplicate with no-template controls included. Relative expression of the mRNA was calculated by 2−ΔΔCt method and normalized to GAPDH.
Plasmid construction
We used the pEGFP-C2 to subclone the HBA1 and HBB sequence for the transfection studies. The cDNA was amplified by PCR with the following primers: HBA1 forward, 5′-CTCGGTGGCCATGCTTCTT-3′, reverse, 5′-GCCGCCCACTCAGACTTTAT-3′, and HBB forward: 5′-ATGGTGCATCTGACTCCTGAGG-3′, Reverse: 5′-GTGATACTTGTGGGCCAGGGC-3′. The PCR productions were digested with endonuclease KpnI and SacI, and ligated with the vector pEGFP-C2 by T4 DNA ligase. Afterwards, the reconstructed plasmid was validated by DNA sequencing.
Cell culture and plasmid transfection
Kasumi, Mv-411, HL-60, K562, and 293T cells were purchased from China Center for Type Culture Collection (Wuhan, China) and cultured in RPMI 1640 (Gibco, U.S.A.) supplemented with 10% fetal bovine serum, penicillin/streptomycin (100 U/mL and 100 µg/mL, respectively) at 37°C in a humidified atmosphere of 5% CO2. K562 cells in log phase were transfected with Lipofectamine 2000 (Invitrogen) according to the manufacturer’s instructions. After 48 h of transfection, cells are subjected to the follow-up experiment. All the cell function experiments were performed in triplicate.
Cell proliferation assay
Cell proliferation assays were conducted using the Cell Counting Kit-8 (CCK-8) (Dojindo, Japan) according to the manufacturer’s instructions. In brief, 150 µL cells were seeded into 96-well plates (6.8 × 103/ml) and cultured in 5% CO2 at 37°C. Every 24 h for seven successive days, 10 µl CCK8 solution were to each well and cultured for additional 2 h. Then, 100 µL cell suspensions were seeded in new 96-well plates and measured using a 450 nm spectrophotometer (EnSpire, PerkinElmer, U.S.A.).
Cell apoptosis assay
Cells in log phase were washed two times with PBS and collected. Suspended cells in binding buffer at a density of 5 × 105/mL were mixed with Annexin V/APC (BD biosciences, San Jose, CA, U.S.A.), and then incubated for 10 min at room temperature. After centrifugation and washed once with PBS, re-suspended cells were stained with propidium iodide (PI, 1 µg/mL, Sigma) for 30 min at 4°C in the dark and then analyzed by flow cytometry.
Cell cycle analysis
Cells in log phase were seeded in six-well plates at a density of 1 × 106/mL. Cells were fixed in paraformaldehyde for 60 min at room temperature and then fixed in 70% ethanol at 4°C over night. Next, cells were collected and washed once with PBS. One hundred microliters RNase A (100 µg/mL) were added, and cells were stained with propidium iodide (PI, 50 µg/mL) for 30 min at 4°C in the dark and then detected by flow cytometry.
Statistical analysis
Statistical analyses were performed using SPSS24.0 statistical software (SPSS Inc., U.S.A.). Normally distributed data are presented as mean ± standard error of mean (M ± S.E.M.). Shapiro–Wilk test was used to test the normality of each data set. Normally distributed data sets were analyzed by Student’s t-tests, while non-normally distributed data were analyzed by Kruskal–Wallis variance analyses. P-value less than .05 was considered to be statistical significance.
Results
Decreased expression of HBA1 and HBB in AML
RT-qPCR was used to detect the expression levels of HBA1 and HBB in AML patients and normal control. In normal controls, the relative average expressions of HBA1 and HBB were 3.71 and 11.60, respectively. In AML patients, their relative average expressions were 1.33 and 7.89, respectively. Our results revealed that the relative expression levels of HBA1 and HBB were significantly decreased in AML patients compared with normal control patients. (HBA1: P < .001, (A); HBB: P < .001, (B)).
Figure 1. HBA1 and HBB in leukemia patients and normal control. The relative HBA1 and HBB expression was determined by RT-qPCR. (A) Levels of HBA1 in leukemia patients are significantly lower than that in normal control. (B) Levels of HBB in leukemia patients are significantly lower than that in normal control, **P < .01.
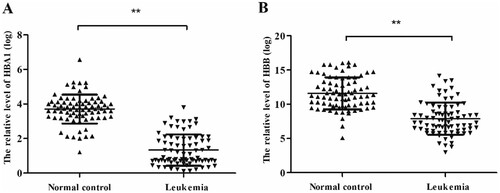
HBA1 and HBB in AML cell lines
Next, we detected the expressions of HBA1 and HBB in several cell lines (Kasumi, Mv-411, HL-60, K562, and 293T). We found that both HBA1 and HBB expressions were significantly lower in AML cell lines (Kasumi, Mv-411, HL-60, and K562) than human embryonic kidney cell 293T cell line () (P < .05).
Overexpression of HBA1 and HBB in K562 cells inhibited cell proliferation
In order to clarify the effects of HBA 1 and HBB in cell growth, cell growth assay was performed. The CCK8 assay showed that overexpression of HBA1 and HBB in K562 cells significantly inhibited their proliferation. The difference of cell growth between overexpressed HBA1 and HBB cells and control reached statistical significance on the seventh day () (P < .05).
Overexpression of HBA1 and HBB promoted apoptosis in K562 cells
To quantify the effects of HBA1 and HBB on apoptosis in K562 cells, Annexin-V and PI staining assays were conducted. Cells in Lower Right (LR) and Upper Right (UR) were considered to be early apoptotic (Annexin + /PI-) and late apoptotic (Annexin + /PI +) respectively. Cells in Lower Left (LL) and Upper Left (UL) quadrants were considered to be alive and necrotic, respectively. The sum percentages of LR and UR quadrants indicated the degree of apoptosis. The percentages of apoptotic cells transfected with HBA1 were higher than those of control cells (20.60% ± 0.82 vs. 9.55% ± 0.52, P < .01) and the percentages of apoptotic cells transfected with HBB were also higher than control cells (29.36% ± 0.73 vs. 9.55% ± 0.52, P < .01), which suggested that overexpression of HBA1 and HBB promoted apoptosis in K562 cells ().
Overexpression of HBA1 and HBB induce cell cycle arrest at G2/M phase
In order to study DNA cell content changes in cell cycle, cell cycle analysis was performed by PI single labeling. While cells transfected with HBA1 and HBB progressed through different phases of cell cycle, cells transfected with HBA1 and HBB displayed higher frequency of cells at the G2/M phases (pEGFP-C2-HBA1: 34.16% ± 2.07% vs. 23.58% ± 1.93%; pEGFP-C2-HBB: 31.25% ± 0.88% vs. 23.58% ± 1.93%, P < .05) than control. The results indicated that both of HBA1 and HBB were able to induce cell cycle arrest at G2/M phase ().
Discussion
HBA1 and HBB are well-known genes that are responsible for oxygen transporter activity [Citation12,Citation13]. It is well studied that deletion of one or more of the duplicated HBA genes usually causes α-thalassemia and point mutations that cripple or completely inactivate expression from the HBB gene lead to β thalassemia [Citation14–16]. However, rare attention has been paid to the field of cancer. In the present study, we found that HBA1 and HBB genes were significantly decreased in acute myeloid leukemia patients and AML cell lines. Then we constructed the expression vectors of HBA1 and HBB cDNA and transfected into human K562 erythroleukemia cells. Our results indicated that exogenous expression of HBA1 and HBB suppressed K562 cell growth significantly. Consistent with their role of growth inhibition, HBA1 and HBB were important factors regulating the cell cycle process. Overexpressed HBA1 and HBB blocked cell cycle at the G2/M phase and prevented cells entering the S phase, which reduced cellular division and proliferation velocities. In addition, the percentages of apoptotic cells transfected with HBA1 and HBB increased significantly, which suggests that they may be involved in cell apoptosis induction. Therefore, it seems likely that HBA1 and HBB play important roles in cell proliferation and differentiation. This is the first report that revealed the tumor suppress role of HBA1 and HBB in AML.
It has been reported that erythroid cell maturation and hemoglobin production are inseparable [Citation17–19]. In the process of RBC maturation, the degree of cell differentiation and hemoglobin content become higher and higher, but the ability of cell proliferation is decreased. The results indicated that hemoglobin content may be positively correlated with the degree of cell differentiation, but negatively correlated with the ability of cell proliferation. Low hemoglobin levels in various types of cancer patients have been universally found [Citation20]. Despite the fact that cancer-associated anemia can be caused by inflammation, iron malabsorption, and nutritional status of cancer patients [Citation21–23], the low expression of hemoglobin due to the inactivation of hemoglobin genes may also be an important factor. Oxidative stress plays an important role in the occurrence and development of leukemia and is closely related to the treatment and prognosis of leukemia [Citation24]. We know that intracellular oxygen is provided by free diffusion through membranes, but intracellular HBA and HBB could affect the intracellular pO2 by binding oxygen, thus rendering it unavailable for the cell sensors [Citation25]. Thus, the hypothesis that HBA1 and HBB may inhibit the progress of cancer through oxidative stress was made. However, the exact mechanisms need to be further researched.
Genetically, HBB locates at 11p15.5 and this locus contains embryonic and adult beta-like globin genes that are ordered as they are expressed during development [Citation26]. At this locus, the centromeric part of chromosome segment 11p15.5 contains a region of frequent allele loss in many solid malignancies [Citation27]. The decreased expression of human SRBC gene (hSRBC), which maps to chromosome region 11p15.5-p15.4, was reported both in mRNA and protein levels in breast and lung cancer cell lines, with retained hSRBC expression in normal epithelial cultures from these organs [Citation28]. TRIM3 gene, a gene that also located at 11p15.5, is markedly reduced in human glioblastoma samples, neurosphere cultures, and cell lines and its reconstitution impairs growth properties in vitro and in vivo [Citation29]. Elizabeth B. Claus et al. identified a new susceptibility locus for meningioma at 11p15.5 using genome-wide association analysis identifies a meningioma [Citation30]. Considering these results, it is highly suggested that novel tumor-suppressor genes might exist at this locus.
In our study, we found a possible tumor-suppressive role of HBA1 and HBB in AML. In agreement with our study, a study by Onda showed that the expression of HBB gene was down-regulated in anaplastic thyroid cancer patients and exogenous expression of HBB could suppress cell proliferation in thyroid cancer cell lines [Citation31]. Another study reported that HBB could suppress neuroblastoma growth and metastasis with potential utility as an anticancer drug and a biomarker signaling the presence of clinically undetectable metastasis [Citation32]. However, Marco Ponzetti and his colleagues found that the expression of HBB was significantly higher in invasive carcinoma histotypes compared to in situ counterparts and HBB-overexpressing breast cancer cells migrate and invade more. Using bioinformatics analyses of public data sets, they also concluded that high HBB expression was correlated with patients’ lower overall survival [Citation33]. We therefore deemed that HBB may play a diverse role in different tumors.
In summary, the results of our investigations showed inactivation of hemoglobin genes in AML and indicated that up-regulation of HBA1 and HBB expression can effectively inhibit the proliferation of K562 cells, induce cell apoptosis, and block cell cycle process, thus provided evidence suggesting the possibility of HBA1 and HBB as novel tumor-suppressor genes in leukemia. A systematic study of its expression in leukemia patients and the analysis of the correlation of HBA1 and HBB with pathological events, such as relapse and recurrence, could be of great interest. Further investigations are still essential to explore the exact molecular mechanism of HBA1 and HBB in leukemia.
Ethics approval
The study protocol was approved by the Medical Ethical Committee of Zhongnan Hospital of Wuhan University (ethical approval 2020010, January 2022).
Consent to participate
Informed consent was obtained from all individual participants included in the study.
Disclosure statement
No potential conflict of interest was reported by the author(s).
Additional information
Funding
References
- Stevanovic M, Drakulic D, Lazic A, et al. SOX transcription factors as important regulators of neuronal and glial differentiation during nervous system development and adult neurogenesis. Front Mol Neurosci. 2021;14:654031. DOI:10.3389/fnmol.2021.654031
- Zhang J, Zhao H, Wu K, et al. Knockdown of spliceosome U2AF1 significantly inhibits the development of human erythroid cells. J Cell Mol Med. 2019;23(8):5076–5086. DOI:10.1111/jcmm.14370
- Hattangadi SM, Wong P, Zhang L, et al. From stem cell to red cell: regulation of erythropoiesis at multiple levels by multiple proteins, RNAs, and chromatin modifications. Blood. 2011;118(24):6258–6268. DOI:10.1182/blood-2011-07-356006
- Dzierzak E, Philipsen S. Erythropoiesis: development and differentiation. Cold Spring Harb Perspect Med. 2013;3(4):a011601, DOI:10.1101/cshperspect.a011601
- Barminko J, Reinholt B, Baron MH. Development and differentiation of the erythroid lineage in mammals. Dev Comp Immunol. 2016;58:18–29. DOI:10.1016/j.dci.2015.12.012
- Rafiei J, Yavari K, Moosavi-Movahedi AA. Preferential role of iron in heme degradation of hemoglobin upon gamma irradiation. Int J Biol Macromol. 2017;103:1087–1095. DOI:10.1016/j.ijbiomac.2017.05.153
- Aldakeel SA, Ghanem NZ, Al-Amodi AM, et al. Identification of seven novel variants in the β-globin gene in transfusion-dependent and normal patients. Arch Med Sci. 2020;16(2):453–459. DOI:10.5114/aoms.2019.84825
- Harteveld CL, Voskamp A, Phylipsen M, et al. Nine unknown rearrangements in 16p13.3 and 11p15.4 causing alpha- and beta-thalassaemia characterised by high resolution multiplex ligation-dependent probe amplification. J Med Genet. 2005;42(12):922–931. DOI:10.1136/jmg.2005.033597
- Chitragar S, Iyer VK, Agarwala S, et al. Loss of heterozygosity on chromosome 11p15.5 and relapse in hepatoblastomas. Eur J Pediatr Surg. 2011;21(1):50–53. DOI:10.1055/s-0030-1267208
- So CK, Nie Y, Song Y, et al. Loss of heterozygosity and internal tandem duplication mutations of the CBP gene are frequent events in human esophageal squamous cell carcinoma. Clin Cancer Res. 2004;10(1 Pt 1):19–27. DOI:10.1158/1078-0432.ccr-03-0160
- Ohshima K, Fujiya K, Nagashima T, et al. Driver gene alterations and activated signaling pathways toward malignant progression of gastrointestinal stromal tumors. Cancer Sci. 2019;110(12):3821–3833. DOI:10.1111/cas.14202
- Vanni S, Zattoni M, Moda F, et al. Hemoglobin mRNA changes in the frontal cortex of patients with neurodegenerative diseases. Front Neurosci. 2018;12:8, DOI:10.3389/fnins.2018.00008
- Pavani G, Fabiano A, Laurent M, et al. Correction of β-thalassemia by CRISPR/Cas9 editing of the α-globin locus in human hematopoietic stem cells. Blood Adv. 2021;5(5):1137–1153. DOI:10.1182/bloodadvances.2020001996
- Zhao J, Li J, Lai Q, et al. Combined use of gap-PCR and next-generation sequencing improves thalassaemia carrier screening among premarital adults in China. J Clin Pathol. 2020;73(8):488–492. DOI:10.1136/jclinpath-2019-206339
- Xu L, Mao A, Liu H, et al. Long-molecule sequencing: a new approach for identification of clinically significant DNA variants in α-thalassemia and β-thalassemia carriers. J Mol Diagn. 2020;22(8):1087–1095. DOI:10.1016/j.jmoldx.2020.05.004
- Sabath DE, Bender MA, Sankaran VG, et al. Characterization of deletions of the HBA and HBB loci by array comparative genomic hybridization. J Mol Diagn. 2016;18(1):92–99. DOI:10.1016/j.jmoldx.2015.07.011
- Ghosh A, Garee G, Sweeny EA, et al. Hsp90 chaperones hemoglobin maturation in erythroid and nonerythroid cells. Proc Natl Acad Sci U S A. 2018;115(6):E1117–e1126. DOI:10.1073/pnas.1717993115
- Cantú I, Philipsen S. Flicking the switch: adult hemoglobin expression in erythroid cells derived from cord blood and human induced pluripotent stem cells. Haematologica. 2014;99(11):1647–1649. DOI:10.3324/haematol.2014.116483
- Khandros E, Weiss MJ. Protein quality control during erythropoiesis and hemoglobin synthesis. Hematol Oncol Clin North Am. 2010;24(6):1071–1088. DOI:10.1016/j.hoc.2010.08.013
- Tchekmedyian NS. Anemia in cancer patients: significance, epidemiology, and current therapy. Oncology. 2002;16(9 Suppl 10):17–24.
- Macciò A, Madeddu C, Gramignano G, et al. The role of inflammation, iron, and nutritional status in cancer-related anemia: results of a large, prospective, observational study. Haematologica. 2015;100(1):124–132. DOI:10.3324/haematol.2014.112813
- Gilreath JA, Rodgers GM. How I treat cancer-associated anemia. Blood. 2020;136(7):801–813. DOI:10.1182/blood.2019004017
- Means RT, Krantz SB. Progress in understanding the pathogenesis of the anemia of chronic disease. Blood. 1992;80(7):1639–1647.
- Dong C, Zhang NJ, Zhang LJ. Oxidative stress in leukemia and antioxidant treatment. Chin Med J. 2021;134(16):1897–1907. DOI:10.1097/cm9.0000000000001628
- Hirota K, Semenza GL. Regulation of angiogenesis by hypoxia-inducible factor 1. Crit Rev Oncol Hematol. 2006;59(1):15–26. DOI:10.1016/j.critrevonc.2005.12.003
- Chang JG, Tsai WC, Chong IW, et al. {Beta}-thalassemia major evolution from {beta}-thalassemia minor is associated with paternal uniparental isodisomy of chromosome 11p15. Haematologica. 2008;93(6):913–916. DOI:10.3324/haematol.12195
- Qin LX. Chromosomal aberrations related to metastasis of human solid tumors. World J Gastroenterol. 2002;8(5):769–776. DOI:10.3748/wjg.v8.i5.769
- Xu XL, Wu LC, Du F, et al. Inactivation of human SRBC, located within the 11p15.5-p15.4 tumor suppressor region, in breast and lung cancers. Cancer Res. 2001;61(21):7943–7949.
- Chen G, Kong J, Tucker-Burden C, et al. Human Brat ortholog TRIM3 is a tumor suppressor that regulates asymmetric cell division in glioblastoma. Cancer Res. 2014;74(16):4536–4548. DOI:10.1158/0008-5472.Can-13-3703
- Claus EB, Cornish AJ, Broderick P, et al. Genome-wide association analysis identifies a meningioma risk locus at 11p15.5. Neuro Oncol. 2018;20(11):1485–1493. DOI:10.1093/neuonc/noy077
- Onda M, Akaishi J, Asaka S, et al. Decreased expression of haemoglobin beta (HBB) gene in anaplastic thyroid cancer and recovery of its expression inhibits cell growth. Br J Cancer. 2005;92(12):2216–2224. DOI:10.1038/sj.bjc.6602634
- Maman S, Sagi-Assif O, Yuan W, et al. The beta subunit of hemoglobin (HBB2/HBB) suppresses neuroblastoma growth and metastasis. Cancer Res. 2017;77(1):14–26. DOI:10.1158/0008-5472.Can-15-2929
- Ponzetti M, Capulli M, Angelucci A, et al. Non-conventional role of haemoglobin beta in breast malignancy. Br J Cancer. 2017;117(7):994–1006. DOI:10.1038/bjc.2017.247