ABSTRACT
Objective
The complex pathogenesis of relapsed and refractory (R/R) immune thrombocytopenia (ITP) contributes to the varied efficacy and tolerability of current treatment regimens. Rapamycin, an immunomodulatory agent, was originally used in the prevention of organ rejection after organ transplantation. Additional evidence now shows that rapamycin can successfully treat R/R ITP. Here, we summarize recent clinical progress on the role and potential mechanism of rapamycin in the treatment of ITP.
Methods
PubMed, Web of Science and CNKI database were searched to identify eligible studies, and the clinical data and preclinical studies on the use of mTOR inhibitors in ITP treatment were reviewed. The key results (efficacy and safety) of the most recent clinical reports were summarized.
Results summarized
Case series provide evidence of the effectiveness and tolerable safety profile of rapamycin in ITP, including primary and some secondary ITP. Mechanistic explorations indicate that rapamycin can regulate immune cell subsets (Th1, Th2, Th17, Treg, Breg, MDSC, etc.), modulate cytokine secretion (IL-6, IL-10, TGF-β, BAFF, etc.) and promote platelet autophagy.
Conclusions
Emerging clinical data and basic studies suggest that rapamycin, as a multifaceted regulator, could provide a new promising option for the therapy of ITP. Additional research is needed to identify those patients which may benefit the most, as well as therapeutic regimens with which rapamycin may be combined.
Introduction
Immune thrombocytopenia (ITP) is an acquired autoimmune disease involving multiple mechanisms. ITP is classified according to etiology as primary immune thrombocytopenia (pITP) with autoimmune disorder of unknown cause or immune thrombocytopenia that develops in the context of other disorders, known as secondary thrombocytopenia (sITP) [Citation1]. Secondary ITP is caused by multiple complicating factors or diseases, including drug induction or/and autoimmune diseases induced thrombocytopenia, such as systemic lupus erythematosus (SLE), antiphospholipid antibody syndrome (APS), autoimmune diseases (AITD), Evans syndrome (ES), lymphocyte proliferative disease and chronic infection (helicobacter pylori, human immunodeficiency virus or hepatitis C virus) [Citation2,Citation3]. In addition, people who receive vaccines, for example, measles-mumps-rubella vaccines and COVID-19 vaccines, may develop thrombocytopenia [Citation4].
So far, first-line treatment of ITP is relegated to the category of glucocorticoids, with subsequent lines of therapy including thrombopoietin receptor agonists (TPO-RAs), rituximab, splenectomy, danazol, vincristine, cyclosporin, mycophenolate mofetil, etc. [Citation5]. The definition of refractory ITP currently is still controversial. ‘Refractory’ was initially defined as failure or relapse after splenectomy [Citation2]. However, applications of new drugs increased the reluctance to undergo or recommend splenectomy among patients and physician, respectively [Citation6]. Therefore, here we define ‘refractory’ as patients who are unresponsive to one or more single-agent therapies, without reference to splenectomy [Citation7–9]. Although multiple lines of drugs can be selected to treat R/R ITP, there is no agreed-upon standard of care.
Additionally, long-term corticosteroids exposure brings serious side effects to patients, including hypertension, hyperglycemia, water and sodium storage, mucosal ulcer, osteoporosis and even femoral head necrosis [Citation10]. Treatment with rituximab (anti-CD20 monoclonal antibody) has a 50% response rate in R/R ITP patients, but with a slow time to response onset and limited long-term efficacy. In addition, chronic use of CD20 mAb may increase the probability of viral infections [Citation3,Citation11]. TPO-RAs are another choice, but long-term use also brings potential risks, such as myelofibrosis [Citation12–14] and cataracts [Citation13,Citation15]. Also, low-to-middle-income countries (LMIC) often have difficulty obtaining TPO-RAs, further reducing widespread application. Taken together, the question begs: are there other drugs with favorable benefit/risk profiles that can be used for the treatment of R/R ITP?
Rapamycin, also named sirolimus, is a macrolide immunosuppressant isolated from a bacterium on Rapa Nui Island in the 1960s and was first studied as a low-toxicity antifungal drug. Later studies found that rapamycin specifically targets a protein kinase in mammals, which was named mammalian target of rapamycin, mTOR. mTOR is a conserved 289KD serine-threonine protein kinase, and a member of the PI3K kinase family, which is also a key enzyme in the cell signaling pathway that regulates cell survival, proliferation and differentiation [Citation16–18]. mTOR exists mainly in the form of two groups of complexes, mammalian target of rapamycin complex 1 (mTORC1) and mTORC2, which are closely related in structure but distinct in function. mTORC1 depends on the phosphorylation of various downstream protein molecules and controls a series of basic cell biological processes, such as cell growth, metabolism and autophagy [Citation19,Citation20]. mTORC2 has been characterized to mainly control cell size and cell death pathways [Citation21]. Rapamycin can inhibit mTOR complex activity, from which is derived the term ‘mTOR inhibitor’ (mTORi). Researchers discovered that rapamycin has immunosuppressive effects, and subjected it to clinical exploration as a new drug for the treatment of organ transplant rejection in the 1980s [Citation22,Citation23]. Recently, increasing reports highlight the use of mTORi for immune hematological diseases, including ITP [Citation24–27]. PubMed, Web of Science and CNKI database were searched to identify eligible studies and the clinical data and preclinical studies on the use of mTOR inhibitors in ITP treatment were reviewed.
Efficacy of rapamycin in the treatment of primary immune thrombocytopenia
Immune thrombocytopenia is highly heterogeneous, resulting in variable second-line therapy for relapsed and refractory ITP. Some patients, even after receiving some drugs mentioned above, still have poor effects, which requires doctors to find newer drugs or re-explore older treatments like mTOR inhibitor, previously for GVHD prophylaxis and treatment. Based on the pharmacological characteristics of rapamycin, increasing clinical evidence demonstrated the effectiveness of mTORi in the treatment of ITP, both pITP and sITP.
Li et al. [Citation28] conducted a randomized trial to investigate the efficacy and safety of rapamycin combined low dose prednisone treatment in adults with chronic ITP. The overall response rate (ORR) was 58%, similar to that of patients treated with cyclosporine A plus prednisone (p = 0.70). However, sustained response (SR) was more pronounced in the rapamycin group (68% vs. 39%, p < 0.05). The median treatment time was 8.48 months (7–10 months) and the median onset time was 1.96 months in the rapamycin group. Both groups showed a similar incidence of adverse events (7% vs. 11%), including mild gastrointestinal reaction, secondary hypertension and respiratory infection. Jasinski et al. [Citation29] used rapamycin to treat 12 ITP patients, in which they observed an OR rate of 83% (10/12) and 75% of patients achieved complete response (CR). Our center initiated a prospective clinical trial using rapamycin to treat R/R ITP patients and evaluated the efficacy every 3 months [Citation24]. By the end of the third month, 40% of patients achieved complete response and 45% of patients achieved partial response (PR), whereby establishing an ORR of 85%. By 6 months of treatment, ORR decreased to 70% without serious side effects. After 12 months of follow-up, the ORR remained at 65%. Oral ulcers and mild hyperlipidemia were the most common adverse events in this study. Li et al. [Citation30] found that, in R/R ITP patients treated with rapamycin for 6–18 months, the ORR at 12 months was 73.7% and CR was 52.6%, indicating that the longer period of administration of rapamycin, the better the sustained effect in responders. Mousavi et al. [Citation31] compared rapamycin and cyclosporine for the treatment of pediatric chronic ITP and reported that rapamycin had similar rate of response with cyclosporine; moreover, rapamycin had a better safety profile. Recently, it is found that calcitriol can enhance the efficacy of rapamycin in ITP; the OR rate of the two-drug regimen increased from 64.4% to 79.5% [Citation32]. Importantly, none of the above studies have reported any serious adverse events of rapamycin, consistent with the well-established safety profile of mTORi. Taken together, these studies support the use of rapamycin as a steroid-sparing agent for R/R ITP ().
Table 1. Rapamycin in the treatment of immune thrombocytopenia.
Efficacy of rapamycin in the treatment of secondary immune thrombocytopenia
Evans Syndrome (ES) is a rare disorder that was initially described as the presence of autoimmune hemolytic anemia (AIHA) and immune thrombocytopenia with unknown etiology [Citation33]. In one study of rapamycin in ES, five ES patients were recruited, and four had a response to rapamycin, with half of them achieving CR and the other two achieving an asymptomatic partial response [Citation29]. Li et al. [Citation30] recruited 12 ES patients and treated with rapamycin, and the ORR at 12 months was 91.7% with a CR rate of 33.3%.
Autoimmune lymphoproliferative syndrome (ALPS), caused by defective lymphocyte homeostasis, is characterized by non-malignant lymphoproliferation, autoimmune disease mostly directed toward blood cells (thrombocytopenia accounts for 54%) and lifelong increased risk for lymphoma [Citation34,Citation35]. Several case series have reported that Rapamycin can effectively control ALPS, which is associated with modulation of the double-negative T cells (DNT)/Treg homeostasis [Citation36–39].
Lai et al. [Citation40] used rapamycin to treat systemic lupus erythematosus (SLE) patients with platelet count (PC) ≥ 100 × 109/L, and, unexpectedly, they observed an elevated PC (p = 0.04). Subsequently, a single-arm, phase II study enrolled 20 refractory connective tissue disease-related thrombocytopenia (CTD-TP) patients. Among the 14 SLE patients, the ORR was 71.4%, with a CR rate of 64.3%. The median platelet counts increased from 34 × 109/L before Rapamycin administration to 82 × 109/L at the 6-month follow-up visit [Citation41]. Notably, none of the three Primary Sjö-gren's syndrome patients included responded to sirolimus therapy, although one study once reported that rapamycin suppressed lacrimal gland inflammation in mice [Citation42].
Xie et al. reported rapamycin monotherapy for thrombocytopenia in seven patients with primary antiphospholipid syndrome (APS) and observed an increased PC after Rapamycin administration. The median PC of 59 × 109/L was significantly increased to 90 × 109/L at the first month (p = 0.028), 131 × 109/L at 3 months (p = 0.028) and 178 × 109/L at 6 months (p = 0.018) [Citation43]. In addition, there are case reports that mTORi is effective for thrombocytopenia secondary to some rare diseases, such as COVID-19 vaccine-related ITP [Citation4], or thrombocytopenia secondary to some immunotherapy, like CD19 CAR-T-Cell therapy [Citation44]. In summary, at present, rapamycin is effective for several secondary ITPs, mentioned above. Whether it is effective for other secondary ITPs, such as virus-related thrombocytopenia, (e.g. hepatitis B virus, COVID-19, influenza virus, etc.) require additional clinical evidence.
Potential mechanism of rapamycin and its use in ITP treatment
Concerning ITP treatment, the therapeutic effects of mTORi are attributed to its newly discovered roles in the survival, proliferation, differentiation and autophagy of T and B lymphocytes, dendritic cells (DCs), myeloid-derived suppressor cells (MDSCs), and platelets themselves – as well as regulation of these various cell types’ function, such as cytokine secretion.
Rapamycin modulates lymphocyte survival and function
Abnormal T cells have been described in ITP, including a higher T-helper cell frequency, a lower frequency of circulating CD4+ CD25+ FoxP3+ Tregs (regulatory T cells) and ratio imbalance of Th1/Th2 and Treg/Th17 [Citation45,Citation46]. Rapamycin may modulate this balance in three distinct ways. First, it can specifically target on mTOR complex of T lymphocytes, phosphorylate the carboxyl terminus of mTOR, block interleukin (IL)-2, IL-15 and CD28/B7 co-stimulatory pathways for T-cell activation, reduce DNA self-replication of T cells, and finally cause cell cycle arrest and proliferation decrease of T cells [Citation47]. Second, mTOR inhibitors reduce the differentiation of T cells into Th1, Th2 and Th17 cells, resulting in the diminished immune response [Citation48,Citation49]. Third, studies have shown that the mTOR pathway stimulates gene transcription and induces cell growth and proliferation, by regulating NFAT, AP-1, STAT and other signaling molecules, and these regulations are increased in Th1, Th2 and Th17 cells, but not in Treg cells [Citation50]. Therefore, the application of mTOR inhibitor (for instance, rapamycin) can increase the proportion of Treg cells in helper T lymphocyte subsets, thereby further exerting its negative immunoregulatory effect and achieving immune tolerance [Citation48].
As mentioned previously, the cellular underpinnings of ITP are complex; in addition to T cell immune abnormalities, it also includes B-cell immune abnormalities. About 40–60% of patients with ITP have detectible plasma and/or platelet-bound autoantibodies [Citation51,Citation52], suggesting abnormal B-cell function. It was found that the expression of B-cell activating factor (BAFF) increased in ITP patients [Citation53]. Recent studies have shown that Rapamycin inhibited human soluble BAFF-stimulated cell proliferation by inducing G1-cell cycle arrest, downregulating the levels of anti-apoptotic proteins (Mcl-1, Bcl-2, Bcl-xL and survivin), and suppressing mTOR-mediated PP2A-Erk1/2 signaling pathway in normal and neoplastic B-lymphoid cells [Citation54–56]. Rapamycin inhibits T-helper cell differentiation, which results in reduced B-cell activation and differentiation (transitional B cells and plasma blasts) [Citation57]. Patients with ITP have decreased number and function of CD19+ CD24+ CD38+ Bregs [Citation58,Citation59], Breg cells inhibit the differentiation of naive T cells into Th1 and Th17 by secreting IL-10 and promote the transformation of CD4+ CD25- T cells into Tregs cells [Citation60]. Rapamycin has been shown to ameliorate steroid-resistant rejection and induce long-term immune tolerance by amplifying CD19+ CD24+ CD38+ Bregs significantly among liver transplant recipients [Citation61]. Therefore, we speculate that one of the mechanisms of Rapamycin in the treatment of ITP includes restoring the number and function of Bregs, but this needs to be confirmed by biomarker and clinical data.
Besides T and B cells, it is also reported that MDSCs decrease in active ITP patients [Citation24], while relapsed ITP patients show lower MDSC levels compared with new patients [Citation62]. Impaired aerobic metabolism in MDSCs was confirmed to be involved in the pathogenesis of glucocorticoid resistance in ITP [Citation63]. In our report, Rapamycin treatment in ITP was associated with an increase in the percentage of M-MDSCs and Tregs, indicating that mTOR inhibitor may reestablish peripheral tolerance of ITP [Citation24].
Rapamycin modulates dendritic cells and cytokines secretion
Rapamycin also can affect dendritic cells (DC) and cytokine secretion. Rapamycin modulates the level of IL-12 secreted by DC cells through the Jak2/stat4 signaling pathway. In addition, rapamycin has differential effects on mature and immature DC, resulting in a reduction in IL-12 secretion from immature DCs, while the secretion of IL-12 from mature DCs was unchanged, resulting in a reduction in the activation of T lymphocytes [Citation64]. Second, rapamycin can significantly inhibit the expression of CD40 and CD86, which are DC co-stimulatory molecules, thus inducing tolerance of DCs and hindering T-cell proliferation [Citation65]. Furthermore, mTORi reduced inflammatory factors, such as IL-17, IFN-γ [Citation66], TNF-α, IL-6 and IL-8 production [Citation67], but increased the expression of anti-inflammatory cytokines IL-10 and TGF-β [Citation28,Citation68,Citation69] in vitro and in vivo.
Rapamycin enhances platelet autophagy
The role of autophagy in megakaryopoiesis, thrombopoiesis and platelet function is gradually being uncovered [Citation70]. Platelet autophagy, regulated by the PI3K/AKT/mTOR pathway, was found to be diminished in ITP patients. The enhancement of platelet autophagy alleviated platelet destruction [Citation71]. Abnormal autophagy in ITP patients may be caused by the deletion of autophagy-related genes such as ATG7 (autophagy-related genes 7) and abnormal signaling due to overexpression of mTOR [Citation72], thereby highlighting an additional mechanism of mTORi efficacy in ITP treatment [Citation24,Citation31,Citation32].
Adverse reactions and concentration monitoring of rapamycin
To be honest, the reports mentioned above are mostly case series, lacking high level of evidence. And excessive enthusiasm for rapamycin needs to be toned down, and attention should be paid to the side effects of mTOR inhibitors. Rapamycin has a strong inhibitory effect on the immune system, thus increasing the probability of infection in patients being administered rapamycin [Citation73,Citation74]. Adverse effects that have been reported so far include: stomatitis and oral ulcers, hyperlipidemia, infection, liver damage, increased blood pressure, edema, proteinuria, hypokalemia and thrombotic microangiopathy [Citation24,Citation37,Citation75]. Our previous article reported that the most common side effects of rapamycin in R/R ITP patients were hyperlipidemia (12%) and mucositis (especially mouth ulcers 7%) [Citation24]. According to reports, the above-mentioned side effects of rapamycin are mostly mild, transient and reversible, and often appear in the first 3 months of administration. Discontinuation of Rapamycin is not necessary in most cases, but symptomatic treatment is necessary [Citation27]. As mTOR inhibitors inhibit the proliferation of endothelial cells and fibroblasts, postoperative wound healing may also be affected. However, these adverse effects are dose dependent, when blood drug concentration is reduced, side effects improve [Citation76]. The recommended plasma drug concentration of rapamycin should be kept at 5–15 ng/ml [Citation77]. It is important to monitor the drug concentration of rapamycin. Normally, it is recommended to monitor concentration once a week for the first 3 months after administration, then once a month thereafter. When the dose of rapamycin is increased or decreased or when it is given concomitantly with drugs metabolized through cytochrome P450, the concentration needs to be monitored more closely [Citation78].
Future and perspective
Although there are various treatment regimens recommended by ITP-relevant guidelines, the complex pathogenesis of refractory ITP results in varied efficacy and safety of those second-line regimens. Rapamycin has been used in R/R ITP treatment in recent years, due to its strong immunomodulatory effect and tolerable safety profile [Citation79–81]. In light of that, the focus of this article is to summarize recent publications reporting clinical progress of rapamycin application in the treatment of ITP and explore the understanding of its underlying mechanism.
Rapamycin, as a multifaceted immunomodulator, can regulate T cells, B cells and MDSCs function in ITP, as described above and depicted in . Besides, rapamycin can directly inhibit the uptake of saturated fatty acids in bone marrow mesenchymal stem cells, reduce the proportion of fat in the bone marrow to improve the hematopoietic microenvironment and promote the production of human platelets [Citation80,Citation82]. Because of these mechanisms, mTORi, either alone or in combination, plays important roles in immune hematological diseases, including ITP, AIHA [Citation25], GVHD [Citation26] and PRCA [Citation83], among others.
Figure 1. Potential mechanism of rapamycin in ITP: Rapamycin modulates lymphocyte survival and function, including T, B and MDSC cells. Second, Rapa reduced inflammatory factors but increased the expression of anti-inflammatory cytokines. Third, Rapa enhances platelet autophagy.
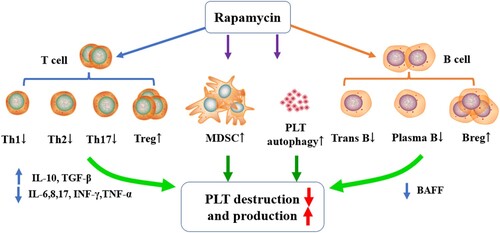
At present, favorable clinical evidence as to the safety and efficacy in the treatment of various immune thrombocytopenia with rapamycin is beginning to accumulate; however, this application is in an early stage with lacking high level of evidence and there are several unanswered questions. First, most of the studies of rapamycin in ITP explore its use in the third line or beyond (3L+); whether it can be used as a front-line drug in ITP treatment needs to be evaluated by more randomized controlled trials. Second, we have not seen reports on the application of other mTOR inhibitors (Everolimus, Temsirolimus, etc.) in ITP patients. Are other mTORi ineffective or simply have not yet been tried? Third, which patients might benefit more from rapamycin compared to other agents or be more at risk of certain side effects (e.g. infection or mucositis) remains to be explored. Perhaps, measurement of baseline mTOR activity in ITP patients before administration may help predict response? Fourth, some ITP patients are indeed sensitive but may be dependent on rapamycin; however, tapering has not been fully studied. From our clinical experience, for patients with CR to rapamycin, with PLT ≥ 70 × 109/L for 3 months, tapering down (0.5 mg/2 weeks) may be safely tolerated. Finally, to reduce platelet destruction and promote platelet production, can rapamycin be combined with TPO-Ras or other agents to achieve better efficacy in the treatment of R/R ITP? We and others are currently performing additional clinical studies to address these important questions for ITP patients.
Disclosure statement
No potential conflict of interest was reported by the author(s).
Additional information
Funding
References
- Zahidin MA, Mohd Noor NH, Johan MF, et al. A review on secondary immune thrombocytopenia in Malaysia. Healthcare (Basel). 2022;10:38.
- Rodeghiero F, Stasi R, Gernsheimer T, et al. Standardization of terminology, definitions and outcome criteria in immune thrombocytopenic purpura of adults and children: report from an international working group. Blood. 2009;113:2386–2393.
- Liu XG, Bai XC, Chen FP, et al. Chinese guidelines for treatment of adult primary immune thrombocytopenia. Int J Hematol. 2018;107:615–623.
- Feng Y, Quan Y, Cassady K, et al. Clinical characteristics in immune thrombocytopenia patients after COVID-19 vaccination. Hum Vaccin Immunother. 2022;7:2119043. doi:10.1080/21645515.2022.2119043.
- Kim DS. Recent advances in treatments of adult immune thrombocytopenia. Blood Res. 2022;57:S112–S119.
- Boyle S, White RH, Brunson A, et al. Splenectomy and the incidence of venous thromboembolism and sepsis in patients with immune thrombocytopenia. Blood. 2013;121:4782–4790.
- Miltiadous O, Hou M, Bussel JB. Identifying and treating refractory ITP: difficulty in diagnosis and role of combination treatment. Blood. 2020;135:472–490.
- Provan D, Arnold DM, Bussel JB, et al. Updated international consensus report on the investigation and management of primary immune thrombocytopenia. Blood Adv. 2019;3:3780–3817.
- Lv Y, Shi H, Liu H, et al. Current therapeutic strategies and perspectives in refractory ITP: what have we learned recently? Front Immunol. 2022;13:953716.
- Yu J, Xu Z, Zhuo Y, et al. Development and validation of a nomogram for steroid-resistance prediction in immune thrombocytopenia patients. Hematology. 2021;26:956–963.
- Gea-Banacloche JC. Rituximab-associated infections. Semin Hematol. 2010;47:187–198.
- Horikoshi A, Tsukuda J, Abe R, et al. Development of myelofibrosis during eltrombopag treatment in a patient with immune thrombocytopenia. Rinsho Ketsueki. 2016;57:638–641.
- Wong RSM, Saleh MN, Khelif A, et al. Safety and efficacy of long-term treatment of chronic/persistent ITP with eltrombopag: final results of the EXTEND study. Blood. 2017;130:2527–2536.
- Ghanima W, Geyer JT, Lee CS, et al. Bone marrow fibrosis in 66 patients with immune thrombocytopenia treated with thrombopoietin-receptor agonists: a single-center, long-term follow-up. Haematologica. 2014;99:937–944.
- Wong RSM, Khelif A, Salama A, et al. Occurrence and management of cataracts in adult patients (PTS) with chronic immune thrombocytopenia (cITP) during long-term treatment with eltrombopag (EPAG): results from the extend study. Blood. 2017;130.
- Janku F. Phosphoinositide 3-kinase (PI3K) pathway inhibitors in solid tumors: from laboratory to patients. Cancer Treat Rev. 2017;59:93–101.
- Zhang Y, Zhang R. Recent advances in analytical methods for the therapeutic drug monitoring of immunosuppressive drugs. Drug Test Anal. 2018;10:81–94.
- Hua H, Kong Q, Zhang H, et al. Targeting mTOR for cancer therapy. J Hematol Oncol. 2019;12:71.
- Linke M, Fritsch SD, Sukhbaatar N, et al. mTORC1 and mTORC2 as regulators of cell metabolism in immunity. FEBS Lett. 2017;591:3089–3103.
- Li HL, Deng NH, He XS, et al. Small biomarkers with massive impacts: PI3K/AKT/mTOR signalling and microRNA crosstalk regulate nasopharyngeal carcinoma. Biomark Res. 2022;10:52.
- Fuhler GM, Tyl MR, Olthof SG, et al. Distinct roles of the mTOR components rictor and raptor in MO7e megakaryocytic cells. Eur J Haematol. 2009;83:235–245.
- Webster AC, Lee VW, Chapman JR, et al. Target of rapamycin inhibitors (sirolimus and everolimus) for primary immunosuppression of kidney transplant recipients: a systematic review and meta-analysis of randomized trials. Transplantation. 2006;81:1234–1248.
- Al-Hammadi S, Almarzooqi S, Albawardi A, et al. Effects of molecularly targeted therapies on murine thymus: highly selective mTOR inhibitors induce reversible thymic involution. Exp Hematol Oncol. 2015;5:22.
- Feng YM, Xiao YS, Yan HJ, et al. Sirolimus as rescue therapy for refractory/relapsed immune thrombocytopenia: results of a single-center, prospective, single-arm study. Front Med (Lausanne). 2020;7:110. doi:10.3389/fmed.2020.00110.
- Hongju Y, Ping W, Yao Q, et al. Clinical efficacy of sirolimus in treatment of relapsed and refractory autoimmune hemolytic anemia in adults. J Third Mil Med Univ. 2020;42:1757–1762. [in Chinese].
- Zhu W, Feng YM, Chen T, et al. The clinical observation of sirolimus combined with calcineurin inhibitors for steroid-resistant/steroid-dependent extensive cGVHD. Zhonghua Xue Ye Xue Za Zhi. 2020;41:716–722.
- Feng Y, Chen X, Cassady K, et al. The role of mTOR inhibitors in hematologic disease: from bench to bedside. Front Oncol. 2021;10:611690.
- Li J, Wang Z, Dai L, et al. Effects of rapamycin combined with low dose prednisone in patients with chronic immune thrombocytopenia. Clin Dev Immunol. 2013;2013:548085.
- Jasinski S, Weinblatt ME, Glasser CL. Sirolimus as an effective agent in the treatment of immune thrombocytopenia (ITP) and Evans Syndrome (ES): a single institution's experience. J Pediatr Hematol Oncol. 2017;39:420–424.
- Li HM, Ji J, Du YL, et al. Sirolimus is effective for primary relapsed/refractory autoimmune cytopenia: a multicenter study. Exp Hematol. 2020;89:87–95.
- Mousavi-Hasanzadeh M, Bagheri B, Mehrabi S, et al. Sirolimus versus cyclosporine for the treatment of pediatric chronic immune thrombocytopenia: a randomized blinded trial. Int Immunopharmacol. 2020;88:106895.
- Li Q, Kang HY, Fan L, et al. Clinical study on calcitriol combined with sirolimus in the treatment of chronic primary immune thrombocytopenia patients. Zhongguo Shi Yan Xue Ye Xue Za Zhi. 2021;29:1911–1916.
- Miano M. How I manage Evans Syndrome and AIHA cases in children. Br J Haematol. 2016;172:524–534.
- Niss O, Sholl A, Bleesing JJ, et al. IL-10/Janus kinase/signal transducer and activator of transcription 3 signaling dysregulates Bim expression in autoimmune lymphoproliferative syndrome. J Allergy Clin Immunol. 2015;135:762–770.
- Consonni F, Gambineri E, Favre C. ALPS, FAS, and beyond: from inborn errors of immunity to acquired immunodeficiencies. Ann Hematol. 2022;101:469–484.
- Gu H, Chen Z, Ma J, et al. Sirolimus is effective in autoimmune lymphoproliferative syndrome-type III: a pedigree case report with homozygous variation PRKCD. Int J Immunopathol Pharmacol. 2021;35:20587384211025934.
- Bride KL, Vincent T, Smith-Whitley K, et al. Sirolimus is effective in relapsed/refractory autoimmune cytopenias: results of a prospective multi-institutional trial. Blood. 2016;127:17–28.
- Gu H, Ma J, Chen Z, et al. Synergistic defects of novo FAS and homozygous UNC13D leading to autoimmune lymphoproliferative syndrome-like disease: a 10-year-old Chinese boy case report. Gene. 2018;672:45–49.
- Nocerino A, Valencic E, Loganes C, et al. Low-dose sirolimus in two cousins with autoimmune lymphoproliferative syndrome-associated infection. Pediatr Int. 2018;60:315–317.
- Lai ZW, Kelly R, Winans T, et al. Sirolimus in patients with clinically active systemic lupus erythematosus resistant to, or intolerant of, conventional medications: a single-arm, open-label, phase 1/2 trial. Lancet. 2018;391:1186–1196.
- Wu C, Wang Q, Xu D, et al. Sirolimus for patients with connective tissue disease-related refractory thrombocytopenia: a single-arm, open-label clinical trial. Rheumatology (Oxford). 2021;60:2629–2634.
- Shah M, Edman MC, Reddy Janga S, et al. Rapamycin eye drops suppress lacrimal gland inflammation In a murine model of Sjögren's syndrome. Invest Ophthalmol Vis Sci. 2017;58:372–385.
- Xie W, Ji L, Zhang Z. Sirolimus monotherapy for thrombocytopenia in primary antiphospholipid syndrome: a pilot study from a tertiary referral center. Front Immunol. 2022;13:857424.
- Xing L, Wang Y, Liu H, et al. Case report: sirolimus alleviates persistent cytopenia after CD19 CAR-T-cell therapy. Front Oncol. 2021;11:798352.
- Takahashi N, Saitoh T, Gotoh N, et al. The cytokine polymorphisms affecting Th1/Th2 increase the susceptibility to, and severity of, chronic ITP. BMC Immunol. 2017;18:26.
- Zufferey A, Kapur R, Semple JW. Pathogenesis and therapeutic mechanisms in immune thrombocytopenia (ITP). J Clin Med. 2017;6:16.
- Manning BD. Game of TOR — the target of rapamycin rules four kingdoms. N Engl J Med. 2017;377:1297–1299.
- Stallone G, Infante B, Di Lorenzo A, et al. mTOR inhibitors effects on regulatory T cells and on dendritic cells. J Transl Med. 2016;14:152.
- Song X, Gao J, Liu H, et al. Rapamycin alleviates renal damage in mice with systemic lupus erythematosus through improving immune response and function. Biomed Pharmacother. 2021;137:111289.
- Terziroli Beretta-Piccoli B, Mieli-Vergani G, Vergani D. Autoimmune hepatitis: standard treatment and systematic review of alternative treatments. World J Gastroenterol. 2017;23:6030–6048.
- Yu Y, Hou Y, Zhao Y, et al. Platelet autoantibody specificity and response to rhTPO treatment in patients with primary immune thrombocytopenia. Br J Haematol. 2021;194:191–194.
- Zhang HY, Hou M, Zhang XH, et al. The diagnostic value of platelet glycoprotein-specific autoantibody detection in idiopathic thrombocytopenic purpura. Zhongguo Shi Yan Xue Ye Xue Za Zhi. 2004;12:204–206.
- Zhu XJ, Shi Y, Peng J, et al. The effects of BAFF and BAFF-R-Fc fusion protein in immune thrombocytopenia. Blood. 2009;114:5362–5367.
- Zeng Q, Qin S, Zhang H, et al. Rapamycin attenuates BAFF-extended proliferation and survival via disruption of mTORC1/2 signaling in normal and neoplastic B-lymphoid cells. J Cell Physiol. 2018;233:516–529.
- Zeng Q, Zhou Z, Qin S, et al. Rapamycin inhibits B-cell activating factor (BAFF)-stimulated cell proliferation and survival by suppressing Ca(2+)-CaMKII-dependent PTEN/Akt-Erk1/2 signaling pathway in normal and neoplastic B-lymphoid cells. Cell Calcium. 2020;87:102171.
- Zeng Q, Zhang H, Qin J, et al. Rapamycin inhibits BAFF-stimulated cell proliferation and survival by suppressing mTOR-mediated PP2A-Erk1/2 signaling pathway in normal and neoplastic B-lymphoid cells. Cell Mol Life Sci. 2015;72:4867–4884.
- Kraaijeveld R, Li Y, Yan L, et al. Inhibition of T helper cell differentiation by tacrolimus or sirolimus results in reduced B-cell activation: effects on T follicular helper cells. Transplant Proc. 2019;51:3463–3473.
- Yu TS, Wang HY, Zhao YJ, et al. Abnormalities of bone marrow B cells and plasma cells in primary immune thrombocytopenia. Blood Adv. 2021;5:4087–4101.
- Semple JW. Bregging rights in ITP. Blood. 2012;120:3169.
- Flores-Borja F, Bosma A, Ng D, et al. CD19+ CD24hiCD38hi B cells maintain regulatory T cells while limiting TH1 and TH17 differentiation. Sci Transl Med. 2013;5:173ra123.
- Song J, Du G, Chen W, et al. The advantage of Sirolimus in amplifying regulatory B cells and regulatory T cells in liver transplant patients. Eur J Pharmacol. 2020;869:172872.
- Zhou J, Zhou Y, Wen J, et al. Circulating myeloid-derived suppressor cells predict disease activity and treatment response in patients with immune thrombocytopenia. Braz J Med Biol Res. 2017;50:e5637.
- Hou Y, Xie J, Wang S, et al. Glucocorticoid receptor modulates myeloid-derived suppressor cell function via mitochondrial metabolism in immune thrombocytopenia. Cell Mol Immunol. 2022. doi:10.1038/s41423-41022-00859-41420.
- Wang GY, Chen GH, Li H, et al. Rapamycin-treated mature dendritic cells have a unique cytokine secretion profile and impaired allostimulatory capacity. Transpl Int. 2009;22:1005–1016.
- Turnquist HR, Cardinal J, Macedo C, et al. mTOR and GSK-3 shape the CD4+ T-cell stimulatory and differentiation capacity of myeloid DCs after exposure to LPS. Blood. 2010;115:4758–4769.
- Borim PA, Mimura LAN, Zorzella-Pezavento SFG, et al. Effect of rapamycin on MOG-reactive immune cells and lipopolysaccharide-activated microglia: an in vitro approach for screening new therapies for multiple sclerosis. J Interferon Cytokine Res. 2022;42:153–160.
- Liu J, Zhang F, Zhang Z, et al. Everolimus ameliorates Helicobacter pylori infection-induced inflammation in gastric epithelial cells. Bioengineered. 2022;13:11361–11372.
- Nguyen JH, Jiang LJ, Kang L, et al. Intranodal sirolimus induces regulatory T cells in human hepatic lymph nodes via interleukin 10 signaling. Liver Transpl. 2021;27:1669–1672.
- Wise RM, Harrison MAA, Sullivan BN, et al. Short-term rapamycin preconditioning diminishes therapeutic efficacy of human adipose-derived stem cells in a murine model of multiple sclerosis. Cells. 2020;9.
- Liu Z, Mei T. Immune thrombocytopenia induces autophagy and suppresses apoptosis in megakaryocytes. Mol Med Rep. 2018;18:4016–4022.
- Wang CY, Ma S, Bi SJ, et al. Enhancing autophagy protects platelets in immune thrombocytopenia patients. Ann Transl Med. 2019;7:134.
- Sun RJ, Shan NN. Megakaryocytic dysfunction in immune thrombocytopenia is linked to autophagy. Cancer Cell Int. 2019;19:59.
- Guglieri-Lopez B, Perez-Pitarch A, Garcia-Cadenas I, et al. Effect of sirolimus exposure on the need for preemptive antiviral therapy for cytomeglovirus infection after allogeneic hematopoietic stem cell transplantation. Biol Blood Marrow Transplant. 2019;25:1022–1030.
- Taboada RG, Riechelmann RP, Mauro C, et al. Everolimus-induced pneumonitis in patients with neuroendocrine neoplasms: real-world study on risk factors and outcomes. Oncologist. 2022;27:97–103.
- Chen X, Sun H, Cassady K, et al. The addition of sirolimus to GVHD prophylaxis after allogeneic hematopoietic stem cell transplantation: a meta-analysis of efficacy and safety. Front Oncol. 2021;11:683263.
- Hoff U, Markmann D, Nieminen-Kelha M, et al. Low-dose rapamycin does not impair vascular integrity and tubular regeneration after kidney transplantation in rats. Sci Rep. 2021;11:16270.
- Hematopoietic Stem Cell Application Group CSoHCMA, China Association for the Prevention of Hematology D. Chinese consensus on the diagnosis and management of chronic graft-versus-host disease (2021). Zhonghua Xue Ye Xue Za Zhi. 2021;42:265–275.
- Nwaroh E, Jupp J, Jadusingh E, et al. Clinical impact and management of fluconazole discontinuation on sirolimus levels in bone marrow transplant patients. J Oncol Pharm Pract. 2018;24:235–238.
- Gu H, Wu RH. Advance of mechanisms and clinical applications about rapamycin for treating immune mediated hemocytopenia – review. Zhongguo Shi Yan Xue Ye Xue Za Zhi. 2018;26:1836–1840.
- You T, Wang Q, Zhu L. Role of autophagy in megakaryocyte differentiation and platelet formation. Int J Physiol Pathophysiol Pharmacol. 2016;8:28–34.
- Xu Z, Chu M. Advances in immunosuppressive agents based on signal pathway. Front Pharmacol. 2022;13:917162.
- Li Y, Wang C, Zhang G, et al. Role of autophagy and mTOR signaling in neural differentiation of bone marrow mesenchymal stem cells. Cell Biol Int. 2014;38:1337–1343.
- Huang Y, Chen M, Yang C, et al. Sirolimus is effective for refractory/relapsed/intolerant acquired pure red cell aplasia: results of a prospective single-institutional trial. Leukemia. 2022;36:1351–1360.