Abstract
Inoculation of sugarcane plants, cv. Jaronu 60-5, with teliospores of Ustilago scitaminea increased the production of sugarcane glycoproteins of high molecular mass (HMMG) and decreased the amount of those of mid-molecular mass (MMMG) recovered from stalks cell-free extracts. Whereas sugarcane glycoprotein of healthy plants totally inhibited the production of fungal mycelium from teliospores, mycelium growth was slightly affected by MMMG, and drastically diminished by HMMG obtained from inoculated plants. The adhesion of sugarcane glycoproteins to fungal teliospores produced cell aggregation. This effect was clearly reduced by incubating teliospores in buffer containing HMMG or MMMG previously digested with invertase. Sugarcane arginase associated to MMMG was retained by teliospores, whereas the enzymatic activity associated to HMMG from inoculated plants increased. Chitinase was not significantly retained by teliospores. Only HMMG and MMMG obtained from healthy plants were able to inhibit cell polarity in some extent. Smut induced changes in the composition of HMMG and MMMG. Some of these new glycoproteins are able to inhibit cell polarity. Since receptors, isolated from the cell wall of smut teliospores, were eluted from activated agarose beads by N-acetyl-D-glucosamine, we concluded that the peptide fraction of HMMG and MMMG bind to this amino sugar in the polysaccharide moiety of smut ligands.
Introduction
Plants in general – and sugarcane in particular – have developed different defensive mechanisms to fight invasion by pathogenic organisms. Some authors (Waller Citation1970; Da Gloria et al. Citation1999) speculate that the varietal resistance of sugarcane to smut is determined by some structural characteristics of buds. However, many reports suggest that resistance is based on chemical rather than bud morphology. Lloyd and Naidoo (Citation1983) isolated glycosidic substances from fresh scales and established a linear correlation between resistance rating to smut and the concentration of chemicals in scales. Piñón et al. (Citation1999) observed differences in polyamine content of sugarcane juices obtained from sensitive or resistant cultivars of sugarcane after experimental inoculation with Ustilago scitaminea teliospores.
There is a plethora of molecules involved in plant defence mechanisms but several proteins play a role in the process of resistance against pathogens. The accumulation glycoproteins causes cell wall toughening and an increase of peroxidase activity that may stimulate the lignification of the host cell wall (Lebeda et al. Citation2001). Other proteins, such as some enzymes involved in the pathway of lignin biosynthesis, are involved in the resistance of sugarcane to pathogens (Ramos et al. Citation2001).
Protease inhibitors are proteins that regulate proteolytic activities and they are part of plant defensive mechanism against the invasion by pathogens. It has recently documented that a protease inhibitor from sugarcane over-expressed in Escherichia coli inhibits the growth of pathogenic sugarcane fungi (Soares-Costa et al. Citation2002). Furthermore, contact between this protein and spores of Trichoderma reesei inhibits the germination of the filamentous fungus spores.
This protease inhibitor seems to act directly on fungal proteases, impeding the normal development of hyphae. Raggi (Citation2000) found that the increase of the amount of hydroxyproline-rich glycoproteins in the cell wall of tobacco leaves induced by infection with the necrotic strain of Y potato virus, potentiate general resistance against powdery mildews.
Sugarcane also produces two different pools of glycoproteins containing a heterofructan as glycidic moiety and tentatively described as high molecular mass (HMMG) and mid-molecular mass (MMMG) glycoproteins (Legaz et al. Citation1995). Analysis of both HMMG and MMMG by capillary electrophoresis reveals that MMMG fraction contains two cationic and four anionic components whereas only one cationic and four anionic proteins are separated from the HMMG fraction (Legaz et al. Citation1998). These glycoproteins can be considered as factors of biological resistance to smut (Martínez et al. Citation2000) since their amount increases, after infection with smut teliospores, in resistant but decreases in susceptible varieties after infection with smut teliospores. In addition, Fontaniella et al. (Citation2002) found that these glycoproteins, preferently those defined as anionic peptides, selectively bind to smut teliospores. After binding, cell aggregation occurs in parallel to a loss of the germination ability of recruited teliospores and the emergence of the germinating tube is completely impeded.
Sugarcane smut is caused by the dimorphic basidiomycete Ustilago scitaminea. The characteristic symptom of culmicolous smut is the formation of a long whip-like sorus in which diploid, airborne teliospores are produced (Lee-Lovick Citation1978). Spore germination originates a pro-mycelium which gives rise to four haploid sporidia after meiosis. When two compatible sporidia form germ tubes that fuse, a dikaryotic, infecting mycelium is produced (Alexander and Srinivasan Citation1966). Hyphal growth occurs throughout the infected plant but mostly in the parenchyma cells of the internode. The formation of the dikarion is readily recognized in vitro when culture changes from a yeast-like appearance to white, fuzzy mycelial growth (Schenk Citation1999).
Previous to or during spore germination, one of the major changes concerns cytoplasm polarization, a process mediated by actin (Evangelista et al. Citation2003; Magdalena et al. Citation2003; Wedlich-Soldner et al. Citation2003), that is essential for fungal tip growth. Ustilago maydis, the causal pathogen of corn smut disease, divides by budding at its unicellular yeast-like stage. During budding, the actin cytoskeleton polarizes at sites of active growth (Banuett and Herskowitz Citation2002). Because during the bud growth, the bud tip is the site of the incorporation of new cell wall material, the concentration of actin at this site suggests that actin plays an active role in the secretion of cell wall polymers. Assembly and disassembly of actin filaments is also on the basis of cell motility (Pollard and Borisy Citation2003).
On the basis of these results, assuming that cell polarization is required for teliospores germination, we hypothesize that the inhibition of smut teliospores germination by sugarcane glycoproteins, HMMG and MMMG, could be specifically related to actin polymerization.
Material and methods
Plant material
Field-grown Saccharum officinarum L., cv. Jaronu 60-5, was used throughout this work. Teliospores of Ustilago scitaminea Syd., agent of sugarcane smut, were collected from whips of infected plants in experimental crops of the National Institute for Sugarcane Investigation (INICA) in Matanzas, Cuba.
Preparation of soluble glycoproteins
Stalks from 22 months-old inoculated and healthy sugarcane plants were mechanically crushed immediately after cutting and the raw juice was centrifuged at 5000 g for 15 min at 2°C. The pellet was discarded and the supernatant was filtered through filter paper. Centrifuged juice was chromatographed through a Sephadex G-10 column (14.5×2.5 cm i.d.) equilibrated and eluted with 10 mM phosphate buffer, pH 6.8. Fractions 1–20 (1.0 ml each) were discarded, fractions 20–40 were filtered through a Sephadex G-50 column (29×2.5 cm i.d.) equilibrated as described above. Fractions 40–70 contained HMMG whereas fractions 70–115 MMMG proteins (de Armas et al. Citation1999). Eluted fractions were monitored for carbohydrates according to Dubois et al. (Citation1956).
Effect of sugarcane glycoproteins on smut teliospores germination
Both HMMG and MMMG from inoculated and healthy plants were newly chromatographed through a column of Sephadex G-100 (103×2.5 cm i.d.) equilibrated and eluted with 10 mM phosphate buffer (pH 6.8). On the other hand, 20 mg teliospores were floated for 2 h at 40°C on 2.0 ml of HMMG or MMMG solution, obtained from inoculated and healthy plants, containing about 0.3 mg protein. Spore suspensions were then centrifuged at 10000 g for 15 min at 2°C. Pellets containing teliospores were separated in two aliquots.
Aliquot one was resuspended in 100 m of sterile medium (Lilly and Barnett Citation1951) and incubated at 38°C for 14 days to study germination. The second aliquot was incubated for 2 h at 40°C in 5.0 ml 50 mM sucrose, centrifuged as described and the supernatant assayed for arginase activity. Supernatants were also assayed for this enzyme activity and then filtered through the same Sephadex G-100 column. Elution of glycoproteins was monitored in the different fractions (5.0 ml each) by the method of Warburg and Christian (Citation1941). Urease (480 kDa), glutamic acid decarboxylase (310 kDa), bovine serum albumin (66 kDa), ovoalbumin (45 kDa), peroxidase (40 kDa) and cytochrome (12 kDa) were used as standards (all from Serva Feinbiochemica GmbH & Co., Heidelberg, Germany).
Detection of proteins by capillary electrophoresis
Zone electrophoresis was performed using a Spectraphoresis 500 system from Spectra-Physics (Fremont, CA, USA). Microbore fused-silica tubing of a total length of 70 cm, a separation length of 63 cm and with 75 µm internal diameter and 190 µm outer diameter, coated with polyimide (Scientific Glass Engineering, Milton Keynes, UK) were used (the capillary was encased for easy handling). On-line detection was performed with a variable-wavelength UV-Vis detector of 6 nm band width (Spectra-Physics, Fremont). Detection of protein was monitored at 280 nm and electrophoregrams were recorded using a SP 4290 integrator (Spectra-Physics, Fremont).
New capillaries were conditioned with 1 M NaOH for 10 min at 60°C, 0.1 M NaOH for 10 min at 60°C and Milli-Q grade water for 10 min at 60°C. Equilibration of the capillary was then performed by washing with 25 mM sodium borate buffer, pH 9.2 for 30 min at 25°C and finally with the same buffer for 30 min at 25°C under applied voltage of 15 kV. Regeneration of the capillary surface between runs was performed by rinsing it in the following sequence: 0.1 M NaOH for 5 min, Milli-Q grade water for 5 min and 25 mM sodium borate buffer, pH 9.2 for 15 min. The buffer used as electrolyte was 25 mM sodium borate buffer, pH 9.2 (Legaz and Pedrosa Citation1993).
Effect of glycoproteins on smut teliospore aggregation
Samples of smut teliospores (2.0 mg) were floated on 2.0 ml of HMMG or MMMG (0.3 mg protein) obtained from healthy and inoculated plants. In addition, both HMMG and MMMG were incubated for 6 h at 45°C (de Armas et al. Citation1992) with commercially purified invertase (from Sigma Chemical Co., St Louis, MO, USA) at pH 4.5 in 20 mM sodium citrate/citric acid buffer, to extensively hydrolyze the glycosidic moiety of both fractions (mainly composed by β-1,2 glycosidic bonds between fructose units) and to verify the participation of hydrolyzed molecules in binding sugarcane glycoproteins to smut teliospores.
Alternatively, samples of 1.0 mg of spores were resuspended in 1.0 ml of different glycoprotein fractions obtained after filtration of both HMMG and MMMG through a Sephadex G-100 column, as described previously. After 1 h of incubation at 40°C, spores were observed by a light microscope (Zeiss optical inverto-microscope). Photographs were taken by a Nikon Coolpix 5000 digital camera.
Cell polarization and visualization of the actin cytoskeleton
Samples of 2 mg of smut teliospores were incubated in a shaking bath at 40°C for 2 h with different glycoprotein fractions obtained by filtration through a Sephadex G-100 column as described above. Spores were recovered by centrifugation at 10,000 g for 15 min at 2°C, and supernatants used for subsequent separation in the same column. Treated spores were used to inoculate Erlenmeyer flasks containing 100 ml of sterile medium (Lilly and Barnett Citation1951), and incubated at 38°C for 24 h. Spores were then processed for visualization of the actin cytoskeleton by fluorescence microscopy as described by Heath (Citation1987). Fluorescein isothiocyanate-conjugated phalloidin (Sigma Chemical Co.) was used to label polymerized (F-) actin. Teliospore cell walls were permeabilized using 2% (v/v) isopropanol instead of Triton X-100, which only permeabilizes cell membranes. As a negative control, teliospores were treated as above in the absence of sugarcane glycoprotein fractions. The stained cells were observed using an Olympus DP50 fluorescence microscope adapted with a CCD camera capturing images provided of Viewfinder Lite program.
Assay of arginase activity
Both HMMG and MMMG were assayed for arginase activity according to Legaz and Vicente (Citation1982), including crystalline urease in the reaction mixtures. These contained, in a final volume of 3.0 ml, 10 µmol Tris-HCl buffer, pH 9.1, 7.5 µmol maleic acid, 5.0 µmol Mn2 + (as manganese sulfate), 0.4 µmol arginine, 8.1 mg crystalline urease, and 10.0 µg sugarcane protein. Reaction was carried out at 37°C for 30 min and was stopped by adding 0.5 ml of a saturated potassium carbonate solution. The amount of ammonia produced was measured by the Conway (Citation1962) microdiffusion method. Specific activity was defined as µmol of ammonia produced per mg protein per min.
Chitinase activity determination in sugarcane juices
To test the existence of chitinase activity in juices or in glycoprotein fractions derived from sugarcane plants, commercial chitin (Sigma Chemical Co.) was used as a substrate. A volume of 0.2 ml extract was added to 2.0 ml of 0.05% chitin 50 mM acetate buffer (pH 5.0) at room temperature. After 30 min, the reaction was stopped by heating at 100°C for 5 min (Hou et al. Citation1998). The increased reducing ends, released by chitinase, were determined according to the method of Imoto and Yagishita (Citation1971). The extracts added to 2.0 ml of the same acetate buffer were used as controls for each reaction.
Purification of teliospores cell wall receptors for HMMG and MMMG
Affinity chromatography in 8% cyanogen-bromide agarose (Sigma Chemical Co.) was used to isolate cell wall receptors of both MMMG and HMMG. Two beads of 8% cyanogen-bromide agarose (5.0 cm×1.0 cm i.d.) were prepared according to Matsumoto (Citation1985) and then 4.0 ml of MMMG were added to one bead, while 4.0 ml of HMMG was loaded onto the second bead. Columns were closed and stored for 2 h at room temperature. During this time, glycoproteins were joined to activated agarose. After this, beads were washed with 10 ml of distilled water (Legaz et al. Citation2004). Then, 3.0 ml of cell wall proteins of X. albilineans were added onto the bead and maintained in contact with the matrix for 2 h at room temperature. After this, beads were washed with 100 ml of distilled water to remove non-retained bacterial proteins. Fractions of 3.0 ml were assayed for their protein content by the Warburg and Christian method (1941). Later, since glycidic moiety of HMMG and MMMG is composed by fructose and galactitol (Millanes et al. Citation2005), 100 ml of 100 mM sucrose and 100 mM galactitol were added to each bead to desorbe retained cell wall receptors. Eluates of 3 ml were assayed for their protein content by the Warburg and Christian (Citation1941) method.
Results
Production of sugarcane glycoproteins and their effect on the growth of fungal mycelium
Inoculation of sugarcane plants cv. Jaronu 60-5, with teliospores of U. scitaminea increased the production of HMMG and decreased the amount of MMMG recovered in juices (A). Production of fungal mycelium by 2.0 mg teliospores on Lilly-Barnett medium, containing HMMG or MMMG, was slightly affected by MMMG whereas obtained from inoculated plants whereas drastically diminished (about 50%) on media containing HMMG. Both HMMG and MMMG obtained from healthy plants impaired fungal growth (B). This effect could be related to the amount of sugarcane glycoprotein sequestered by teliospores during incubation (C). The amount of HMMG and MMMG sequestered by teliospores from purified juices of healthy plants was higher than the amount of retained glycoproteins from juices of inoculated plants
Figure 1. (A) Elution profile of juices obtained from healthy () or inoculated (•) plants for the separation of a mixture of HMMG and MMMG by filtration through Sephadex G-50. (B) Growth of mycelium from 2.0 mg teliospores of Ustilago scitaminea floated for 14 days on sterile Lilly and Barnett (Citation1951) medium, previously in contact for 2 h at 38°C with 2 ml distilled water containing 0.3 mg of HMMG and MMMG from stalks of healthy and inoculated plants. Values are the mean of three replicates. Bars give the standard error. (C) Protein content of glycoprotein fractions, before and after incubation with smut teliospores. Numbers indicated per cent of protein retention by teliospores. Values are the mean of three replicates. Bars give the standard error.
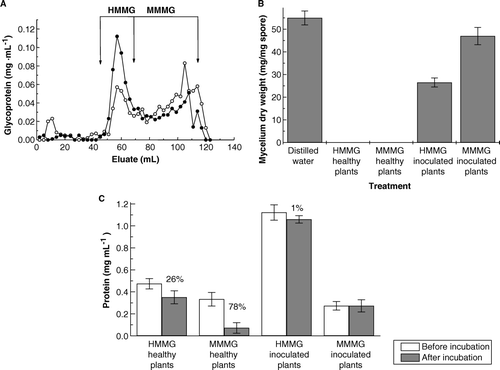
The adhesion of sugarcane glycoproteins to fungal teliospores produced aggregation, as it is shown in . The recruitment of smut teliospores dispersed in distilled water containing HMMG or MMMG from healthy plants (B and 2C) was higher than the one observed in inoculated plants (D and 2E). This effect was clearly reduced using invertase-digested HMMG or MMMG in 20 mM citrate buffer, pH 4.5 (instead of non-digested HMMG or MMMG indistilled water) to incubate teliospores (F–2J) to incubate teliospores containing previously digested with invertase, as described in Methods. This suggests that the glycidic moiety of sugarcane glycoproteins, containing β-D-fructofuranosyl-1,2-β-D-fructose, may be involved in the process of cell aggregation since the extensive hydrolysis of β-1,2 bond impedes cell adhesion.
Figure 2. Teliospores of Ustilago scitaminea dispersed and incubated for 1 h at 38°C in distilled water (A) or in 2.0 ml distilled water containing 0.3 mg of HMMG (B) or of MMMG (C) obtained from healthy plants, or in 0.3 mg of HMMG (D) or in 0.3 mg of MMMG (E) obtained from inoculated plants. Alternatively, teliospores were dispersed and incubated for 1 h at 38°C in citrate buffer (F) or in 2.0 ml of the same buffer containing 0.3 mg of HMMG (G) or MMMG (H) obtained from healthy plants; or in 0.3 mg of HMMG (I) or of MMMG (J) obtained from inoculated plants, and all of them previously digested with invertase. Arrows indicate cell aggregation. Bars = 20 µm.
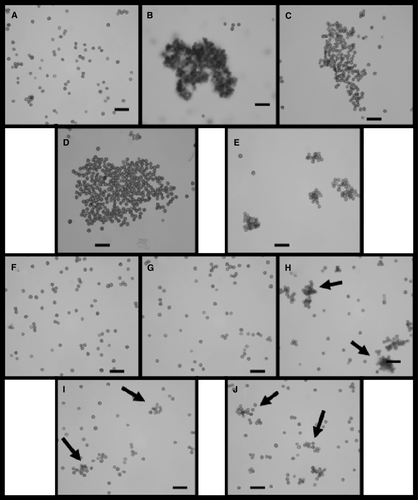
Alteration of the enzymatic activitiy pattern of sugarcane glycoproteins upon inoculation with smut teliospores
Inoculation of sugarcane plants with smut teliospores decreased arginase activity associated to HMMG fraction. However, this activity increased in the MMMG fraction after inoculation (A). Both in healthy and infected plant juices MMMG-associated (and to a lower extent HMMG-associated) sugarcane arginase was substantially retained by teliospores after incubation whereas in the case of HMMG obtained from inoculated plants increased (B). Chitinase activity only appeared in HMMG obtained from inoculated plants (C) and slightly decreased after incubation with teliospores (D).
Figure 3. (A) Arginase activity of both HMMG and MMMG from healthy and inoculated plants. (B) Arginase activity of both HMMG and MMMG, from healthy and inoculated plants, before and after incubation with smut teliospores. (C) Chitinase activity of both HMMG and MMMG from healthy and inoculated plants. (D) Chitinase activity of both HMMG and MMMG, from healthy and inoculated plants, before and after incubation with smut teliospores. Values are the mean of three replicates. Bars give the standard error.
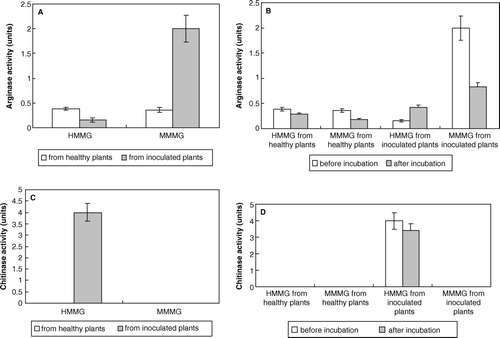
Effect of sugarcane glycoproteins on teliospore polarity
As shown in , only HMMG and MMMG obtained from healthy plants were able to inhibit cell polarity. Two proteins were identified in HMMG fraction obtained from healthy plants with molecular masses of 187 kDa (peak a) and 141 kDa (peak b), respectively, that were significantly retained by smut teliospores after incubation (A). Peak a did not affect teliospores polarity whereas peak b inhibited it. On the other hand, three different glycoproteins from MMMG fraction apparently interacted with teliospores. These glycoproteins were separated by size-exclusion chromatography using a Sephadex G-100 column. About a 68% of the first one (peak c in B), with a molecular weight of 90 kDa, was removed from the media after incubation with teliospores but it did not affect polarization significantly. The other two peaks, d (molecular mass of 57 kDa) and e (molecular mass of 13 kDa), were also similarly retained. The first one (peak d) inhibited, but the second (peak e) did not affect polarization.
Figure 4. Polarization of smut teliospores incubated with or without HMMG or MMMG obtained from both healthy or inoculated plants. Values are the mean of three replicates. Bars give the standard error.
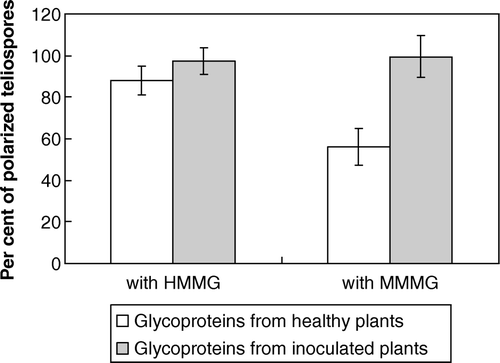
Figure 5. Elution profiles through a Sephadex G-100 column of glycoprotein fractions before (•) and after () incubation with smut teliospores, corresponding to HMMG from healthy (A) and inoculated (B) plants and MMMG from healthy (C) and inoculated (D) plants. In E, a semi-logaritmic representation of the relationship between log of molecular mass of several markers and elution volume is shown. Molecular markers are: 1 = urease (480 kDa); 2 = glutamic acid decarboxylase (310 kDa); 3 = bovine serum albumin (66 kDa); 4 = ovoalbumin (45 kDa); 5 = peroxidase (40 kDa) and 6 = cytochrome c (12 kDa).
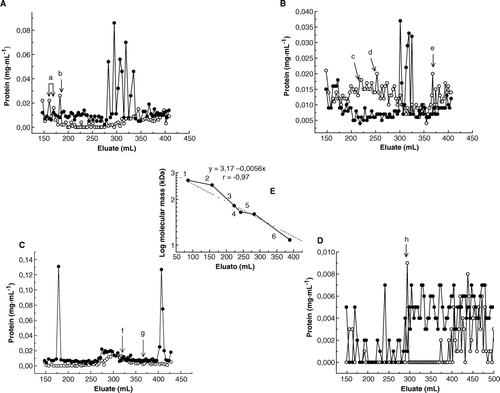
Only two peaks of glycoproteins from the fraction HMMG were partially retained by teliospores (32% and 14%, respectively), those called peaks f (molecular mass 25 kDa) and g (20 kDa), as shown in C. The binding of the 25 kDa protein (peak f) to teliospores increased cell polarity whereas polarity was inhibited after teliospores binding to the 20 kDa protein (peak g). Two new peaks appeared after incubation, peaks h and i, included in the MMMG fraction. Peak h inhibited teliospore polarity whereas peak i probably contained proteins secreted by teliospores to the medium (D).
Separation of glycoproteins interacting with teliospores by capillary electrophoresis (CE)
Peak a (A) of HMMG obtained from healthy plants did not change the pattern of teliospore polarization, as shown in A and 6B. This fraction was composed by only one glycoprotein (C), as revealed by CE, with a migration time value of 25.8 min (µa=1.43·10−3 cm2s−1V−1), that was also recovered after incubation of this fraction with teliospores (D), showing a slightly modified migration time value of 26.92 min (µa=1.38·10−3 cm2s−1V−1). However, part of this glycoprotein could be bound to teliospores, as deduced from the decrease of area counts in a 45% after incubation.
Figure 6. (A) Polarization of smut teliospores incubated for 2 h at 40°C in 10 mM phosphate buffer or (B) in 10 mM phosphate buffer plus for 24 h in Lilly-Barnett medium at 38°C peak a of HMMG from healthy plants (A), and after this, for 24 h in Lilly-Barnett medium at 38°C. Bars = 5.0 µm. (C) Electropherogram of HMMG peak a from healthy plants, obtained by filtration of HMMG pool through Sephadex G-100. (D) Electropherogram of proteins from peak a recovered after incubation with smut teliospores. Number near each peak indicates the migration time of the protein in min.
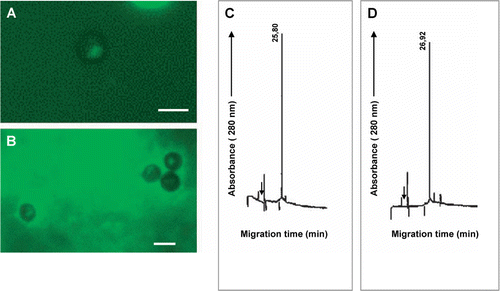
Incubation of peak b with teliospores induced the appearance of multifocal polarization revealing an anomalous distribution of F-actin (, compare A and B). Two peaks appeared in CE (C), displaying migration time values of 13.12 min (µa=2.56·10−3 cm2s−1V−1) and 35.20 min (µa=9,54·10−4 cm2s−1V−1), corresponding to peak b and they completely disappeared after incubation (data not shown), suggesting interaction of the protein with teliospores. Only peak d from MMMG (B and 8C, migration time value = 27.54 min, µa=1.22 10−3 cm2 s−1 V−1) inhibited actin polarization (, compare A and B), and it was completely retained by teliospores since it disappeared from the capillary electrophoresis profile after incubation (C and 8D). Peaks c and e did not show any interaction with teliospores (data not shown).
Figure 7. (A) Polarization of smut teliospores incubated for 2 h at 40°C in 10 mM phosphate buffer and after this, for 24 h in Lilly-Barnett medium at 38°C. (B) Polarization of smut teliospores incubated for 2 h at 40°C in 10 mM phosphate buffer containing peak b of HMMG from healthy plants (A) and after this, for 24 h in Lilly-Barnett medium at 38°C. Bars = 5.0 µm. (C) Electropherogram of HMMG peak b from healthy plants, obtained by filtration of HMMG pool through Sephadex G-100. No peak was obtained after capillary electrophoretic separation of peak b after incubation with teliospores (data not shown). Number near each peak indicates the migration time of the protein in min.
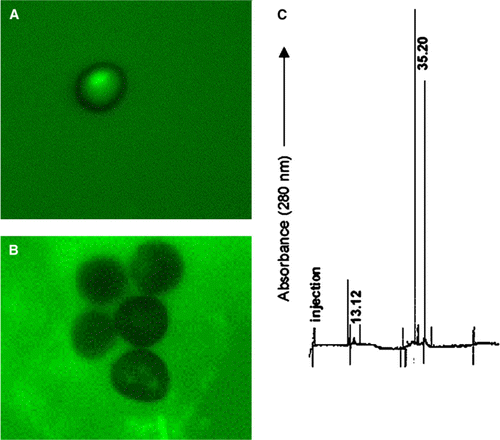
Figure 8. (A) Polarization of smut teliospores incubated for 2 h at 40°C in 10 mM phosphate buffer and after this, for 24 h in Lilly-Barnett medium at 38°C. (B) Polarization of smut teliospores incubated for 2 h at 40°C in 10 mM phosphate buffer containing peak d of HMMG from inoculated plants (B) and after this, for 24 h in Lilly-Barnett medium at 38°C. Bars = 5.0 µm. (C) Electropherogram of HMMG peak d from inoculated plants, obtained by filtration of HMMG pool through Sephadex G-100. (D) Electropherogram of proteins from peak d recovered after incubation with smut teliospores. Number near each peak indicates the migration time of the protein in min.
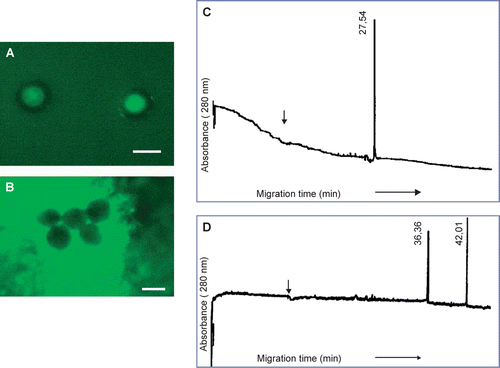
Peak g, alone from HMMG, disorganized actin cytoskeleton (A and 9B). This peak was composed by two main glycoproteins with migration time values of 12.64 min (µa=3.33·10−3 cm2s−1V−1) and 29.12 min (µa=1.45·10−3 cm2s−1V−1), respectively, and a third, minor peak at 29.72 min (µa=1.13·10−3 cm2s−1V−1) (C). These three components were retained by teliospores after incubation, although two new peaks appeared, probably proteins secreted by teliospores, with migration time values of 37.5 min (µa=8.47·10−4 cm2s−1V−1) and 45.13 min (µa=7.04·10−4 cm2s−1V−1), respectively (D).
Figure 9. (A) Polarization of smut teliospores incubated for 2 h at 40°C in 10 mM phosphate buffer and after this, for 24 h in Lilly-Barnett medium at 38°C. (B) Polarization of smut teliospores incubated for 2 h at 40°C in 10 mM phosphate buffer containing peak g of MMMG from healthy plants (C) and after this, for 24 h in Lilly-Barnett medium at 38°C. Bars = 5.0 µm. (C) Electropherogram of MMMG peak g from healthy plants, obtained by filtration of HMMG pool through Sephadex G-100. (D) Electropherogram of proteins from peak g recovered after incubation with smut teliospores. Number near each peak indicates the migration time of the protein in min.
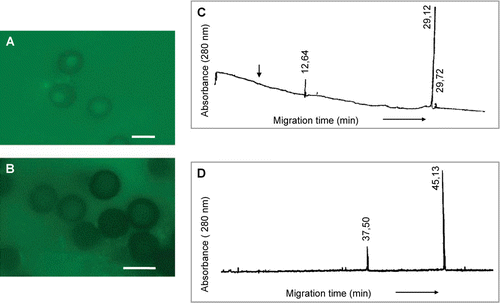
Finally, peak h identified from MMMG isolated from inoculated plants inhibited actin polarization and it was composed by a main peak of protein with a migration time value of 12.24 min (µa=3.10·10−3 cm2s−1V−1) and a minor peak at 24.86 min (µa=1.01·10−3 cm2s−1V−1) (C), which was retained by teliospores. Two peaks of fungal protein appeared after incubation with migration time values of 30.49 min (µa=1.21·10−3 cm2s−1V−1) and 37.80 min (µa=9.75·10−4 cm2s−1V−1) (D).
Figure 10. (A) Polarization of smut teliospores incubated for 2 h at 40°C in 10 mM phosphate buffer and after this, for 24 h in Lilly-Barnett medium at 38°C. (B) Polarization of smut teliospores incubated for 2 h at 40°C in 10 mM phosphate buffer containing peak h of MMMG from inoculated plants (D) and after this, for 24 h in Lilly-Barnett medium at 38°C. Bars = 5.0 µm. (C) Electropherogram of MMMG peak h from inoculated plants, obtained by filtration of MMMG pool through Sephadex G-100. (D) Electropherogram of proteins from peak h recovered after incubation with smut teliospores. Number near each peak indicates the migration time of the protein in min.
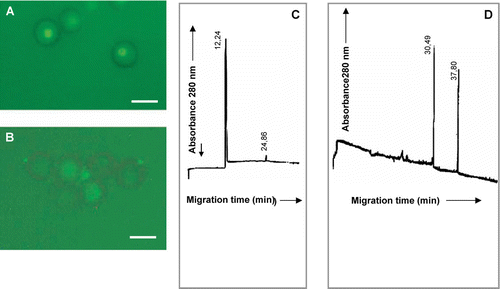
Identification of cell wall ligands for sugarcane glycoproteins in smut teliospores
When HMMG obtained from healthy plants were bound to activated agarose, some teliospore proteins (∼11%) were not retained on the column (A). The electropherogram of this peak showed three different peaks with migration time values of 6.89 min (µa=5.45·10−3 cm2s−1V−1), 8.53 min (µa=4.40·10−3 cm2s−1V−1) and 23.53 min (µa=1.60·10−3 cm2s−1V−1) (B), only the first one (migration time 6.89 min) corresponding to a cationic protein at pH = 9.2. About 89% of total protein loaded onto the bead was actively retained, and 30% of it was eluted with 100 mM N-acetyl-D-glucosamine (C). The eluted fraction was resolved by CE as a single peak (D) at 33.03 min (µa=4.86·10−3 cm2s−1V−1). No protein was eluted with 100 mM sucrose.
Figure 11. Separation by affinity chromatography and capillary electrophoresis of teliospore receptors for HMMG and MMMG obtained from healthy plants. (A) Elution profile of non-retained, smut ligands from agarose beads containing HMMG from healthy plants, and (B) its electropherogram. (C) Elution profile of retained, smut ligands on agarose beads containing HMMG from healthy plants and eluted with N-acetyl-D-glucosamine and (D) the electropherogram of this eluted fraction. (E) Elution profile of non-retained, smut ligands from agarose beads containing MMMG from healthy plants, and (F) its electropherogram. (G) Elution profile of retained, smut ligands on agarose beads containing MMMG from healthy plants and eluted with N-acetyl-D-glucosamine and (D) the electropherogram of this eluted fraction. Black arrow indicates the migration time value of the internal standard.
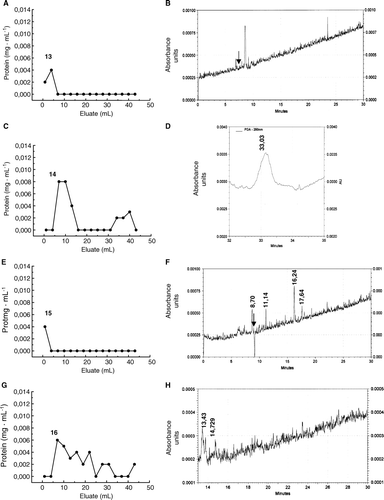
Some proteins from the teliospore cell wall were not retained by MMMG from healthy plants bound to activated agarose (E). The corresponding electropherogram revealed four different peaks with a migration time value of 8.70 min (µa=4.86·10−3 cm2s−1V−1), 11.14 min (µa=3 .80·10−3 cm2s−1V−1), 16.24 min (µa=2.60·10−3 cm2s−1V−1) and 17.64 min (µa=2.40·10−3 cm2s−1V−1) (F). On the other hand, partial elution of cell wall proteins retained on the bead was achieved by application of 100 mM N-acetyl-D-glucosamine (G). The corresponding electropherogram showed two peaks that migrated at13.43 min (µa=3.70·10−3 cm2s−1V−1) and 14.73 min (µa=3.70·10−3 cm2s−1V−1), respectively (H).
Finally, HMMG and MMMG obtained from inoculated plants were bound to activated agarose to retain cell wall proteins extracted from smut teliospores. A shows that some fungal proteins were not retained by HMMG. This protein pool was resolved by CE as two different peaks with migration time values of 8.55 min (µa=4.85·10−3 cm2s−1V−1) and 17.10 min (µa=2.43·10−3 cm2s−1V−1) (B), the first one behaving as a cationic protein. Elution of retained protein with 100 mM N-acetyl-D-glucosamine (C) produced only one peak in CE that migrated at 14.49 min (µa=2.68·10−3 cm2s−1V−1) (D). MMMG in the agarose column did not retained two different proteins (E) that separated in CE at 8.69 min (µa=4.87·10−3 cm2s−1V−1) and 14.56 min (µa=1.59·10−3 cm2s−1V−1) (F). Protein eluted with N-acetyl-D-glucosamine (G) were resolved in CE as six different peaks that migrated at 13.34 min (µa=2.88·10−3 cm2s−1V−1), 13.65 min (µa=2.82·10−3 cm2s−1V−1), 14.23 min (µa=2.70·10−3 cm2s−1V−1), 21.03 min (µa=1.83·10−3 cm2s−1V−1), 27.98 min (µa=1.37·10−3 cm2s−1V−1) and 28.93 min (µa=1.33·10−3 cm2s−1V−1) (H). Part of retained protein after elution with N-acetyl-D-glucosamine was not eluted even by using 100 mM sucrose as mobile phase.
Figure 12. Separation by affinity chromatography and capillary electrophoresis of teliospore receptors for HMMG and MMMG obtained from inoculated plants (A) Elution profile of non-retained, smut ligands from agarose beads containing HMMG from inoculated plants, and (B) its electropherogram. (C) Elution profile of retained, smut ligands on agarose beads containing HMMG from inoculated plants and eluted with N-acetyl-D-glucosamine and (D) the electropherogram of this eluted fraction. (E) Elution profile of non-retained, smut ligands from agarose beads containing MMMG from inoculated plants, and (F) its electropherogram. (G) Elution profile of retained, smut ligands on agarose beads containing MMMG from inoculated plants and eluted with N-acetyl-D-glucosamine and (D) the electropherogram of this eluted fraction. Black arrow indicates the migration time value of the internal standard.
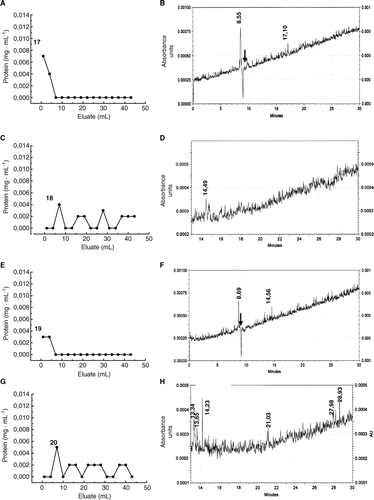
Discussion
Sugarcane glycoproteins are related to plant resistance and to smut teliospore aggregation
In accordance, our data show that inoculation of sugarcane plants with smut teliospores of U. scitaminea induces a significant increase of HMMG, polymers from which MMMG derive (Martínez et al. Citation1990). Furthermore, complete inhibition of mycelium growth by HMMG and MMMG produced by healthy plants underline the defensive nature of these glycoproteins against smut. However, HMMG and MMMG from inoculated plants partially inhibit smut proliferation (B), which suggests that the pathogen modifies the chemical structure of sugarcane glycoproteins rendering them partially inefficient against disease. The defensive role of HMMG and MMMG is likely to require the binding to teliospores. A recent report has uncovered the selective binding of some of these glycoproteins, obtained from smut-sensitive Jaronu cv., to teliospores of U. scitaminea (Fontaniella et al. Citation2002), and the data presented here confirms that HMMG and MMMG really induced homotypic adhesion in teliospores (Legaz et al. Citation1990). In a similar way, HMMG and MMMG obtained from smut-resistant Mayari cv induced recruitment of smut teliospores, which occurred through the binding of glycoproteins to specific sites on the cell wall, and completely impeded spore germination and mycelium growth when MMMG bound to the cell wall (Millanes et al. Citation2005). This was irrespective to the origin of the glycoprotein fraction, either from healthy or inoculated plants. This is in agreement with the enhanced cell adhesion observed after MMMG treatment, which partially counteracted by adding HMMG simultaneously. However, teliospore recruitment produced by HMMG and MMMG from healthy Jaronu plants is slightly more efficient than that produced by these glycoproteins isolated from inoculated plants and drastically reduced by a previous digestion of their glycidic moiety by invertase (). Since the polysaccharide moiety of both HMMG and MMMG contain several domains composed of β-D-fructofuranosyl-1,2-β-D-fructose, it is not surprising that their enzymatic hydrolysis was related to the loss of the binding ability. The integrity of this glycidic moiety is absolutely required to maintain the spatial conformation of the active binding site rather than an unequivocal demonstration of the glycidic nature of the binding site.
In some cases, the amount of protein recovered after incubation of HMMG and MMMG with teliospores is higher than that used for incubation (). A feasible interpretation includes secretion of fungal protein to the incubation medium. Alternatively, it may represent partial solubilization of proteins from surface teliospores to the media. This concurs with the appearance of extracellular matrix of pathogenic fungi, which plays a decisive role in the adhesion of spores to the surface of host plants. These extracellular matrix proteins include the 200 kDa protein of the conidia of Colletotrichum graminicola (Sugui et al. Citation1998), or those which are expressed during the juvenile phase of development of C. lindemunthianum (Perfect et al. Citation2001). Apoga et al. (Citation2001) found that Bipolaris sorokiniana conidia produced exocellular material required for the adhesion to the host surface. Treatment of conidia with tunicamicin, an inhibitor of protein glycosilation, significantly reduced adhesion.
Since the most active fraction of sugarcane glycoproteins is related to polyamine biosynthesis, we assesses arginase activity of sugarcane juice contains several enzymes related to polyamine biosynthesis, mainly ornithine decarboxylase (Legaz et al. Citation2001), using ornithine as a substrate produced by enzymatic hydrolysis of arginine. Moreover, glycosilated arginase produced by lichenized and pathogenic ascomycetes binds to the algal partner and alters the level of putrescine produced by the host cell (Molina et al. Citation1998). Inoculation of Jaronu plants with smut teliospores decreases the arginase content of HMMG, but dramatically increases it in MMMG (A). Furthermore, MMMG arginase is always retained by smut teliospores whereas only HMMG-arginase from healthy plants binds to these structures (B) which suggests changes in the quantitative and qualitative composition of glycoproteins. In fact, the lower size of the polysaccharide moiety of MMMG (Legaz et al. Citation1990) could increase binding of these glycoproteins, although studies of the topography of the ligand distribution on the teliospore cell wall and of the size and polymerization status of the sugarcane glycoproteins are required to confirm this hypothesis. On the contrary, chitinase activity related to HMMG and MMMG fractions do not significantly change after plant infection and do not bind to smut teliospores (C and 3D).
The role of actin in the germination of smut teliospores
Arabidopsis thaliana and rye seedlings developed when latrunculin B was added during germination, but these plants were stunted as a result of the inhibition of cell elongation (Baluska et al. Citation2001). In addition, latrunculin B, which polymerizes G-actin to F-actin, blocked the root hair formation in maize and Arabidopsis (Baluska et al. Citation2000). Our data suggest that inhibition of teliospore germination is related to alteration of the actin cytoskeleton induced by exposure to different glycoproteins from HMMG and MMMG. A feasible link is provided by the increase observed in sugarcane arginase. However, such link between cytoskeletal organization and increased production of sugarcane arginase and its binding to the teliospore cell wall remains elusive. Arginase activity can only be related to polyamine biosynthesis. The role of polyamines on cytoskeleton organization has been mainly studied in animal and human cells. In this regard, McCormack et al. (Citation1999) depleted migrating IEC-6 cells of polyamines with DFMO. DFMO treatment reduced the amount of F-actin, redistributed G-actin, and increased the amount of nuclear thymosin-b4 (Tb4), and prevented EGF-induced actin polymerization. In addition, DFMO caused a significant decrease of RhoA in both the cytoplasm and membrane of IEC-6 cells (Ray et al. Citation2002). Polyamines promote the assembly of actin monomers in mammalian cells. If is also the case of smut teliospores, retention of glycosylated arginase outside teliospores would explain the inhibition of cell polarization and germination by sugarcane glycoproteins from healthy plants. However, polarization also takes place in water. Thus, glycoproteins other than arginase might exhibit an inhibitory effect (). Conversely, the increase of arginase activity observed in glycoprotein fractions from inoculated plants may represent the entry of a supplement of sugarcane arginase inside teliospores after saturation of cell wall ligands. This may promote an increase in the amount of polyamines inducing the inhibition of cell polarization related to HMMG of 141 kDa from healthy plants (peak b in A and 7C), to MMMG of 20 kDa from healthy plants (peak h in C, and 9C and 9D) or MMMG from inoculated plants (D, and 10C and 10D). Thus, it can be concluded that the binding of sugarcane glycoproteins from inoculated plants to ligands located on the spore cell wall surface involves at least two different mechanisms. A glycosylated, sugarcane arginase seems to be partially retained on the cell wall, but part of this enzyme seems to be internalized by teliospores (D), which then increases the concentration of putrescine and inhibits polarization at high amounts of the diamine (>0.05 mM), as it has been found for glycoproteins of Mayari cv (Millanes et al. Citation2005). Polarization based on actin cytoskeleton is absolutely required for polarized growth in U. maydis (Fuchs et al. Citation2005) and for the hyphal-induced positioning of the Golgi apparatus in Candida albicans (Rida et al. Citation2006) although microtubules are dispensable for these processes.
Binding HMMG and MMMG ligands on the surface of smut teliospores
In this work, isolated proteins of smut teliospores are able to bind to HMMG. These proteins are resolved in two chromatographic peaks (D). This chromatographic resembles the occurrence of two major binding proteins in HMMG fraction in the range of 187 and 141 kDa, respectively. However, three peaks of ligand have been isolated from HMMG obtained from inoculated plants. This is in agreement with the appearance of three different proteins of ∼20 kDa in this HMMG fraction, as identified by CE. Ligands for MMMG show a more complicate pattern of elution from agarose although it is interesting to note that one of the main peak of teliospore ligand able to bind to MMMG from inoculated plants corresponds to a single binding protein contained in this fraction. These ligands can be eluted from their binding proteins linked to the agarose matrix by N-acetyl-D-glucosamine, which indicate that the peptide moiety of both HMMG and MMMG contains specific domains that bind to N-acetyl-D-glucosamine in the glycosidic moiety of the cell wall protein of smut teliospores in a lectin-like recognition reaction (Min et al. Citation1992). Since no desorption of ligands from the agarose beads has been achieved by using 100 mM sucrose as mobile phase ( and ), the β-1,2-fructofuranoside moiety of both HMMG and MMMG can be excluded as responsible of the binding action (Legaz et al. Citation2005) and this fact can be explained on the basis of the loss of recruitment capability of sugarcane glycoproteins after digestion with invertase (F–2J). Probably, the integrity of the polysaccharide moiety of both HMMG and MMMG was required to maintain the spatial conformation of the peptide domain active for the binding.
Relationships between HMMG and MMMG and their teliospore receptors seem to be related to a spore recruitment, which probably improves plant infection rather than a defence mechanism. Thus, this interaction is quite different to that described for plant resistance (R) and pathogen avirulence (Avr) proteins (Parker and Coleman Citation1997) since the specific binding of Avr by its matching R protein leads to activation of the plant defence responses. In addition, R factors are transmembrane (Dixon et al. Citation1996) or cytoplasmic (Whitham et al. Citation1994) proteins whereas HMMG and MMMG are located in the plant cell wall (Martínez et al. Citation1990).
Acknowledgements
This work has been supported by a grant from the Ministerio de Educación y Ciencia (Spain) BFI2003-06234. We gratefully acknowledge the excellent technical assistance of Mrs Raquel Alonso.
References
- Alexander , KC and Srinivasan , KV . 1966 . Sexuality in Ustilago scitaminea Syd . Current Sci. , 35 : 603 – 604 .
- Apoga , D , Jansson , HB and Tunlid , A . 2001 . Adhesion of conidia and germlings of the plant pathogenic fungus Bipolaris sorokiniana to solid surfaces . Mycol Res. , 105 : 1251 – 1260 .
- Baluska , F , Jasik , J , Edelman , HG , Salajova , T and Volkmann , D . 2001 . Latrunculin B-induced plant dwarfism: Plant cell elongation is F-actin dependent . Dev Biol. , 231 : 113 – 124 .
- Baluska , F , Salaj , J , Mathur , J , Braun , M , Jasper , F , Samaj , J , Chua , NH , Barlow , PW and Volkmann , D . 2000 . Root hair formation: F-actin-dependent tip growth is initiated by local assembly of profilin-supported F-actin meshworks accumulated within expansion-enriched bulges . Dev Biol. , 227 : 618 – 632 .
- Banuett , F and Herskowitz , I . 2002 . Bud morphogenesis and the actin and microtubule cytoskeletons during budding in the corn smut fungus, Ustilago maydis . Fungal Gen Biol. , 37 : 149 – 170 .
- Conway , EJ . 1962 . Microdiffusion analysis and volumetric error , 122 – 125 . London : Crosby Lockwood .
- Da Gloria , BA , Capote-Albernas , MC , Amorim , L and Bergamin-Filho , A . 1999 . “ Morphological characteristics of sugarcane clones, susceptible and resistant to smut (Ustilago scitaminea) ” . In Sugarcane pathology , Edited by: Rao , GP , Bergamin-Filho , A , Magarey , RC and Autrey , LJC . 167 – 182 . Enfield, NH : Science Publishers Inc .
- de Armas , R , Martínez , M , Rodriguez , CW , Legaz , ME , Mateos , JL , Caffaro , SV and Vicente , C . 1992 . The chemical nature of high molecular mass heterofructans from cane juice . Intern Sugar J. , 94 : 147 – 150 .
- de Armas , R , Martínez , M , Vicente , C and Legaz , ME . 1999 . Free and conjugated polyamines and phenols in raw and alkaline-clarified sugarcane juices . J Agr Food Chem. , 47 : 3086 – 3092 .
- Dixon , MS , Jones , DA , Keddie , JS , Thomas , CM , Harrison , K and Jones , JDG . 1996 . The tomato Cf-2 disease resistance locus comprises two functional genes encoding leucine-rich repeat proteins . Cell. , 84 : 451 – 459 .
- Dubois , M , Gilles , KA , Hamilton , JK , Rebers , PA and Smith , F . 1956 . Colorimetric method for determination of sugars and related substances . Anal Chem. , 28 : 350 – 356 .
- Evangelista , M , Zigmond , S and Boone , C . 2003 . Formins: Signaling effectors for assembly and polarization of actin filaments . J Cell Sci. , 116 : 2603 – 2611 .
- Fontaniella , B , Márquez , A , Rodríguez , CW , Piñon , D , Solas , MT , Vicente , C and Legaz , ME . 2002 . A role for sugarcane glycoproteins in the resistance of sugarcane to Ustilago scitaminea . Plant Physiol Biochem. , 40 : 881 – 889 .
- Fuchs , U , Manns , I and Steinber , G . 2005 . Mucrotubules are dispensable for long-distance Hyphal growth in the corn smut fungus Ustilago maydis . Mol Biol Cell. , 16 : 2746 – 2758 .
- Heath , IB . 1987 . Preservation of labile cortical array of actin filaments in growing hiphal tips of the fungus Saprolegnia ferax . Eur J Cell Biol. , 44 : 10 – 16 .
- Hou , WC , Chen , C and Lin , YH . 1998 . Chitinase activity of sweet potato (Ipomoea batatas (L.) Lam var. Taimong 57) . Bot. Bull Acad Sin. , 39 : 93 – 97 .
- Imoto , T and Yagishita , K . 1971 . A simple activity measurement lysozyme . Agric Biol Chem. , 35 : 1154 – 1156 .
- Lebeda , A , Luhová , L , Sedlářová , D and Jančová , D . 2001 . The role of enzymes in plant-fungal pathogen interactions . J Plant Dis Protect. , 108 : 89 – 111 .
- Lee-Lovick , G . 1978 . Smut of Sugarcane-Ustilago scitaminea . Rev Plant Pathol. , 57 : 181 – 188 .
- Legaz , ME , de Armas , R , Millanes , AM , Rodriguez , CW and Vicente , C. 2005 . Heterofructans and heterofructancontaining glycoproteins from sugarcane: structure and function . Recent Res Devel Biochem. , 6 : 31 – 51 .
- Legaz , ME , Fontaniella , B , de Armas , R and Vicente , C. 2001 . Determination by high performance liquid chromatography of ornithine and lysine decarboxylases in sugarcane juices . Chromatographia. , 53 : S260 – 265 .
- Legaz , ME , Fontaniella , B , Millanes , AM and Vicente , C. 2004 . Secreted arginases from far-phylogenetically farrelated lichens species act as cross-recognition factors for two different algal cells . Eur J Cell Biol. , 83 : 435 – 446 .
- Legaz , ME , Martín , L , Pedrosa , MM , Vicente , C , de Armas , R , Martínez , M , Medina , I and Rodríguez , CW. 1990 . Purification and partial characterization of a fructanase which hydrolyzes natural polysaccharides from sugar cane juice . Plant Physiol. , 92 : 679 – 683 .
- Legaz , ME and Pedrosa , MM. 1993 . Separation of acidic proteins by capillary zone electrophoresis and sizeexclusion high performance liquid chromatography: a comparison . J Chromatogr A. , 655 : 21 – 29 .
- Legaz , ME , Pedrosa , MM , Armas , R , MartLegaznez , M and Vicente , C. 1995 . Soluble glycoproteins from sugar cane juice analyzed by high performance liquid chromatography and fluorescence emission . J Chromatogr. , 697 : 329 – 335 .
- Legaz , ME , Pedrosa , MM , de Armas , R , Rodríguez , CW , de los Ríos , V and Vicente , C. 1998 . Separation of soluble glycoproteins from sugarcane juice by capillary electrophoresis . Anal Chim Acta. , 372 : 201 – 208 .
- Legaz , ME and Vicente , C. 1982 . Two forms of arginase in Evernia prunastri thallus . Biochem Biophys Res Commun. , 104 : 1441 – 1446 .
- Lilly , VG and Barnett , HL . 1951 . Physiology of the fungi , 97 – 99 . New York : McGraw-Hill .
- Lloyd , HL and Naidoo , G . 1983 . Chemical array potentially suitable for determination of smut resistance of sugarcane cultivars . Plant Dis. , 67 : 1103 – 1105 .
- Magdalena , J , Millard , TH and Machesky , LM . 2003 . Microtubule involvement in NIH 3T3 Golgi and MTOC polarity establishment . J Cell Sci. , 116 : 743 – 756 .
- Martínez , M , Legaz , ME , Paneque , M , Armas , R , Pedrosa , MM , Medina , I , Rodríguez , CW and Vicente , C . 1990 . The origin of soluble fructans in sugarcane juice . Intern Sugar J. , 92 : 155 – 159 .
- Martínez , M , Medina , I , Naranjo , S , Rodriguez , CW , de Armas , R , Piñon , D , Vicente , C and Legaz , ME . 2000 . Changes of some chemical parameters, involved in sucrose recovery from sugarcane juices, related to the susceptibility or resistance of sugarcane plants to smut (Ustilago scitaminea) . Intern Sugar J. , 102 : 445 – 448 .
- Matsumoto , I . 1985 . “ Activation procedures ” . In Affinity chromatography: A practical approach , Edited by: Dean , PDG , Johnson , WS and Middle , FA . 31 – 34 . Oxford : IRL Press .
- McCormack , SA , Ray , RM , Blanner , PM and Johnson , LR . 1999 . Polyamine depletion alters the relationship of F-actin, and thymosin b 4 in migrating IEC-6 cells . Am J Physiol Cell Physiol. , 45 : C459 – C468 .
- Millanes , AM , Fontaniella , B , Legaz , ME and Vicente , C . 2005 . Glycoproteins from sugarcane plants regulate cell polarity of Ustilago scitaminea teliospores . J Plant Physiol. , 162 : 253 – 265 .
- Min , W , Dunn , AJ and Jones , DH . 1992 . Non-glycosylated recombinant pro-concanavalin A is active without polypeptide cleavage . EMBO J. , 11 : 1303 – 1307 .
- Molina , MC , Stocker-Wörgötter , E , Turk , R , Bajon , C and Vicente , C . 1998 . Secreted, glycosylated arginase from Xanthoria parietina thallus induces loss of cytoplasmic material from Xanthoria photobionts . Cell Adhes Commun. , 6 : 481 – 490 .
- Parker , JE and Coleman , MJ . 1997 . Molecular intimacy between proteins specifying plant-pathogen recognition . Trends Biochem Sci. , 22 : 291 – 296 .
- Perfect , SE , Green , JR and O'Connell , RJ . 2001 . Surface characteristics of necrotrophc secondary hyphae produced by the bean anthracnose fungus, Colletotrichum lindemuthianum . Eur J Plant Pathol. , 107 : 813 – 819 .
- Piñón , D , de Armas , R , Vicente , C and Legaz , ME . 1999 . Role of polyamines in the infection of sugarcane buds by Ustilago scitaminea spores . Plant Physiol Biochem. , 37 : 57 – 64 .
- Pollard , TD and Borisy , GG . 2003 . Cellular motility driven by assembly and disassembly of actin filaments . Cell. , 112 : 453 – 465 .
- Raggi , V . 2000 . Hydroxyproline-rich glycoprotein accumulation in tobacco leaves protected against Erysiphe cichoracearum by potato virus Y infection . Plant Pathol. , 49 : 179 – 186 .
- Ramos , RLB , Tovar , FJ , Junqueira , RM , Lino , FB and Sacheto-Martins , G . 2001 . Sugarcane expressed sequences tags (ESTs) encoding enzymes involved in lignin biosynthesis pathways . Genet Mol Biol. , 24 : 235 – 241 .
- Ray , RM , Patel , A , Viar , MJ , McCormack , SA , Zheng , Y , Tigyi , G and Johnson , LR . 2002 . RhoA inactivation inhibits cell migration but does not mediate the effects of polyamine depletion . Gastroenterology. , 123 : 196 – 205 .
- Rida , PCG , Nishikawa , A , Wong , GY and Dean , N . 2006 . Yeast-to-hyphal transition triggers forming-dependent Golgi localization to the growing tip in Candida albicans . Mol Biol Cell. , 17 : 4364 – 4378 .
- Schenk , S . 1999 . “ Molecular aspects of the sugarcane smut disease pathogen, Ustilago scitaminea ” . In Sugacane pathology , Edited by: Rao , GP , Bergamin-Filho , A , Magarey , RC and Autrey , LJC . 131 – 139 . Enfield, NH : Science Publishers Inc .
- Soares-Costa , A , Beltramini , LM , Thieman , OH and Henrique-Silva , F . 2002 . A sugarcane cystatin: Recombinant expression, purification and antifungal activity . Biochem Biophys Res Commun. , 296 : 1194 – 1199 .
- Sugui , JA , Leite , B and Nicholson , RL . 1998 . Partial characterization of the extracellular matrix released onto hydrophobic surfaces by conidia and conidial germlings of Colletotrichum graminicola . Physiol Mol Plant Pathol. , 52 : 411 – 425 .
- Waller , JM . 1970 . Sugarcane smut (Ustilago scitaminea) in Kenya. II. Infection and resistance . Trans British Mycol Soc. , 54 : 405 – 414 .
- Warburg , O and Christian , W . 1941 . Isolierung und Kristallisation des Gärungsferments Enolase . Biochem Z. , 310 : 384 – 421 .
- Wedlich-Soldner , R , Altschuler , S , Wu , L and Li , R . 2003 . Spontaneous cell polarization through actomyosin-based delivery of the Cdc42 GTPase . Science. , 299 : 1231 – 1235 .
- Whitham , S , Dinesh-Kuma , SP , Choi , D , Hehl , R , Corr , C and Baker , B . 1994 . The product of the tobacco mosaic virus resistance gene N: Similarity to toll and the interleukin-1 receptor . Cell. , 78 : 1101 – 1115 .