Abstract
The interaction of sodium (Na) and potassium (K) on growth, yield, nutrients and citric acid composition of fruit of tomato (Lycopersicon lycopersicum [L.] Karst) was investigated. Six rates of Na at 0, 2, 4, 8, 16 and 32 mgkg−1 soil and four rates of K at 0, 32, 64 and 128 mgkg−1 soil, applied as NaCl and KCl, respectively, were arranged in a randomized complete block design and replicated three times. Plant growth rate, number of flowers and fruit yields, nutrient composition and citric acid content of fruit juice were determined. The application of 2–4 mg Na kg−1 soil with 32–64 mg K kg−1 soil increased fruit yield by about 100%. Fruit yield correlated positively with the number of leaves (0.65***), number of flowers (0.57**) and shoot-N (0.40*). Sodium applied at Na:K ratio of 1:8 to 1:32 increased the growth and yield of the tomato plant.
Introduction
Soils of southwestern Nigeria contain approximately equal levels of sodium (Na) and potassium (K) (Aduayi et al. Citation1999, Akintunde et al. Citation2000). The concentration of Na increased towards the northern zone of the country (Odunze & Esu Citation1989, Idowu Citation2005). Sodium and potassium have almost identical chemical properties in the soil (Peter Citation1993). Plants, including tomato (Lycopersicon lycopersicum) absorb relatively high levels of sodium (Wybenga Citation1975). The functions of potassium in the nutrition of plants are fully known but those of sodium are not well documented (Idowu & Aduayi Citation2003). Some crops showed a yield response to sodium even in the presence of adequate amounts of potassium. For instance, sodium cannot be substituted completely by potassium in marigolds (Tagetes erecta), spinach (Amaranthus sp.) (Marschner & Possingham Citation1975), sugar beet (Beta vulgaris L.) (Beringer Citation1987) and fodder beet (Beta vulgaris L.) (Phillips & Chiy Citation1995).
Reports on the functions of Na on higher plants are inconsistent. Lawlor and Milford (Citation1973) and Wyn Jones et al. (Citation1979) independently found an increase in the growth, tuber yield and sugar quality of sugar beet at the application of Na with K. Phillips and Chiy (Citation1995) noted that grasses treated with Na are more palatable and more acceptable by animals and consequently increased milk production. The increase in the yield of crop was attributed to better plant water management when sodium was applied with potassium. Jeschke (Citation1977) and Nunes et al. (Citation1984) suggested that Na might surpass K in the contribution to the solute potential in vacuoles, in the generation of turgor and cell expansion in some plants. Conversely, Marschner (Citation1998) found no significant difference in the percentage of water in leaves of tomato at the application of sodium with potassium. The trends on the role of sodium in plant nutrition are moving steadily towards its being recognized as an essential plant nutrient element. More recent studies observed that the application of low levels of sodium, along with major nutrients in order to obtain the desired electrical conductivity improved the performance and yield of tomato in the nutrient culture, and reduced the cost of production (Papadopoulos et al. Citation1999, Dorais et al. Citation2000). Malash et al. (Citation2002) also noted an improved growth and soluble salt of tomato in the presence of Na and K in nutrient medium.
Photochemicals in fruits and vegetables attracted a great deal of attention mainly due to their role in preventing diseases caused by oxidative stress (Nicoli et al. Citation1999, Lavelli et al. Citation2000). Oxidative stress releases free oxygen radicals in the body and has often resulted into a number of disorders, which include cardiovascular diseases, malnutrition, cataracts, cancers, rheumatic and many other auto-immune diseases besides ageing (Steinmetz & Potter Citation1996, Kaur & Kapoor Citation2001). Tomato is one of the leading edible vegetable crops worldwide (Adams Citation1991) and is a major source of antioxidants for the Nigerian population due to its high consumption level (Idowu & Aduayi Citation2006). As an antioxidant, it prevents the oxidation of free oxygen radical in human body (Bahorun et al. Citation2004). In the southwestern Nigeria, tomato is grown typically on ultisol (Typic Paleustult) because of its fine texture but the soil is highly weathered and has low fertility status, which requires high application of potassium for optimal tomato production. However, potassium fertilizers are too expensive for resource-poor farmers.
Loss of tomato fruits during harvesting, transportation and storage is another problem of tomato production in Nigeria. Moretti et al. (Citation1998) and Ergun et al. (Citation2006) reported a reduction in flavor acceptability, carotenoids, vitamin C and titratable acidity (citric acid) of broken tomato fruits. Thew citric acid content of tomato fruits influences the shelf life of tomato, and hence, enhances the fruit quality. Citric acid content in fruit juice is controlled by cation:anion ratio of plant cells (Adams Citation1986). In another study, Papadopoulos et al. (Citation1999) observed that the cation:anion ratio is controlled by sodium and potassium content of plants. Thus, the application of sodium along with the major nutrients could be an economic way to achieve sustainable tomato production in Nigeria and in Africa in general (Idowu & Aduayi Citation2003).
The results of the preliminary study by Idowu and Aduayi (Citation2006) showed positive interaction of Na and K on shoot water content, which reduced fruit abortion of tomato. The current study examined the interaction of low levels of sodium with potassium on growth, yield and citric acid content of tomato, with the view to establishing the optimal levels of sodium and potassium that would promote good growth, yield and quality of tomato in soil culture.
Materials and methods
Description of the study area
The experiment was conducted in a greenhouse at the Obafemi Awolowo University (O. A. U), Ile-Ife during 2002 and 2003. Ile-Ife lies between latitudes 7° 31′ and 7° 33′ N and longitudes 4° 33′ and 4° 34′ E, and is located in the forest zone of southwestern Nigeria. The study was conducted in the greenhouse. The pattern of rainfall is bimodal, with the average annual rainfall estimated to about 1400 mm. The average monthly temperature ranges from 18.9–34.6°C and the mean monthly relative humidity is 61% and 83% for the early and late planting seasons, respectively. Light intensity of the greenhouse ranged between 46.54 and 262.26 Candle light, compared with 180.41 and 3348.86 Candle light for outside the greenhouse.
Soil sampling, preparation and analysis
Bulk surface soil samples (0–15 cm) were collected from unfertilized plots from Obafemi Awolowo University, Teaching and Research Farm, Ile-Ife. Soils at the sampling site were classified as Iwo series (Smyth & Montgomery Citation1962) and as Typic Paleustult (Soil Survey Staff Citation1975, Okusami & Oyediran Citation1985), the typical soil for tomato cultivation in the region. Soil samples were air dried, crushed in agate mortar, passed through a 2-mm sieve, and analyzed for Na and K, and other elements. Total Na and K in soils were determined by wet digestion technique using combinations of Hydrogen Flouride and H2SO4 as described by Klesta and Bartz (Citation1996). Exchangeable K, Ca, Na and Mg were extracted with neutral (pH 7) solution of 1N NH4OAc. Potassium, Ca and Na were determined using the flame photometer and Mg by the atomic absorption spectrophotometer (AAS).
Particle size distribution was determined by the modified hydrometer method (Bouyoucous Citation1962) using 0.2 M NaOH solution as the dispersing agent. The field moisture capacity (FMC) was determined according to the method of Hanks et al. (Citation1954). Soil pH was determined using a glass electrode pH meter in both distilled water and 0.01 M CaCl2 solution, using 1:2 soil:CaCl2 solution as described by Thomas (Citation1996). Soil organic carbon was determined by the chromic acid digestion method of Walkley and Black (Citation1934). The total N concentration was determined by macro-Kjeldahl method according to Bremner (Citation1996), and the available P was determined by Bray-1 method as described by Kuo (Citation1996).
Soil culture experimental design
The experiment was a 6×4 factorial, arranged in a randomized complete block design (RCBD). The factors were six levels of Na treatments: 0, 2, 4, 8, 16 and 32 mg Na kg−1 soil applied as NaCl and four levels of K treatments: 0, 32, 64 and 128 mg K kg−1 soil applied as KCl and replicated three times. Two kilograms of soil were weighed into each pot. To each pot was added a previously determined concentration of basal elements (), in addition to the treatments. All nutrients were added by weight and mixed thoroughly with the main treatments, starting with lower concentrations of Na and K to the highest concentrations. The mixture was moistened and left for three days before transferring the tomato seedlings.
Table I Sources and concentration of nutrient elements in the greenhouse study.
Seedling preparation and planting in the greenhouse
Tomato seeds of cultivar DT97/74 were obtained from the National Horticultural Research Institute (NIHORT), Ibadan. The tomato plant has indeterminate fruiting habit and high yielding potential. The fruits were smoothly grooved to spiral shape with high seed content. The tomato seeds were first raised in the nursery in sterilized soils obtained from the Teaching and Research Farm using wooden trays, for four weeks in the greenhouse. The soils used for the study were sterilized to eliminate nematodes. At transplanting, vigorous seedlings of uniform height were selected and two seedlings were planted per pot. Pots were supplied with deionized water at a consistent volume to maintain 75% of field moisture capacity (FMC). Tomato seedlings were sprayed with dithane fungicide shortly after transplanting to prevent damping off disease and the treatment was repeated at 38 days. White flies, Bemisia tabaci, were noted on the plants 2 weeks after planting (WAP), hence, plants were sprayed with 500 mg kg−1 of Cypermethrim (Cymbush 10 EC) and this was repeated at 4, 8 and 13 WAP as described by Oyewole (Citation1988) and Blume and Jara (Citation2004). Plants were staked at 4 WAP.
Agronomic characteristics of tomato
The number of flowers was recorded weekly. The fruit set data were obtained after inflorescence and fruits were harvested and weighed fresh as they ripened. Symptoms of nutritional stress on the foliage of plants were also observed and recorded on weekly basis.
Determination of citric acid content of tomato fruit juice
Freshly collected ripe fruits were cleaned, weighed and placed in polyethylene bags. Ten grams of ripe tomato fruits were ground in Philips blender and mixed with 10 ml distilled water. The mixture was filtered into a 100 ml flask, washing down the residue with small amounts of water until the residue left in the filter paper was pale in colour. The volume in the flask was then made up to 100 ml. Fifty milliliters of the filterate were pipetted into a 250 ml conical flask, and 100 ml of water added. The mixture was titrated with a 0.1N NaOH standard solution to pH 8.1, checking pH at intervals with a pH meter. Total acidity was expressed as the percentage of the predominant acid in the fruit and expressed as citric acid content in tomato. Percent citric acid was calculated using the following formula (Bernard Citation1965, Oyewole & Aduayi Citation1992):
Chemical analysis of plant tissue of tomato
The tomato plants, indeterminate type, were harvested at seven months when about seventy five percent reduction in floral formation was observed. The shoots were cut at soil level using a stainless blade and the roots were carefully removed by washing off the soil under running tap water, blotted dry and kept in well labeled paper bags. The plants were partitioned into shoots and roots, and were oven dried to constant weight at 65°C. Plant tissue was ground in a Wiley micro-hammer stainless mill to pass through a 1 mm sieve (Piper Citation1944), tissue samples were kept in labeled air tight containers. Before chemical analyses of the individual plant part, a portion of each sample was re-dried at 105°C and then placed in a desiccator and 0.5 g portion of ground plant tissue was ignited in a muffle furnace at 450°C for 2 h (until ash was whitish/greyish white). The ash was dissolved in 5 ml of 4N HCl solution as described by Piper (Citation1944). Concentrations of K, Ca and Na were determined by flame photometer while Mg was by AAS. Phosphorus was determined by the vanadomolybdate method (IITA Citation1979), and nitrogen content determination was by the micro-Kjeldahl method (Bremner Citation1996).
Data analysis
Data collected were subjected to analysis of variance (ANOVA) to assess treatment effects. Significant differences between means were determined by using Least Significant Difference (LSD) and the relationships between components were tested by correlation analysis (Steel & Torrie Citation1980, SAS Citation1990).
Results and discussion
Characteristics of the soil used for the greenhouse study
Soil used in this study was a loamy sand with adequate pH (6.14), high organic carbon (1.64 g kg−1), medium total N (1.9mg kg−1) and available P (12.75 mg kg−1) (). Exchangeable Ca, Mg, K and Na were 2.0, 2.1, 0.36, and 0.23 cmol kg−1 soil, respectively. The Na and K concentrations were within the medium fertility range with Na:K ratio of 1:3, supporting reports of Aduayi et al. (Citation1999) and Akintunde et al. (Citation2000). Potassium concentration of 0.1–0.45 cmol kg−1 soil were within the low range (International Fertilizer Development Centre [IFDC] Citation1984, Enwezor et al. Citation1990), and Liang (Citation1999) also reported Na concentration of 7 meq/L (161 mg kg−1 soil) as low, although, the Na requirements for high yield and good quality tomato fruits have not been established. With regard to potassium, Besford (Citation1978) found that the minimum concentration of K required to prevent visible deficiency symptom in tomato was 0.203 cmol kg−1 soil while 0.503 cmol kg−1 was required to produce maximum fruit yield. Aduayi and Onesirosan (Citation1979) suggested 200 mg K kg−1 soil for optimal fruit yield of tomato in Iwo series (Oxic Hapludalf). On the other hand, Usherwood (Citation1985) noted that for improved yield and quality of tomato fruit, potassium requirement could be as high as 400 mg K kg−1 soil.
Table II Characteristics of soils used for the soil culture studies prior to experimentation.
Morphological observations at vegetative and fruiting stages of the tomato plant
Tomato plants grown with the control treatment were normal with well-spaced foliages during the first 6 WAP. However, plants became stunted with small leaves and interveinal chlorosis of older leaves at 16 WAP. The application of 32–64 mg K kg−1 soil resulted in healthy plants with dark green, well-spaced foliages and strong stems. There was mild interveinal chlorosis of older leaves and no fruit set at 14 WAP. At 128 mg K kg−1 soil, plants were stunted with light green younger leaves, interveinal chlorosis was observed on leaves from middle of the plant downward, which later turned purplish coloration and no fruit was observed at 14 WAP.
Plant growth was moderate with bright green colour, broad and well spaced foliages, mild interveinal chlorosis of older leaves at 16 WAP when 2–8 mg Na kg−1 soil and 0 mg K kg−1 soil was applied but only treatment with 2 mg Na kg−1 soil with 0 mg K kg−1 soil produced one fruit at 13 WAP. The interveinal chlorosis was more severe on plants treated with 16 and 32 mg Na kg−1 soil without K application.
The interaction of 2–4 mg Na kg−1 soil with 32–64 mg K kg−1 soil resulted in vigorous and healthy plant with bright green leaves, broad, succulent and well-spaced foliages. At 13 WAP, one fruit was observed on plants treated with 2 mg Na kg−1 soil and 32 mg K kg−1 soil, whereas plants treated with 2 mg Na kg−1 soil and 64 mg K kg−1 soil produced three fruits, being the highest fruit yield observed at 13 WAP. The interaction of 8–32 mg Na kg−1 soil with 32–128 mg K kg−1 soil resulted in fair plant growth but no fruit formation at 14 WAP, while the adverse effects of the single application of either 32 mg Na kg−1 or 128 mg K kg−1 soil on plant growth were reversed with the interaction of 32 mg Na kg−1 soil and 128 mg K kg−1.
Nutrient composition of shoot of tomato
Nitrogen content was (p<0.05) increased at 2, 4 and 8 mg Na kg−1 soil but decreased significantly between 16 and 32 mg Na kg−1 soil compared to the control treatments (). This result agreed with the report of Papadopoulos et al. (Citation1999) on tomato, and Aduayi and Olowoyo (Citation2003) on okra (Abelmoschus caillei). The application of increasing levels of K up to 64 mg kg−1 soil reduced shoot-N significantly. Unlike nitrogen, application of Na at 16–32 mg kg−1 soil and K at 32–128 mg kg−1 soil increased phosphorus content in the shoot. Potassium content in the shoot was increased significantly at 4–16 mg Na kg−1 soil but significantly decreased at the highest rate of Na application (32 mg Na kg−1 soil). This result indicated an antagonistic relationship between Na and K in the soil at the highest Na application rate, reducing K uptake and transport to the shoot.
Table III Effects of Na and K interactions on nutrient composition of shoots of the tomato plant in soil culture.
Calcium content in the shoot was significantly increased up to 4 mg Na kg−1 soil and then decreased at higher Na rate. The trends were similar to the result observed for nitrogen content in the shoot. Increased leaf formation and delay leaf senescence were noted with an increase in calcium content in the shoot. This result was in line with an earlier observation by Al-harbi (Citation1995) when Na and Ca were applied to tomato plants. The application of K at zero Na had no significant effect on shoot-Ca when compared with the control treatment. Magnesium in the shoot decreased with increasing levels of either Na or K probably due to induced magnesium deficiency. Some of the plant treated with high level of either Na or K was stunted with leaves showed interveinal chlorosis. Moreover, the increasing levels of Na did not significantly affect sodium content in the shoot, while the application of 32 mg K kg−1 soil, slightly increased shoot-Na.
Considering the effects of Na and K interactions on N, P, K, Ca, Mg and Na in the shoot as presented in , nitrogen content in the shoot was significantly (p<0.05) increased at 2 up to 4 mg Na kg−1 soil with 32–128 mg K kg−1 soil. The effect of Na and K interactions on P and K were inconsistent. However, shoot-N, -P, -K, -Ca, -Mg and -Na were increased at 2 mg Na kg−1 soil with 64 mg K kg−1 soil. The composition of all these elements in the shoot at 2 mg Na kg−1 soil with 64 mg K kg−1 soil resulted in early fruiting of tomato. The combined synergistic effects of Na and K on nutrient contents in the shoot differ from the sum of their separate effects. Contrary to Usherwood (Citation1985) report that very high K would be needed for high yield and good quality tomato, the results of this study, indicated an efficient nutrient combination of Na and K at Na:K ratio of 1:8 to 1:32, which resulted in balanced tomato nutrition when compared with plants that received high doses of K.
Number of flowers
The number of flowers increased slightly up to 4 mg Na kg−1 soil and decreased to the value obtained for the control treatments at 8–32 mg Na kg−1 soil with 0 mg K kg−1 soil as shown in a. This observation is in line with the result obtained by Aduayi and Olowoyo (Citation2003), using okra as the test crop. The application of 32 mg K kg−1 soil with zero Na significantly increased the number of flowers, which decreased slightly at 64–28 mg K kg−1 soil at zero Na. These results agreed with the report of Adams (Citation1986) that application of high K level could induce magnesium deficiency and thus, depress the floral formation in the tomato plant.
Figure 1. Effects of different levels of Na and K on (a) number of flowers and (b) number of fruits of tomato in soil culture.
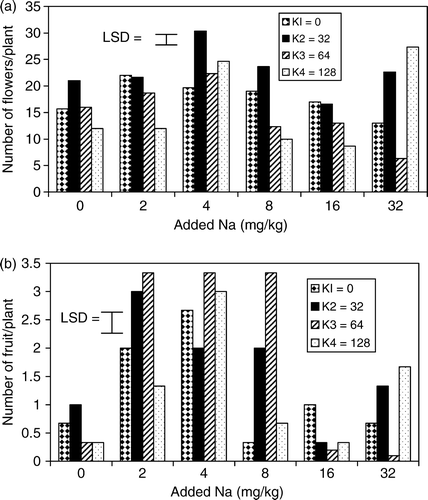
The interactions between Na and K at 2–4 mg Na kg−1 soil and 32–64 mg K kg−1 soil significantly increased the number of flowers but drastically depressed it at 8–16 mg Na kg−1 soil and 64–128 mg K kg−1 soil. The results of this study also indicated that at 2–4 mg Na kg−1 soil and 32–64 mg K kg−1 soil, leaves were succulent, thicker and broader, which enhanced floral formation. The addition of 32 mg Na kg−1 soil and 128 mg K kg−1 soil, reversed the depressing effect of separate application of either high Na or K on the floral production.
Fruit yield
The average number of fruits per plant and average weight of fruits obtained from the application of Na and K are presented in b and 2a. The number of fruits increased significantly (p<0.05) at 2–4 mg Na kg−1 soil but decreased at 8–32 mg Na kg−1 soil with 0 mg K kg−1 soil when compared with the control treatment. The trends of number of fruit were identical to the results obtained for the number of flowers. Interaction of 2–8 mg Na kg−1 soil with 32–64 mg K kg−1 soil significantly increased the number of fruits, which decreased consistently with increasing Na addition. The weight of fruit increased significantly at 32 and 64 mg K kg−1 soil but depressed at 128 mg K kg−1 soil, compared to the control treatments. The functions of K in tomato nutrition have been extensively investigated (Ho & Hewitt Citation1986); K was noted to be next to N for the large amount required for high fruit yields. However, the highest fruit weight of 16.10 g plant−1 obtained at 32 mg K kg−1 soil and 14.57 g plant−1 at 64 mg K kg−1 soil were lower than the fruit yields of 20.57 g plant−1 obtained at 2 mg K kg−1 and 20.71 g plant−1 at 4 mg Na kg−1 soil. This result supports the report of Mengel and Viro (Citation1974) that the function of K in partitioning of assimilates in the tomato plant needs further investigation.
The fruit yield obtained at the interaction of 2–4 mg Na kg−1 soil with 32–64 mg K kg−1 soil was 100% greater than the yield at 32–64 mg K kg−1 soil with zero Na. Sodium enhanced the function of K in translocation of assimilates to the fruit, as it was shown in increased fruit yields. A constant average number was obtained at 2–8 mg Na kg−1 soil and 64 mg K kg−1 soil, whereas the highest average fruit weight was observed at 2 mg Na kg−1 soil with 64 mg K kg−1 soil and decreased consistently up to 16 mg Na kg−1 soil with 64 mg K kg−1 soil. These results indicated that large fruit sizes were produced at 2 mg Na kg−1 soil with 64 mg K kg−1 soil while 8 mg Na kg−1 soil with 64 mg K kg−1 soil produced small sized fruits. A similar trend was observed for N content in the shoot of the tomato plant at 2–4 mg Na kg−1 soil with 32–64 mg K kg−1 soil, which increased fruit yields. The results of this study confirmed the observation of Aduayi and Olowoyo (Citation2003) and Idowu and Aduayi (Citation2003) that the application of low level of Na increased N nutrition of tomato plant and thereby enhanced fruit yield.
Citric acid content of fruit juice
Citric acid content of fruit juice was significantly (p<0.05) increased at 2–4 mg Na kg−1 soil and 0 mg K kg−1 soil (b). Increasing K levels resulted in a slight increase in citric acid content. Citric acid content increased significantly with interaction of Na at 2–4 mg kg−1 soil and 32 up to 64 mg K kg−1 soil. The addition of 8–32 mg Na kg−1 soil with 32–128 mg K kg−1 soil, significantly depressed the citric acid content. This result was in line with the report of Satti and Al-Yahyai (Citation1995), who noted that the citric acid content of tomato fruit generally decreased at high Na and K in the growth medium. In this study, the interaction of 4 mg Na kg−1 soil with 128 mg K kg−1 soil gave the highest value of citric acid content but the yield was reduced at this treatment level. The result was in agreement with Adams (Citation1991) who noted that the yield and quality of tomato are inversely related. Gould (Citation1974) observed a control of citric acid content of the tomato fruit juice by the cation:anion balance of plant cells. Papadopoulos et al. (Citation1999) related improved citric acid content by moderate Na and K levels to Na enhancing the effectiveness of K in activation of enzymes and synthesis of amino acids. The interaction of 2–4 mg Na kg−1 soil with 32–64 mg K kg−1 soil increased both the fruit yield and citric acid content, an important component of the shelf life quality of tomato fruits (Chapagain et al. Citation2003). The mechanism by which low rates of Na with moderate K increase the yield and quality of fruits is not clear and will require further investigation.
Relationship between fruit yield and other growth parameters as influenced by the application of Na and K
Shoot-K was significantly and positively correlated with shoot-N (r=0.43*) and -Ca (r=0.50*). These results agreed with Marschner (Citation1998), although the relationships were not very strong. Soil exchangeable Na and K were negatively but not significantly related to shoot-Na and -K, respectively. This result implies that plant roots selectively absorb nutrients in soil solution. According to Marschner (Citation1998), with increasing levels of Na and K in the soil, a point would be reached at which Na and K concentration in the soil could be toxic to the tomato plant. The ion toxicity effects would induce negative water potential around the root, thus, inhibiting nutrient uptake and transport to the shoot.
A significant positive correlation was observed between the number of leaves and soil-Na (r=0.51*) but negatively correlated with soil-K (r= − 0.07NS). Furthermore, the number of leaves correlated positively with shoot-Ca (r=0.60**) and shoot-N (r=0.46*). Lawlor and Milford (Citation1973) reported similar observations. The observed increased nitrogen and calcium content in the shoot, enhanced cell extension and reduced leaf senescence, thus increased the yields and quality of tomato fruits. Fruit yields best correlated with the number of leaves (r=0.65***), number of flowers (r=0.57**) and shoot-N (r=0.40*).
Conclusion
The soil used for the study contained total- and exchangeable- Na:K ratio of 1:1 and 1:3, respectively. The original K concentration in the soil was not sufficient for optimal yield and good quality tomato fruits. The addition of 32 mg Na kg−1 and 128 mg K kg−1 soil eliminated the toxicity effects observed at high levels of either Na or K. In conclusion, the inferences from this study, based on single and interactive effects of Na and K, showed that Na enhanced the water content of the tomato plant, improved root growth and increased number and area of leaves. Nitrogen, potassium and calcium content of shoot, and the phosphorus and magnesium content of root of the tomato plant were also increased. Overall, sodium and potassium at 2–4 mg Na kg−1 soil and 32–60 mg K kg−1 soil, at the ratio between 1:8 and 1:32 increased the growth, fruit yield and citric acid content of tomato fruit juice.
Acknowledgements
The authors gratefully acknowledge the financial assistance granted by the University Research Committee, Obafemi Awolowo University, Ile-Ife, Nigeria.
References
- Adams , P . 1986 . “ Mineral nutrition ” . In The tomato crop: A scientific basis for improvement , Edited by: Atherton , JG and Rudich , J . 281 – 334 . London/New York : Chapman and Hall .
- Adams , P . 1991 . Effects of increasing the salinity of the nutrient solution with major nutrients or sodium chloride on the yield, quality and composition of tomatoes grown in the rockwool . J Horticult Sci , 66 : 201 – 208 .
- Aduayi , EA and Onesirosan , PT . 1979 . Potassium-boron relationship in tomato under greenhouse conditions in a typical western Nigerian soil . Nigerian J Sci , 13 ( 1 & 2 ) : 415 – 423 .
- Aduayi , EA , Olayinka , A and Muoghalu , J . 1999 . The performance of maize (Zea mays L.) and cowpea (Vigna unguiculata [L.] Walp) varieties in soils from different agroecological zones in Southwestern Nigeria . Ife J Agricult , 20 ( 1 & 2 ) : 81 – 93 .
- Aduayi , EA and Olowoyo , BA . 2003 . Response of okra to sodium nutrition in an Ultisol . Moor J Agricult Res , 4 ( 1 ) : 8 – 12 .
- Akintunde , AY , Obigbesan , GO , Kim , SK and Akinrinde , EA . 2000 . Effects of nitrogen rates on grain yield response of maize varieties in four ecological zones of Nigeria . Nigerian J Soil Sci , 12 : 35 – 44 .
- Al-Harbi , AR . 1995 . Growth and nutrient composition of tomato and cucumber seedling as affected by sodium chloride salinity and supplementary calcium . J Plant Nutrit , 18 : 1403 – 1416 .
- Bahorun , T , Luximon-ramma , A , Crozier , A and Aruoma , OI . 2004 . Total phenol, flavonoid, proanthocyanidin and vitamin C levels and antioxidant activities of Mauritian vegetables . J Sci Food Agricult , 84 ( 12 ) : 1553 – 1561 .
- Besford , RT . 1978 . Effect of replacing nutrient (potassium by sodium) on uptake and distribution of sodium in tomato plants . Plant Soil , 50 : 399 – 409 .
- Blume , E and Jara , ASA . 2004 . Diseases on tomato cultivated in plastic greenhouse in four locations in the central region of Rio Grande do Sul, Brazil . Ciencia Rural , 34 ( 3 ) : 661 – 666 .
- Bernard , LO . 1965 . Determination of citric acid: Pentabromoacetone method. Physiological Chemistry , 1106 – 1107 . New York : Mcgraw-Hill .
- Beringer , H . 1987 . “ Recent data about P, K and Na fertilizer application to sugar beet in Central Europe ” . In Potash facts (1990) , Edited by: Gething , PA . 123 Switzerland : International Potash Institute. Pp .
- Bouyoucous , GH . 1962 . Hydrometer method improved for making particle size analysis of soils . Agronomy J , 54 : 464 – 465 .
- Bremner , JM . 1996 . “ Total nitrogen ” . In Methods of soil analysis: Chemical method Part 3 , Edited by: Sparks , DL . 1123 – 1184 . Madison, WI : SSSA, ASA .
- Chapagain , BP , Wiesman , Z , Zaccai , M , Imas , P and Magen , H . 2003 . Potassium chloride enhances fruit appearance and improves quality of fertigated greenhouse tomato as compared to potassium nitrate . J Plant Nutrit , 26 ( 3 ) : 643 – 658 .
- Dorais , M , Chretien , S and Gosselium , A . 2000 . High electrical conductivity and radiation-based water management improve fruit quality of greenhouse tomatoes grown in rock wool . Hortiscience , 35 ( 4 ) : 627 – 631 .
- Enwezor , WO , Udo , EJ , Ayotade , KA , Adepetu , JA and Chude , VQ . 1990 . A review of soil and fertilizer use research in Nigeria , 109 – 200 . Lagos : Federal Ministry of Agriculture, Water resources and Rural Development .
- Ergun , M , Sargent , SA and Huber , DJ . 2006 . Postharvest quality of grape tomatoes treated with I-methylcylopropene at advanced ripeness stages . Hortiscience , 41 ( 1 ) : 183 – 187 .
- Gould AG. 1974 . Tomato, production, processing and quality evaluations . Westport, CT : Avi. Publishing Company Inc . 445 pp.
- Hanks , RJ , Holmes , WE and Tanner , CB . 1954 . Field capacity approximation based on the moisture-transmitting properties of the soil . Soil Sci Soc Am Proc , 18 : 252 – 254 .
- Ho , CI and Hewitt , JD . 1986 . “ Fruit development ” . In The tomato crop: A scientific basis for improvement , Edited by: Atherton , JG and Rudich , J . 201 – 239 . New York : Chapman and Hall .
- Idowu MK. 2005 . The effects of sodium and potassium on growth, yield, nutrient content and nutritional quality of tomato (Lycopersicon lycopersicum [L.] Karst) . PhD Thesis. Obafemi Awolowo University, Ile-Ife, Nigeria. pp 1–147 .
- Idowu , MK and Aduayi , EA . 2003 . Assessing the nutritional quality of sodium in combination with potassium for the growth of tomato in soil culture . Moor J Agricult Res , 4 ( 2 ) : 183 – 190 .
- Idowu , MK and Aduayi , EA. 2006 . Effects of sodium and potassium on water content and yield of tomato in southwestern Nigeria . J Plant Nutrit , 29 : 2131 – 2145 .
- International Fertilizer Development Centre (IFDC) . 1984 . The vital role of potassium in tropical agriculture. The present position, future potential and constraints to progress . Muscle Shoals, Alabama, USA: IFDC. In: Literature review on soil fertility investigations in Nigeria. Federal Ministry of Agriculture and Natural Resources (1990). pp 1–47 .
- International Institute of Tropical Agriculture (IITA) . 1979 . Chemical analysis of plant tissues. Selected methods for soil and plant analysis . Manual Series No. 1. Ibadan, Nigeria. 23 pp .
- Jeschke , WD . 1977 . K+ Na+ exchange and selectivity in barley root cells: Effects of Na+ on the Na+ fluxes . J Experim Botany , 32 : 1257 – 1272 .
- Kaur , C and Kapoor , HC . 2001 . Antioxidants in fruits and vegetables – the millenium's health . Int J Food Sci Technol , 36 : 703 – 725 .
- Klesta , EJ and Bartz , JK . 1996 . “ Quality assurance and quality control ” . In Methods of soil analysis. Part 3. Chemical methods , Edited by: Sparks , DL . 19 – 48 . Madison, WI : SSSA, ASA .
- Kuo , S . 1996 . “ Phosphorus ” . In Methods of soil analysis. Part 3. Chemical methods , Edited by: Sparks , DL . 869 – 920 . Madison, WI : SSSA, ASA .
- Lavelli , V , Peri , C and Rizzolo , A . 2000 . Antioxidant activity of tomato products as studied by model reactions using xanthine oxidase, myeloperoxidase, and copper-induced lipid peroxidation . J Agricul Food Chem , 48 : 1442 – 1448 .
- Lawlor , DW and Milford , GFJ . 1973 . The effects of sodium on growth of water stressed sugar beet . Ann Botany , 37 : 597 – 604 .
- Liang , Y . 1999 . Effects of silicon on enzyme activity and sodium, potassium and calcium concentration in barley under salt stress . Plant Soil , 209 : 217 – 224 .
- Malash , N , Ghaibeh , A , Abdelkarim , G , Yeo , A , Flowers , T , Ragab , R , Cuartero , J , Aksoy , U , Anac , D , Anac , S , Beltrao , J , Ben-Asher , J , Cuartere , J , Flowers , TJ and Hepaksoy , S . 2002 . Effect of irrigation water salinity on yield and fruit quality of tomato . Acta Horticulturae , 573 : 423 – 434 .
- Marschner , H . 1998 . Beneficial elements. Mineral nutrition of higher plant , 2nd ed , 403 – 417 . New York : Academic Press .
- Marschner , H and Possingham , JV . 1975 . Effects of K+ and Na+ on growth of leaf discs of sugar beet and spinach . Z pflanzanphysiol , 75 : 6 – 16 .
- Mengel , K and Viro , M . 1974 . Effects of potassium supply on the transport of photosynthates to the fruits of tomato (Lycopersicon esculentum) . Plant Physiol , 30 : 295 – 300 .
- Moretti , CL , Sargent , SA , Huber , DJ , Calbo , AG and Puschmann , R . 1998 . Chemical composition and physical properties of pericarp, locule, and placental tissues of tomatoes with internal bruising . J Am Soc Horticult Sci , 123 ( 4 ) : 665 – 670 .
- Nicoli , MC , Anese , M and Parpinel , M . 1999 . Influence of processing on the antioxidant properties of fruits and vegetables . Trends Food Sci Technol , 10 : 94 – 100 .
- Nunes , MA , Dias , MA , Correia , M and Oliveira , MM . 1984 . Further studies on growth and osmo-regulation of sugar beet leaves under low salinity conditions . J Experim Botany , 35 : 322 – 331 .
- Odunze AC Esu IE 1989 . Degraded soils of Northern Nigeria: A case study of the Bakura area in Sokoto State, Nigeria . Proceedings of the 17th Annual Conference of the Soil Science Society of Nigeria. Nsukka, Anambra State, Nigeria. pp 61–69 .
- Okusami , TA and Oyediran , GO . 1985 . Slope-soil relationships on an aberrant Toposeqence in Ife area of southwestern Nigeria: Variabilities in soil properties . Ife J Agricult , 7 ( 1 & 2 ) : 1 – 15 .
- Oyewole OI 1988 . The effects of the interaction between boron and calcium on the mineral nutrition and growth of ‘Ife plum’ tomato . MSc Thesis. Faculty of Agriculture, Department of Soil Science. Ile-Ife, Nigeria: Obafemi Awolowo University . pp 25 – 39 .
- Oyewole , OI and Aduayi , EA . 1992 . Evaluation of the growth and quality of the ‘Ife plum’ tomato as affected by boron and calcium fertilization . J Plant Nutrit , 15 ( 2 ) : 199 – 210 .
- Papadopoulos , AP , Pararajusingham , S and Hao , X . 1999 . Fertilizer substitution in hydroponically grown greenhouse tomatoes . Hortcult Technol , 9 ( 1 ) : 59 – 65 .
- Peter , OM . 1993 . Sodium and potassium. Environmental chemistry , 2nd ed , 184 – 192 . London : Chapman and Hall .
- Phillips , CJC and Chiy , C . 1995 . “ Sodium fertilizers ” . In Sodium in agriculture , Edited by: Phillips , CJC and Chiy , C . 71 – 81 . Hungary : Chalcombe Publisher .
- Piper , CS . 1944 . Dry ashing. Soil and plant analysis , 221 – 222 . New York : Interscience Publishers Inc .
- SAS Institute . 1990 . SAS users’ guide: Basics . Cary, NC : SAS Institute .
- Satti , SME and Al-Yahyai , RA . 1995 . Salinity tolerance in tomato: Implications of potassium, calcium and phosphorus . Communic Soil Sci Plant Analysis , 26 ( 17 & 18 ) : 2749 – 2760 .
- Soil Survey Staff . 1975 . Soil taxonomy. A basic system of soil classification for making and interpreting soil surveys . USDA Agricultural Handbook. 436 pp .
- Smyth , AJ and Montgomery , RF . 1962 . The soil and land use of central western Nigeria , 265 Ibadan, , Nigeria : The Government Printer .
- Steel , RGD and Torrie , JH . 1980 . Principles and procedures of statistics – A biometrical approach , 2nd ed , 627 London : McGraw-Hill .
- Steinmetz , KA and Potter , JD . 1996 . Vegetable, fruit and cancer epidemiology . Cancer Causes Control , 2 : 325 – 351 .
- Thomas , GW . 1996 . “ Soil pH and soil acidity ” . In Methods of soil analysis. Part 3: Chemical methods , Edited by: Sparks , DL . 475 – 490 . Madison, WI : SSSA, ASA .
- Usherwood , NR . 1985 . “ The role of potassium in crop quality ” . In Potassium in agriculture , Edited by: Robert , DM . 501 – 502 . Madison, WI : SSSA .
- Walkley , A and Black , IA . 1934 . An examination of the Degtareff method for determining soil organic matter and proposed modification of the chromic acid titration method . Soil Sci , 37 : 29 – 38 .
- Wybenga JM 1975 . Investigations with radioactive sodium . PhD Thesis. Kantoordruk de Coede, Wageningen. Holland. 211 pp .
- Wyn Jones , RG , Brady , CJ and Speirs , J . 1979 . “ Ionic and osmotic relations in plant cells ” . In Recent advances in the biochemistry of cereals , Edited by: Laidman , DL and Wyn Jones , RG . 63 – 103 . London : Academic Press .