Abstract
In the present work, the response to NaCl applied at the vegetative stage to Medicago truncatula and Lotus japonicus has been evaluated in order to ascertain whether the effect of salt stress on nitrogen fixation is due to a limitation on nodular carbon metabolism. Results show maximum sucrose synthase (SS) and alkaline invertase (AI) activities were obtained at the vegetative stage, when maximum nitrogenase activity was detected in both species. SS activity decreased with the salt treatment, providing evidence of the regulatory role of this enzyme for the carbon supply to the bacteroids. Phosphoenolpyruvate carboxylase (PEPC) and malate dehydrogenase (MDH) activities could account for higher nitrogen fixation efficiency detected in L. japonicus nodules and isocitrate dehydrogenase (ICDH) activity compensated for the carbon limitations that occur under salt stress. These results support that nitrogenase inhibition in nodules experiencing salt stress is doubt to a carbon flux shortage, as result of carbon metabolism enzymes activities down-regulation.
Abbreviations
AI | = |
alkaline invertase |
ANA | = |
apparent nitrogenase activity |
DAT | = |
days after treatment |
HK | = |
hexokinase |
ICDH | = |
isocitrate dehydrogenase |
Lb | = |
leghemoglobin |
MDH | = |
malate dehydrogenase |
PDW | = |
plant dry weight |
PEPC | = |
phosphoenolpyruvate carboxylase |
RSR | = |
root-shoot ratio |
SS | = |
sucrose synthase |
TNA | = |
total nitrogenase activity |
TSS | = |
total soluble sugars. |
Introduction
Salinity is considered a significant factor affecting crop production and agricultural sustainability in arid and semi-arid regions of the world, reducing the value and productivity of the affected land (Munns Citation2002). Symbiotic nitrogen fixation in legume nodules is extremely sensitive to environmental stresses such as salinity, which seriously limit legume yields. Salt stress affects several physiological processes in root nodules, such as the range of carbon substrate available to the bacteroids (Delgado et al. Citation1993; Soussi et al. Citation1998) and the activity of enzymes related to carbon metabolism (Khadri et al. Citation2001; Serraj Citation2002). Nitrogen-fixing plants are more sensitive to salinity than N-fertilized plants (González et al. Citation2001).
Nodule nitrogen fixation depends on the photosynthate in the form of sucrose delivered from the phloem (Gordon et al. Citation1998). Once in the nodule, sucrose can be cleaved by either sucrose synthase (SS; EC 2.4.1.13) or alkaline invertase (AI; EC 3.2.1.26). Sucrose synthase is reported to be essential for nitrogen fixation in legume nodules (Gordon et al. Citation1999), however, less is known about alkaline invertase. The relative activity of both enzymes varies with developmental stage (Ocaña et al. Citation1998). Hexose phosphates derived from sucrose cleavage are metabolized through the glycolytic pathway to render phosphoenolpyruvate, which is converted to malate via the combined action of phosphoenolpyruvate carboxylase (PEPC; EC 4.1.1.31) and malate dehydrogenase (MDH; EC 1.1.1.82) (Salminen and Streeter Citation1992). Phosphoenolpyruvate carboxylase has been demonstrated to be crucial for the carbon metabolism in Lotus japonicus nodules (Nomura et al. Citation2006). Multiple evidences supports that C4-dicarboxylates are the main products of sucrose breakdown supplied to the bacteroids to fuel nitrogen fixation (Lodwig and Poole Citation2003).
It has been widely reported that biological nitrogen fixation in legume nodules declines under salinity and other environmental stresses (Zahran Citation1999). The decrease in nitrogen fixation has been ascribed to a direct effect on nitrogenase (Serraj and Drevon Citation1998 ) or an indirect effect through decreases in leghaemoglobin content, respiration rate and malate concentration in nodules (Delgado et al. Citation1994). SS has been shown to be the first nodule enzyme activity that declines under salt stress, and a strong correlation between nodule SS activity and nitrogen fixation under a variety of stresses has been reported (Arrese-Igor et al. Citation1999).
NADP-isocitrate dehydrogenase activity (ICDH; EC 1.1.1.42) is considered to be one of the main sources of NADPH and substrates for energy-yielding metabolism of bacteroids, in addition to carbon skeletons for ammonia assimilation (Chopra et al. Citation2002). This implies that ICDH might be of crucial relevance for the C/N balance within nodules under stress conditions and NADPH requirements by the antioxidant ascorbate/glutathione pathway, activated in nodules under salt stress conditions (Matamoros et al. Citation2003).
Organic solutes such as sugars and amino acids have been proven to be helpful in osmorregulation and enzyme activity in plant species (Mansour Citation2000; Khadri et al. Citation2007). It has been assumed the accumulation of organic solutes in response to salt stress is involved in protection mechanisms such as the restoration of the cell volume and turgor, the reduction of the cell damage induced by free radicals, the protection and stabilization of enzymes and membrane structures (Bartels and Sunkar Citation2005). Salinity is known to boost the nodular carbohydrate content and sucrose is the predominant carbohydrate in legume root nodules (Fougère et al. Citation1991; Gordon et al. Citation1997). It has been argued that the accumulation of sucrose under salinity, associated with inhibition of the sucrose hydrolytic enzyme sucrose synthase (Arrese-Igor et al. Citation1999), induces a deficiency of carbon for bacteroids with the subsequent inhibition of nitrogenase activity (Hunt and Layzell Citation1993). A strong correlation between sugar accumulation and osmotic stress tolerance has been widely reported, including transgenic experiments (Gilmour et al. Citation2000; Streeter et al. Citation2001; Taji et al. 2002).
In the present work, the response to NaCl applied at the vegetative period to Medicago truncatula and L. japonicus under symbiotic conditions, has been evaluated in order to establish the differences in nitrogen fixation and carbon metabolism between both legumes, forming indeterminate and determined nodules respectively, and adopted internationally as models for genomic and proteomic research. The aim of this work is to ascertain whether the negative effect of salt stress on nitrogen fixation is due to a limitation on the nodular carbon metabolism. In this sense, the interaction between nitrogen fixation and nodular carbon metabolism has been investigated, as well as the relationships of these activities with changes in the level of sugars and amino acids in nodules and leaves of both legumes subjected to salt stress.
Materials and methods
Plant material and experimental treatments
Lotus japonicus (cv. Gifu) and Medicago truncatula (var. Jemalong) seeds were scarified by immersion in concentrated H2SO4 for 5 min, washed with sterile water, surface sterilized by immersion in 5% NaClO plus Tween 20 for 20 min and germinated onto 0.8% water-agar plates at 28°C in the darkness. After four days, Lotus and Medicago seedlings were transferred to sterile vermiculite and watered with Hornum nutrient solution (Handberg and Stougaard Citation1992) and a modified Rigaud and Puppo (Citation1975), respectively. Two days later, L. japonicus seedlings were inoculated with 1 ml of a stationary culture of Mesorhizobium loti R7A strain and M. truncatula seedlings with Sinorhizobium meliloti GR4 strain (c. 109 cell ml−1) grown in a TY medium (tryptone-yeast extract, Beringer Citation1974). Plants, in individual pots of about 300 ml, were grown in a controlled environmental chamber with a 16/8 h light-dark cycle, 23/18°C day night temperature, relative humidity 55/65% and photosynthetic photon flux density (400–700 nm) of 450 µmol m−2 s−1 supplied by combined fluorescent and incandescent lamps. Once the symbiosis was well established and plants were at vegetative stage (five weeks M. truncatula and seven weeks L. japonicus after sowing), they were subjected to salt treatment by adding NaCl to the growth medium (50 mM). Control plants were maintained in a NaCl-free nutrient solution. Plants were harvested at 0 (vegetative stage), 14 (flowering), 21 (early fructification) and 28 (advance fructification) days after salt addition. Nodules and leaves were frozen at -80°C for further analyses. Samples of leaves, stems, roots and nodules were dried at 70°C for 24 h and dry weight estimated.
Nitrogen fixation
Nitrogenase activity (E.C. 1.7.9.92) was measured as the representative H2 evolution in an open-flow system (Witty and Minchin Citation1998) using an electrochemical H2 sensor (Qubit System Inc., Canada). For nitrogenase measurements, pots maintained in a controlled environmental chamber as described above, were sealed and H2 production was recorded. Apparent nitrogenase activity (ANA, rate of H2 production in air) was determined under N2:O2 (80%:20%) with a total flow of 0.4 l min−1. After reaching steady-state conditions total nitrogenase activity (TNA) was determined under Ar:O2 (79%:21%). Standards of high purity H2 were used to calibrate the detector.
Leghemoglobin determination
Leghemoglobin (Lb) content was determined by the fluorometric procedure of La Rue and Child (Citation1978) with some modifications. Crude nodule extracts were obtained by grinding 0.5 g nodules in 6 ml 50 mM phosphate buffer (pH 7.4) containing 0.02% K3Fe(CN)6 and 0.1% NaHCO3. The extract was centrifuged at 24,000 g for 20 min and 50 µl of supernatant was mixed with a saturated solution of oxalic acid and heated at 120°C for 30 min. Fluorescence of the supernatant was measured in a Shimadzu RF-540 fluorimeter (excitation wavelength 405 nm, emission wavelength 600 nm) using calibration curves of bovine hemoglobin.
Preparation of extracts and enzyme assays
Extracts were prepared by homogenizing 0.2 g of nodules in a mortar with 33% (w/w) polyviniyl-polypyrrolidone and 1.5 ml of 50 mM phosphate K buffer (pH 8) containing 1 mM EDTA and 20% (v/v) ethylenglycol for SS and hexokinase (HK) activities; 1.5 ml 100 mM maleic KOH buffer (pH 6.8) containing 100 mM sucrose, 2% (v/v) β- mercaptoethanol and 20% (v/v) ethylenglycol for (PEPC, ICDH and MDH) and 2 ml of 100 mM MES buffer (pH 6.3) containing 2 mM EDTA and 2 mM PMSF for AI. Extracts were centrifuged at 30000 g for 20 min and supernatants were used to determine enzyme activities. All operations were carried out at 4°C, and enzyme activities were monitored between 2 and 4 h.
Sucrose synthase activity (E.C. 2.4.1.13) was determined according to Morell and Copeland (Citation1985). The production of UDP-glucose was coupled to the reduction of NAD+ in the presence of excess UDP-glucose dehydrogenase. The activity was spectrophotometrically measured by following the NAD+ reduction at 340 nm.
Alkaline invertase activity (E.C. 3.2.1.26) was assayed by incubating for 30 min at 30°C enzyme aliquots in 20 mM phosphate K buffer (pH 7.5) containing 100 mM sucrose (modified from Morell and Copeland Citation1984).
Hexokinase activity (E.C. 2.7.1.1) was assayed according to Levi and Preiss (Citation1978) and isocitrate dehydrogenase (E.C. 1.1.1.42) according to Chen et al. (Citation1988), both activities was followed by reduction of NADP+ at 340 nm.
Phosphoenolpyruvate carboxilase (E.C. 4.1.1.31) and malate dehydrogenase (E.C. 1.1.1.37) activity assays were optimiced from the Soussi et al. (Citation1998) method. Activity was followed by oxidation of NADH at 340 nm.
Organic solute analysis
Total soluble sugars and amino acids were determined by the colorimetric methods of Irigoyen et al. (Citation1992) and Yemm and Cocking (Citation1955). The quantification of TSS was performed on the ethanolic extracts incubated with anthrone reagent at 100°C for 10 min and the absorbance measured at 625 nm. Amino acids were determined using ninhydrine reagent. Standard curves, prepared with glucose and L-asparagine, respectively, were used to estimate concentrations.
Statistical analyses
The experimental layout was a randomized complete block design. The growth and nitrogen fixation values were means of five replicates per treatment. Three replicates were performed for the other parameter studied. All results were subjected to two-way analysis of variance with a least significant difference (LSD) test between means using a Statgraphics 5.0 (Statistical Graphics Corp., Rockville, MD, USA). The standard errors (SE) were also calculated.
Results
Plant growth and nitrogen fixation
The effects of salt treatment on plant growth are shown in . Plant growth was monitored throughout 28 days from vegetative to fructification stages. At the intermediate harvest times (14 and 21 DAT), NaCl treatment accelerated plant development, inducing an increase of plant biomass around 30% at flowering stage (14 DAT) in both species, and 12% and 30% in M. truncatula and L. japonicus at 21 DAT, respectively, relative to control plants. At the end of the experimental time (28 DAT) salt treatment reduced plant growth (PDW) by 20% in M. truncatula and 10% in L. japonicus (not statistically significant). The root to shoot ratio experimented different responses with salinity in each specie, increasing in L. japonicus at all harvest times and decreasing in M. truncatula except at 21 DAT, when no significant difference is found.
Table 1 Effect of sodium chloride on growth and nitrogen fixation parameters: plant dry weight (PDW, mg−1 plant); root to shoot ratio (RSR); apparent nitrogenase activity (ANA, µmol H2 g−1 h−1); total nitrogenase activity (TNA, µmol H2 g−1 h−1) and leghemoglobin content (Lb, mg−1 NDW) in M. truncatula and L. japonicus inoculated with S. meliloti GR4 and M. loti R7A strains, respectively.
Apparent nitrogenase activity was inhibited in M. truncatula plants from the first harvest (14 DAT) around 15% by the salt treatment, while in L. japonicus this parameter only showed a statistically significant decrease at 21 DAT (53%), and a slight increase (no significant) at 14 DAT (14%). Similar behaviour was observed for total nitrogenase activity, although only a significant decrease is observed in M. truncatula at 14 DAT (25%) and, in L. japonicus, at 21 (52%) and 28 DAT (30%). So much in M. truncatula as in L. japonicus, nitrogenase activity decreased in the transition from the vegetative to flowering stage (14 DAT). The Lb levels in nodules of L. japonicus were unaffected by the salt treatment, although its concentration decreased around 30% throughout the experimental time. On the contrary, Lb levels decreased 40% and 60% at 21 and 28 DAT in M. truncatula nodules by the salinity.
Nodule enzymes
Nodule enzyme activities involved in carbon metabolism in general decreased with the salt treatment (). Sucrose synthase activity was inhibited by 30%, 20% and 50% at 14, 21 and 28 DAT, respectively in M. truncatula nodules by the salt treatment; whereas in L. japonicus, the inhibition percentages oscillate between 25% at 14 DAT and 60% at 21 and 28 DAT. Alkaline invertase activity, involved in sucrose cleavage as well as sucrose synthase, showed an activity decrease in M. truncatula proportional to the salt treatment length, with decreases of 10%, 15% and 25% at 14, 21 and 28 DAT, respectively. In L. japonicus, the activity decrease was independent of the treatment length, showing around 15% inhibitions at all harvest times. In control plants of both species, so much SS as AI activity diminished during the experimental time, being the decrease around 20% in SS and 55% in AI. Regarding HK activity, it showed different trend under salt stress in each legume; in M. truncatula augmented at 21 and 28 DAT 22% and 41%, respectively, and in L. japonicus decreased around 35% at each harvest time. On the other hand, PEPC and MDH activities behaved similarly in M. truncatula nodules, where the salt treatment effect was more evident at 28 DAT with activity decreases of 55% and 30%, respectively. In L. japonicus nodules, the same enzyme activities showed in general a lower inhibition by the salt effect: PEPC only decreased significantly 14 DAT (20%) and MDH declined around 15% at each harvest time. Conversely, ICDH activity was higher in nodules of salt stressed plants, showing 5%, 8% and 15% increase in M. truncatula at 14, 21 and 28 DAT, and 6% and 15% increase in L. japonicus at 21 and 28 DAT respectively. So much PEPC as MDH activities in control nodules of L. japonicus declined at fructification stages (21 and 28 DAT), showing the maximum activity at flowering stage (14 DAT).
Figure 1. Effect of NaCl treatment on nodule enzyme activities: sucrose synthase (SS), alkaline invertase (AI), hexokinase (HK), phosphoenolpyruvate carboxylase (PEPC), malate dehydrogenase (MDH) and isocitrate dehydrogenase (ICDH) of M. truncatula and L. japonicus. Vertical bars represent±SE (n=4) of four replicates.
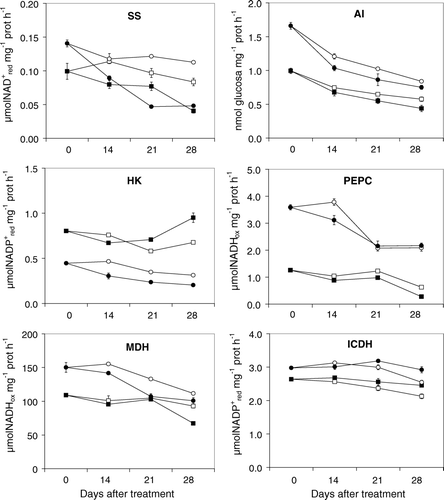
Organic solutes in leaves and nodules
Total soluble sugar content in leaves and nodules () of both legumes augmented over experimental time in salt stressed plants, except in M. truncatula nodules, where the total soluble sugars content decreased 18% at 21 and 45% at 28 DAT relative to the control plants. Salinity stress triggered an increase of sugars concentration in L. japonicus nodules (3-fold) and leaves (20% and 40%) relative to control plants at 14 and 21 DAT, in M. truncatula leaves, the increase was inversely proportional to the length of the salt treatment, showing 80%, 70% and 50% higher sugars concentrations at 14, 21 and 28 DAT, respectively. The concentration of soluble sugars was 2- to 4-fold higher in nodules than in leaves of M. truncatula; conversely, the concentration in L. japonicus was higher in leaves than in nodules, except at 28 DAT when it was similar.
Table 2 Effect of sodium chloride on total soluble sugars (TSS, mg glucose g−1 DW) and total amino acids (mg g−1 DW) in M. truncatula and L. japonicus inoculated with S. meliloti GR4 and M. loti R7A strains, respectively.
Salt drastically induced the free amino acid accumulation in nodules of both legumes at all experimental times; this accumulation was higher in M. truncatula nodules, at 21 and 28 DAT (32% and 60%), as compared with L. japonicus (6% and 27%), whereas no clear trend in M. truncatula leaves and a significant decrease in L. japonicus leaves is observed by the salt effect. The total amino acid content did not experience changes in nodules of M. truncatula over the experimental time; meanwhile, a slight increase in leaves of L. japonicus and M. truncatula was detected.
Discussion
The adaptation capacity to salinity differ considerably among species and, as a result, tolerance to salt of the host plant cultured under symbiotic conditions were different in M. truncatula and L. japonicus. In this regard, the decrease in growth by salinity proved significant only in M. truncatula plants at the last experimental stage (28 DAT). Depressive effects of NaCl on growth have been reported in several important legumes (Delgado et al. Citation1994; Tejera et al. Citation2006). However, plant dry weight showed that 50 mM NaCl promoted growth of both legumes relative to the control plants at 14 and 21 DAT. Similar results have been reported in several L. japonicus genotypes (Quero et al. Citation2007). Shoot growth was more extensively affected than root growth in L. japonicus plants, consequently increases of root to shoot ratio with NaCl in L. japonicus plants are observed. Similar results have been detected in other legumes such as Cicer arietinum (Soussi et al. Citation1998) and Phaseolus vulgaris (Khadri et al. Citation2006), where the authors considered this behaviour profitable since it could improve plant water status by increasing the root requirements to explore a higher soil volume.
The specific nitrogenase activity (µmol H2 g−1) decreased from the vegetative (0 DAT) to the fructification stage (28 DAT) in nodules of both legumes; however, the nodular mass (data not shown) augmented in this period, by which the total nitrogenase activity (µmol H2 plant−1) did not decrease in the same extend, supplying fixed nitrogen for the plant growth. Salinity significantly reduced the apparent nitrogenase activity in M. truncatula at all experimental times; meanwhile, in L. japonicus no significant differences were found at 14 and 28 DAT. The inhibition of the nitrogen fixation process as result of NaCl stress has been widely reported (Soussi et al. Citation1999; Khadri et al. Citation2007). Leghemoglobin content showed higher values in L. japonicus than in M. truncatula nodules in the same way that nitrogenase activity, which would be related to the observation that in indeterminate nodules (M. truncatula) only part of the tissue contains Lb and fixes nitrogen (Sprent Citation1980; Hirsch Citation1992).
The accumulation of organic solutes under saline conditions has been well documented (Mansour Citation2000; Bartels and Sunkar Citation2005). The clear accumulation of carbohydrates in leaves supports its role as osmoprotectants in both species. However, total soluble sugars only increased in nodules of L. japonicus under salinity, suggesting the decline in nitrogenase activity observed in this specie was not caused directly by a lack of photosynthates (Hunt and Layzell Citation1993). This sugar accumulation may be due to the substantial decline in sucrose synthase activity, which controls the flux of carbon and energy for the bacteroids (Arrese-Igor et al. Citation1999), leading to the decrease in nitrogen fixation. A strong correlation between sugar accumulation and osmotic stress tolerance has been widely reported (Gilmour et al. Citation2000; Streeter et al. Citation2001; Taji et al. 2002), which support a higher tolerance of L. japonicus nodules to salt stress. M. truncatula nodules did not show nodule carbohydrate accumulation; however, amino acids accumulated in these nodules by NaCl, which could be explained as a consequence of damage produced by saline stress, in accordance with results of Gordon et al. (Citation1997), Soussi et al. (Citation1998) and Khadri et al. (Citation2007) in nodules of soybean, chickpea and common bean, respectively.
The simultaneous action of alkaline invertase and sucrose synthase in nodules of both legumes ensures hexose supply for meeting the energy and reducing power demands of nodules during the stage of active nitrogen fixation, and these enzymes may regulate carbon metabolism and nitrogen fixation. In this regard, maximum SS and AI activities were obtained at the vegetative stage, when maximum nitrogenase activity was detected in both species. In addition, L. japonicus presented higher SS and AI activities than M. truncatula, in accordance with its higher nitrogenase activity. It has been previously hypothesized that there is a close link between ANA and the capacity of the nodule to metabolize sucrose (Gordon et al. Citation1997, Citation1999). In particular, SS activity was more sensitive to salt stress as compared with AI, in agreement with previous works that have established that nodule sucrose synthase are particularly sensitive to stress (González et al. Citation1995; Gordon et al. Citation1997).
The conversion of sucrose to malate in legume nodules occurs via PEPC and MDH enzyme activities, responsible for the carbon supply to the bacteroids (Salminen and Streeter Citation1992). It has been suggested that PEPC activity rates are closely related to nitrogen fixation rates and decreasing PEPC expression resulted in impaired nitrogen fixation in L. japonicus nodules (Nomura et al. Citation2006). However, the rapid response of SS activity to the salt treatment in both species observed in this study, provides further evidence of the key regulatory role that SS activity plays in the supply of glycolytic carbon flux to the bacteroids. PEPC and MDH activities showed higher values and were more tolerant to salinity in L. japonicus versus M. truncatula nodules, which could account for higher nitrogen fixation efficiency in L. japonicus nodules. Conversely to the other enzyme activities assayed, ICDH showed a slight increase in nodules under salt stress. It has been reported that this enzyme activity provides carbon skeletons for ammonia assimilation (Chopra et al. Citation2002) through the GS/GOGAT pathway by the synthesis of α-ketoglutarate (Scheible et al. Citation2000). Thus, the increase of ICDH activity by the salinity might compensate for the carbon limitation that occurs in nodules under salt stress, as it has been previously suggested under water stress (Gálvez et al. Citation2005). Moreover, ICDH could play a relevant role ensuring an adequate supply of NADPH required by the antioxidant ascorbate/glutathione pathway, which has been shown to occur within nodules under salt stress conditions (Matamoros et al. Citation2003).
This study provides evidences supporting that nitrogenase activity inhibition in indeterminate and determined nodules experiencing salt stress, is doubt to a carbon flux shortage, as result of carbon metabolism enzymes activities down-regulation. L. japonicus plants showed a higher accumulation of TSS in nodules, which support carbohydrate accumulation as a strategy to tolerate salinity in this specie. Conversely, M. truncatula accumulated amino acids in nodules, which could reflect the damage caused by NaCl.
References
- Arrese-Igor , C , González , EM , Gordon , AJ , Minchin , FR , Gálvez , L , Royuela , M , Cabrerizo , PM and Aparicio-Tejo , PM . 1999 . Sucrose synthase and nodule nitrogen fixation under drought and other environmental stresses . Symbiosis. , 27 : 189 – 212 .
- Bartels , D and Sunkar , R . 2005 . Drought and salt tolerance in plants . Crit Rev Plant Sci. , 24 : 23 – 58 .
- Beringer , JE . 1974 . R Factor transfer in Rhizobium leguminosarum . J Gen Microbiol. , 84 : 188 – 198 .
- Chen , RD , Marechal , JV , Jacquot , JP and Gadal , P . 1988 . Purification and comparative properties of the citosolic isocitrate dehydrogenases (NADP) from pea (Pisum sativum L.) root and green leaves . Eur J Biochem. , 175 : 565 – 572 .
- Chopra , J , Kaur , N and Gupta , AK . 2002 . A comparative developmental pattern of enzymes of carbon metabolism and pentose phosphate pathway in mugbean and lentil nodules . Acta Physiol Plant. , 24 : 67 – 72 .
- Delgado , MJ , Garrido , JM , Ligero , F and Lluch , C . 1993 . Nitrogen fixation and carbon metabolism by nodules and bacteroids of pea plants under sodium chloride . Physiol Plant. , 89 : 824 – 829 .
- Delgado , MJ , Ligero , F and Lluch , C . 1994 . Effects of salt stress on growth and nitrogen fixation by pea, faba-bean, common bean and soybean plants . Soil Biol Biochem. , 26 ( 3 ) : 371 – 376 .
- Fougère , F , Rudulier , D and Streeter , JG . 1991 . Effects of salt stress on amino acid, organic acid, and carbohydrate composition of roots, bacteroids, and cytosol of alfalfa (Medicago sativa L.) . Plant Physiol. , 96 : 1228 – 1236 .
- Gálvez , L , González , EM and Arrese-Igor , C . 2005 . Evidence for carbon flux shortage and strong carbon/nitrogen interactions in pea nodules at early stages of water stress . J Exp Bot. , 56 : 2551 – 2561 .
- Gilmour , SJ , Sebolt , AM , Salazar , MP , Everard , JD and Thomashow , MF . 2000 . Overexpression of the Arabidopsis CBF3 transcriptional activator mimics multiple biochemical changes associated with cold acclimation . Plant Physiol. , 124 : 1854 – 1865 .
- González , EM , Gordon , AJ , James , CL and Arrese-Igor , C . 1995 . The role of sucrose synthase in the response of soybean nodules to drought . J Exp Bot. , 46 : 1515 – 1523 .
- González , EM , Gálvez , L , Royuela , M , Aparicio-Tejo , PM and Arrese-Igor , C . 2001 . Insights into the regulation of nitrogen fixation in pea nodules: Lessons from drought, abcisic acid and increased photoassimilate availability . Agronomie. , 21 : 607 – 613 .
- Gordon , AJ , Minchin , FR , Sk⊘t , L and James , CL . 1997 . Stress-induced declines in soybean N2 fixation are related to nodule sucrose synthase activity . Plant Physiol. , 114 : 937 – 946 .
- Gordon AJ , Skot L , Webb KJ , Minchin FR , James CL. 1998 . Biological nitrogen fixation for the 21st century. Chapter 4, Down-regulation of nodule sucrose synthase by mutation and antisense . The Netherlands : Eelmerch . pp 67 – 83 .
- Gordon , AJ , Minchin , FR , James , LC and Komina , O . 1999 . Sucrose synthase in legume nodules is essential for nitrogen fixation . Plant Physiol. , 120 : 867 – 878 .
- Handberg , K and Stougaard , J . 1992 . Lotus japonicus, an autogamous, diploid legume species for classical and molecular genetics . Plant J. , 2 : 487 – 496 .
- Hirsch , A . 1992 . Developmental biology of legume nodulation . New Phytol. , 122 : 211 – 237 .
- Hunt , S and Layzell , DB . 1993 . Gas exchange of legume nodule and the regulation of nitrogenasa activity . Ann Rev Plant Physiol Plant Mol Biol. , 44 : 483 – 511 .
- Irigoyen , JJ , Emerich , DW and Sánchez-Díaz , M . 1992 . Water stress induced changes in concentrations of proline and total soluble sugar in nodulated alfalfa (Medicago sativa) plants . Physiol Plant. , 84 : 55 – 60 .
- Khadri , M , Pliego , L , Soussi , M and Ocaña , A . 2001 . Ammonium assimilation and ureide metabolism in common bean (Phaseolus vulgaris) nodules under salt stress . Agronomie. , 21 : 635 – 643 .
- Khadri , M , Tejera , NA and Lluch , C . 2006 . Alleviaton of salt stress in common bean (Phaseolus vulgaris) by exogenous abscisic acid supply . J Plant Grow Regul. , 25 : 110 – 119 .
- Khadri , M , Tejera , NA and Lluch , C . 2007 . Sodium chloride-ABA interaction in two common bean (Phaseolus vulgaris) cultivars differng in salinity tolerante . Environ Exp Bot. , 60 : 211 – 218 .
- La Rue and Child . 1978 . Sensitive fluorometric assay for leghemoglobin . Annal Biochem . 92 : 11 – 15 .
- Levi , C and Preiss , AS . 1978 . Amylopectin degradation in pea chloroplast extracts . Plant Physiol. , 61 : 218 – 220 .
- Lodwinsg , E and Poole , P . 2003 . Metabolism of Rhizobium bacteroids . Crit Rev Plant Sci. , 22 : 37 – 78 .
- Mansour , MMF . 2000 . Nitrogen containing compounds and adaptation of plants to salinity stress . Biol Plant. , 43 : 491 – 500 .
- Matamoros , MA , Dalton , DA , Ramos , J , Clemente , MR , Rubio , MC and Becana , M . 2003 . Biochemistry and molecular biology of antioxidants in the rhizobia-legume symbiosis . Plant Physiol. , 133 ( 2 ) : 499 – 509 .
- Morell , M and Copeland , L . 1984 . Enzymes of sucrose breakdown in soybean nodules: Alkaline invertase . Plant Physiol. , 74 : 1030 – 1034 .
- Morell , M and Copeland , L . 1985 . Sucrose synthase of soybean nodules . Plant Physiol. , 78 : 149 – 154 .
- Munns , R . 2002 . Comparative physiology of salt and water stress . Plant Cell Environ. , 25 : 239 – 250 .
- Nomura , M , Mai , HT , Fujii , M , Hata , S , Izui , K and Tajima , S . 2006 . Phosphoenolpyruvate carboxylase plays a crucial role in limiting nitrogen fixation in Lotus japonicus nodules . Plant Cell Physiol. , 47 : 613 – 621 .
- Ocaña , A , Caba , JM , Ligero , F and Lluch , C . 1998 . Nitrogen fixation in Vicia faba root nodules over ontogeny . Isr J Plant Sci. , 47 : 35 – 41 .
- Quero , G , Melchiorre , M , Lascano , R , Parola , R , Trippi , V and Racca , R . 2007 . Differential tolerance to salinity in Lotus japonicus MG20 with choroplastic Mn-SOD and GR overexpression and parental genotypes of Lotus genera . Lotus Newsletter. , 37 : 31 – 33 .
- Rigaud , J and Puppo , A . 1975 . Indole-3-acetic acid catabolism by soybean bacteroids . J Gem Microbiol. , 88 : 223 – 228 .
- Salminen , SO and Streeter , JG . 1992 . Labeling of carbon pools in Bradyrhizobium japonicum and Rhizobium leguminosarum bv viciae bacteroids following incubation of intact nodules with 14CO2 . Plant Physiol. , 100 : 597 – 604 .
- Scheible , WR , Krapp , A and Stitt , M . 2000 . Reciprocal diurnal changes of phosphoenolpyruvate carboxylase expression and cytosolic pyruvate kinase, citrate synthase and NADP-isocitrate dehydrogenase expression regulate organic acid metabolism during nitrate assimilation in tobacco leaves . Plant Cell Environ. , 23 : 1155 – 1167 .
- Serraj , R and Drevon , JJ . 1998 . Effect of salinity and nitrogen source on growth and nitrogen fixation in alfalfa . J Plant Nutr. , 21 : 1805 – 1818 .
- Serraj , R . 2002 . Response of symbiotic nitrogen fixation to drought and salinity stresses . Physiol Mol Biol Plants. , 8 ( 1 ) : 77 – 86 .
- Soussi , M , Ocaña , A and Lluch , C . 1998 . Effects of salt stress on growth, photosynthesis and nitrogen fixation in chick-pea (Cicer arietinum L.) . J Exp Bot. , 49 : 1329 – 1337 .
- Soussi , M , Lluch , C and Ocaña , A . 1999 . Comparative study of nitrogen fixation and carbon metabolism in two chickpea (Cicer arietinum L.) cultivars under salt stress . J Exp Bot. , 50 : 1701 – 1708 .
- Sprent , J . 1980 . Root nodule anatomy, type of export product and evolutionary origin in some leguminosae . Plant Cell Environ. , 3 : 35 – 43 .
- Streeter , JG , Lohnes , DG and Fioritto , RJ . 2001 . Pattern of pinitol accumulation in soybean plants and relationships to drought tolerance . Plant Cell Environ. , 24 : 429 – 438 .
- Taji T , Ohsumi C , Iuchi S , Seki M , Kasuga M , Kobayashi M , Yamaguchi-Shinozaki K , Shinozaki K. 2000 . Important roles of drought- and cold-inducible genes for galactinol synthase in stress tolerance in Arabidopsis thaliana . XIII International conference of Arabidopsis Research, Seville .
- Tejera NA , Soussi M , Lluch C. 2006 . Physiological and nutritional indicators of tolerance to salinity in chickpea plants growing under symbiotic conditions . Environ Exp Bot . 58 : 17 – 24 .
- Witty , JF and Minchin , FR . 1998 . Methods for the continuous measurement of O2 consumption and H2 production by nodulated legume root systems . J Exp Bot. , 49 : 1041 – 1047 .
- Yemm , EW and Cocking , EC . 1955 . The determination of amino acids with nihydrin . Analystic. , 80 : 209 – 213 .
- Zahran , HH . 1999 . Rhizobium-legume symbiosis and nitrogen fixation under severe conditions and in an arid climate . Microbiol Mol Biol Rev. , 63 : 968 – 989 .