Abstract
The effect of tobamovirus (TMV and ToMV) infection on changes in antioxidant enzymes, endogenous salicylic acid, endogenous hydrogen peroxide, lipid peroxidation and protein oxidation was studied in compatible (tomato and bell pepper) and incompatible hosts (Nicotiana glutinosa and Nicotiana tabacum cv. xanthi). A time course study of antioxidant enzymes such as peroxidase and catalase was studied in both interactions. Along with these studies, a time course change of endogenous hydrogen peroxide in both compatible and incompatible hosts against tobamoviruses was carried out spectrophotometrically. Histochemical changes in hydrogen peroxide were studied by using Starch-potassium iodide (KI) method. The endogenous levels of salicylic acid were quantified at 24 hours post inoculation (hpi). The effect of tobamoviruses on the lipid peroxidation levels along with protein oxidation was also studied at 24 hpi. The results showed increased peroxidase, salicylic acid, lipid peroxidation, protein oxidation, hydrogen peroxide and decreased catalase in incompatible host-tobamovirus interaction in comparison to compatible hosts. In incompatible hosts, more numbers of cells showed hydrogen peroxide accumulation at 24 hpi. These results suggested the involvement of antioxidant enzymes, salicylic acid, hydrogen peroxide and oxidative stress in incompatible host defense against tobamoviruses. As a consequence of tobamovirus infection, an oxidative stress, indicated by the increase in lipid peroxidation was observed in incompatible interaction. Slight changes in enzymes, lipid peroxidation and hydrogen peroxide in incompatible hosts indicate that these changes have roles in susceptible hosts.
Introduction
Tobacco mosaic tobamovirus (TMV) and Tomato mosaic tobamovirus (ToMV) are very serious pathogens of pepper and tomato (Waterworth and Hadidi Citation1998). Being seed-borne, both these viruses also affect the movement of seed materials. Yield reductions up to 90% have been reported in pepper infected with TMV in association with other viruses (Escudero Citation1996). The yield of tomato crops can be reduced by up to 25% due to ToMV infection (Candilo et al. Citation1992).
Primary hosts of TMV are Nicotiana tabacum (tobacco), Lycopersicon esculentum (tomato), Capsicum annuum (bell pepper). Similarly, primary hosts of ToMV are Lycopersicon esculentum (tomato), Capsicum annuum (bell pepper), Capsicum frutescens (chilli). Inoculation of TMV to Nicotiana tabacum cvs. Turkish, Sylvestris, Turkish Samsun, Samsun, White Burley and Burley produce systemic infection whereas inoculation to N. glutinosa, N. tabacum cvs. Samsun NN, Xanthi NN and Xanthi-nc, plants with the N genotype, and Chenopodium amaranticolor, C. quinoa, and Phaseolus vulgaris cv. Pinto, form necrotic lesions. Similarly inoculation of ToMV to N. glutinosa and N. tabacum cv. xanthi NN and Xanthi-nc produces necrotic lesions (Source: CABI). In the present study, the plants such as tomato and bell pepper plants, which produce systemic infection upon inoculation with TMV and ToMV were used as compatible hosts whereas N. glutinosa and N. tabacum cv. xanthi plants were used as incompatible hosts.
Peroxidases (EC 1.11.1.7) are oxido-reductive enzymes that participate in the cell wall–building processes such as oxidation of phenols, suberization, and lignification of host plant cells during the defense reaction against pathogenic agents (Chittoor et al. Citation1999). Peroxidase is involved in the hypersensitive response and plays an active role in the H2O2-dependent polymerization of hydroxycinnamoyl alcohols (monolignols) during the final stages of lignin biosynthesis. Peroxidase also catalyzes rapid, H2O2-dependent cross-linking of cell wall proteins such as the hydroxyproline rich glycoproteins and proline rich glycoproteins. Enhanced peroxidase activity has been correlated with resistance in different plants inoculated with phytopathogens. Peroxidase activity rather delayed or remained unchanged during the compatible interaction in susceptible plants (Mohammadi and Kazemi Citation2002).
Catalase (EC 1.11.1.6) scavenges hydrogen peroxide (H2O2) and it directly dismutates to H2O (Chen et al. Citation1993). Salicylic acid involved in plant defense responses binds and inhibits catalase activity and consequently elevates cellular levels of H2O2. A protein similar in sequence and activity to catalase in tobacco leaves, which indeed bound to salicylic acid (SA), effecting an increase in H2O2 levels, suggests that the induction of pathogenesis-related gene expression by H2O2 is dependent on SA (Goy et al. Citation1992). It is reported that H2O2 from the oxidative burst acts as a trigger for cell death (Tenhaken et al. Citation1995), which also makes it a source of signal molecules (Gechev and Hille Citation2005). Hydrogen peroxide is produced by plant cells at the level of the plant cell wall (Bestwick et al. Citation1997) where it is required for several peroxidase-mediated reactions (Otte and Barz Citation1996).
H2O2 is a widespread molecule in many biological systems. It is created enzymatically in living cells during various oxidation reactions and by leakage of electrons from the electron transport chains. It can act as a signaling molecule that regulates plant development, stress adaptation, and programmed cell death (PCD) (Apel and Hirt Citation2004). H2O2-induced PCD itself is very much required for a number of developmental processes and environmental responses, including aleurone cell death and the hypersensitive response to pathogens (Bethke and Jones Citation2001; Apel and Hirt Citation2004).
Salicylic acid (SA), a plant hormone widely distributed in angiosperms, is known to be a key element in pathogen defense and its simple and accurate quantification in plants is therefore of prominent importance. Hypersensitive response, systemic acquired resistance, pathogenesis-related (PR) gene expression, oxidative burst, and programmed cell death are the various resistance mechanisms involved by SA signaling. Furthermore, SA seems to induce resistance to viruses by inhibition of viral replication and systemic movement (Singh et al. Citation2004).
Several groups have reported the effects of virus infection on plant antioxidant systems. Virus infection has also been shown to increase peroxidase (POX) activity, which can utilize H2O2 as a substrate for cell wall cross-linking (Montalbini et al. Citation1995). Several studies have indicated that, in incompatible interactions, ROS are key mediators of programmed cell death during the hypersensitive response (Vranova et al. Citation2002). In contrast with incompatible reactions, little is known about the involvement of ROS in symptom development and pathogenesis in compatible plant–virus interactions. Oxidative stress in compatible virus-host plant interactions was studied in Cucumis sativus and Cucurbita pepo plants infected with cucumber mosaic virus and zucchini yellow mosaic virus (Riedle-Bauer Citation2000). The findings of Love et al. (Citation2005), implicate reactive oxygen species and ethylene in signaling in response to Cauliflower Mosaic Virus (CaMV) infection.
With this background the present study was carried out to gain more information on the possible changes of endogenous hydrogen peroxide and activity of antioxidant enzymes during systemic virus infections (compatible hosts) and hypersensitive infections (incompatible interaction). The present study was carried out to elucidate the effect of TMV and ToMV on the activities of antioxidant enzymes, endogenous SA level and H2O2. The study also aimed at study of the isoforms of peroxidase at different time intervals post-inoculation of viruses. Changes in endogenous H2O2 concentration were estimated by using spectrophotometer method in both the interactions. In addition localization of H2O2 in compatible and incompatible hosts was studied by starch-KI method. The study also includes changes in lipid peroxidation and protein oxidation levels in both the interactions.
Materials and methods
Maintenance of host plants, indicator plants and viruses
The systemic host plants tomato, bell pepper and local lesion indicator plants Nicotiana glutinosa and Nicotiana tabacum cv. xanthi-nc were maintained in an insect-proof screenhouse. TMV was maintained on bell pepper plants and ToMV on tomato plants.
Preparation of standard virus inoculum
The leaves showing mosaic symptoms were harvested from the virus-infected plants. The leaves were homogenized in the potassium phosphate buffer (pH 7.2, 0.1M) in a pre-chilled pestle and mortar. After homogenization, the extract was filtered through muslin cloth and the supernatant was used as the source of inoculum.
Time course expression of antioxidant enzymes
The leaf samples were harvested at six-hour intervals. The changes in antioxidant enzymes such as peroxidase and catalase activity at different time intervals were studied.
Determination of peroxidase activity
The leaf sample (0.1 g) was extracted with potassium phosphate buffer (pH 7.0, 0.1M) in a pre-chilled pestle and mortar. The extracted sample was centrifuged at 4°C at 10,000 rpm for 10 min. The protein content in the supernatant was estimated by using dye-binding method (Bradford Citation1976). The reaction mixture, 3 ml of phosphate buffer along with 0.25% Guaiacol (v/v), was taken in the spectrophotometer sample cuvette along with 40 µl crude extract sample and 40 µl of substrate H2O2 (10 mM) was added. The reaction was measured spectrophotometrically at 470 nm (Hammerschmidt et al. Citation1982). Peroxidase activity was expressed as units mg−1 protein−1 min−1.
Determination of catalase activity
By using phosphate buffer (0.1 M, pH 7.0), leaf sample (0.1 g) was extracted in pre-chilled pestle and mortar. The extracted sample was centrifuged at 4°C at 10,000 rpm for 10 min. The protein content in the supernatant was estimated by using dye-binding method (Bradford Citation1976). The reaction mixture containing 3 ml of Phosphate buffer along with 40 µl crude extract was taken in the spectrophotometer sample cuvette and 40 µl of H2O2 substrate was added. The reaction was measured spectrophotometrically at 240 nm. Catalase activity was expressed as units mg−1 protein−1 min−1 (Havir and Mettale Citation1987).
Spectrophotometric determination of H2O2
Endogenous H2O2 present in the extracts of compatible and incompatible seedlings harvested at different time intervals after inoculation with TMV or ToMV was estimated according to the procedure of Frew et al. (Citation1983).
The sample was prepared by homogenizing the tissue in 0.1M phosphate buffer pH.6.9. The extracted sample was centrifuged at 4°C at 10,000 rpm for 10 min and the supernatant was taken. The extract (40 µl) was taken for estimating H2O2 and 3 ml of reagent solution (100 ml contains 0.234 g of phenol, 0.1 g of 4-aminoantipyrine, 1 ml of 0.1 M phosphate buffer pH 6.9) was used to estimate the H2O2. The quantified amount of H2O2 is compared with standard graph. Quantified H2O2 is expressed in µM g−1 fresh weight.
Histochemical detection of H2O2 in compatible and incompatible host leaves
Histochemical detection of hydrogen peroxide (H2O2) in leaf tissues was carried out by following the method of Olson and Varner (Citation1993). The peelings were placed in freshly prepared aqueous solution of 50 mM potassium iodide in 4% aqueous solution of soluble starch. After incubation for 45 min, the epidermal peelings were mounted in 10% glycerol and observed under the microscope. H2O2 was localized due to dark blue coloration in the periplasmic space of the cells. The number of cells with H2O2 in 20 random microscopic fields was counted and percentage calculated (Bestwick et al. Citation1997).
Quantification of endogenous salicylic acid
One gram of leaf tissue was collected after 24 hpi of tobamoviruses and ground in 2.5 ml of 90% methanol using a pre-chilled pestle and mortar. The extract was centrifuged at 12,000 g for 15 min. The pellet was resuspended in 100% methanol (v/v) and re-extracted at 12,000 g for another 15 min. Supernatants from both extractions were combined and dried under liquid N2. The residue was resuspended in 2.5 ml of 5% trichloroacetic acid (v/v) and filtered. The filtrate was partitioned with 5 ml of 1:1 (v/v) mixture of ethyl acetate/cyclohexane containing 1% (v/v) isopropanol. The top organic phase was harvested, and then dried under liquid N2. The residue was resuspended in 23% methanol in 20mM sodium acetate (pH 5.0). Salicylic acid was quantified by fluorescence using HPLC. Fifty microlitres of sample were injected onto a HPLC (model LC-10A, Shimadzu) on a reverse phase shimpak C18 column (4.6×280 mm, Shimadzu). Salicylic acid was separated isocratically with 23% (v/v) methanol in 20 mM sodium acetate buffer (pH 5.0) at a flow rate of 1 ml/min. Salicylic acid in samples was determined with a HPLC spectrofluorescence detector equipped with a 200-watt xenon-mercury continuous lamp and a 360 nm cut-off filter. An excitation wavelength was 313 nm and an emission wavelength of 405 nm was used to quantify the SA concentration as described by Raskin et al. (Citation1989). Quantification was determined over the linear range (10–1000 ng ml−1) of the calibration curve for salicylic acid sodium salt (Sigma).
Estimation of oxidative stress
Quantification of the Thiobarbituric Acid Reactive Substances (TBARS) (to study the levels of lipid peroxidation) and carbonyl (CO) proteins (to study the levels of protein oxidation) were used as indicators of oxidative stress in the compatible and incompatible hosts at 24 hpi.
Estimation of lipid peroxidation
Lipid peroxidation was estimated by using fluorometric method (Yagi Citation1976). The tobamovirus challenge inoculated leaf sample was harvested 24 h after inoculation along with proper control. The sample was extracted by using phosphate buffer (pH 7.0, 0.1 M) in pre-chilled pestle and mortar. The extracted sample was centrifuged at 4°C at 10,000 rpm for 10 min and the supernatant was taken. Sample (0.1 ml), 0.2 ml of 8.1% SDS (w/v), 1.5 ml of 20% acetic acid (v/v) and 1.5 ml of 0.8% TBA (dissolved in water) (w/v) was heated at 95°C at 60 min in water bath. Five milliliters of butanol was added to the solution and centrifuged at 4000 rpm for 10 min and the upper layer was taken and absorbance was measured in spectrofluorimeter (Hitachi F-2000 fluorescence spectrophotometer) with excitation at 515 nm and emission at 553 nm. Tetra ethyl peroxidate (TEP) was used as the standard at different concentrations. To the standard, 4 ml of N/12 sulphuric acid and 1 ml TBA was added. The solution was boiled at 90°C for 60 min and 5 ml of butanol was added. The solution was centrifuged at 4000 rpm for 10 min and the upper layer was taken and absorbance was measured in spectrofluorimeter with excitation at 515 nm and emission at 553nm.
Estimation of protein oxidation
The crude protein extracted from the leaves harvested at 24 hpi of tobamoviruses was used. The protein solution (0.1 mg) taken in the eppendorf tube was precipitated with trichloroacetic acid (TCA). To each tube, 500 µl of 10 mM 2,4- dinitrophenylhydrazine in 2M HCl was added and allowed to stand at room temperature for 1 h, with vortexing every 10–15 min. Trichloroacetic acid (20% (v/v), 500 µl) was added and tubes were centrifuged at 11,000 g for 3 min, and the supernatant was discarded. The obtained pellet was washed with 1 ml ethanol-ethyl acetate (1:1) to remove free reagent, allowing the sample to stand for 10 min before centrifugation and supernatant was discarded at each time (the step was repeated for three times). The precipitated protein was redissolved in 100 mM NaOH at 37°C for 15 min. The insoluble material was removed by centrifuging at 10,000 rpm for 3 min. The spectrum was obtained by reading against the complementary blank. The carbonyl content was estimated by using the maximum absorbance (360–390 nm) using a molar absorption coefficient of 22,000 M−1 cm−1.
Data analysis
For data generated from the quantification of hydrogen peroxide was analyzed by using the statistical computer application package SPSS 10.0 was employed. The data generated were average of three independent experiments having three replicates each. Data were subjected to analysis of variance (ANOVA) and the means were compared for significance using Duncan's new Multiple Range Test (DMRT; p=0.05). The data generated in other studies were the average of three independent experiments having three replicates each and standard error was calculated.
Results
Temporal changes in peroxidase and catalase activity in compatible and incompatible seedlings inoculated with tobamoviruses
The temporal changes in peroxidase and catalase activity during infection in compatible (tomato and bell pepper) and incompatible (N. glutinosa and N. tabacum cv. xanthi-nc) host seedlings due to tobamovirus inoculation was studied. The inoculated samples were drawn at hourly intervals up to 30 h. The seedlings inoculated with buffer served as control. A differential peroxidase and catalase activity was observed after inoculation of seedlings in both the compatible and incompatible interaction. In the incompatible interaction, however, peroxidase activity increased significantly over buffer whereas catalase activity decreased compared with control.
Maximum peroxidase activity was noticed at 24 hpi of viruses in both compatible and incompatible host-virus interaction. An increase of 3.75-fold and 2.25-fold peroxidase activity was noticed in TMV and ToMV-inoculated tomato seedlings in comparison with control seedlings (a). In TMV and ToMV-inoculated bell pepper seedlings, 0.2 and 0.75-fold increase in peroxidase activity was observed in comparison with control seedlings (a). Nicotiana glutinosa seedlings inoculated with TMV and ToMV showed an increase of 0.6 and 2.25-fold peroxidase activity compared to control seedlings (a). In N. tabacum cv. xanthi-nc seedlings, inoculation of both the viruses showed a 2.25-fold increase in comparison with controls seedlings (a).
Figure 1. (a–c) Changes in peroxidase, catalase and hydrogen peroxide in tomato seedlings inoculated with TMV and ToMV. The data represents mean of three replicates±SE.
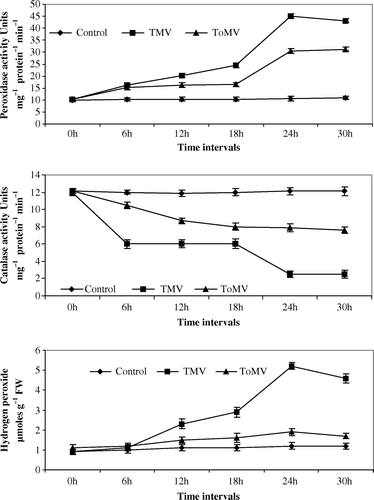
Figure 2. (a–c) Changes in peroxidase, catalase and hydrogen peroxide in bell pepper seedlings inoculated with TMV and ToMV. The data represents mean of three replicates±SE.
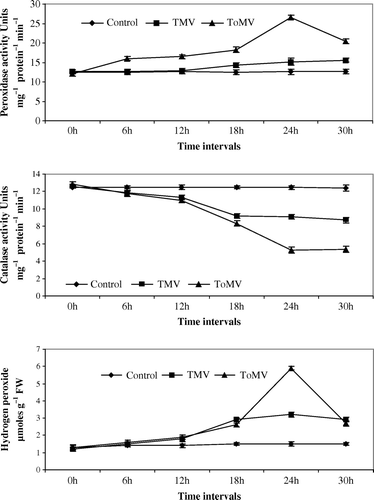
Figure 3. (a–c) Changes in peroxidase, catalase and hydrogen peroxide in N. glutinosa seedlings inoculated with TMV and ToMV. The data represents mean of three replicates±SE.
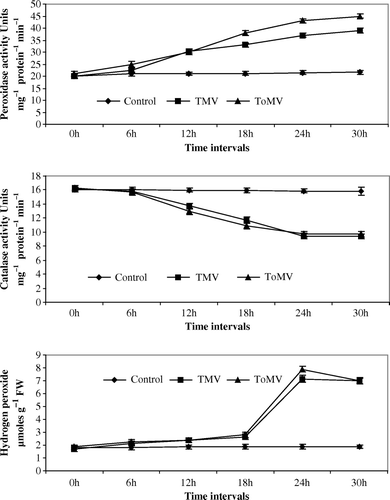
Figure 4. (a–c) Changes in peroxidase, catalase and hydrogen peroxide in N. tabacum Cv. xanthi seedlings inoculated with TMV and ToMV. The data represents mean of three replicates±SE.
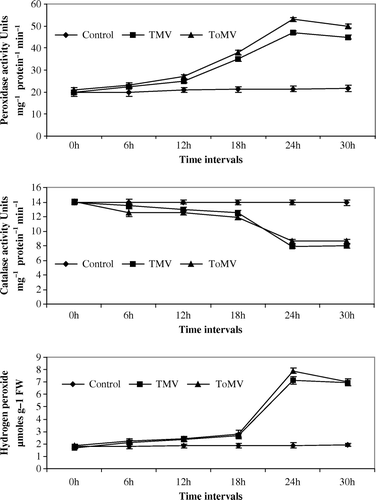
The catalase activity was lowest at 24 hpi in both compatible and incompatible host-virus interaction. In TMV and ToMV-inoculated tomato seedlings, 3-fold and 1-fold decline in catalase activity was observed in comparison with control seedlings (b). A decrease of 1.8-fold and 7.2-fold catalase activity was noticed in bell pepper seedlings inoculated with TMV and ToMV, respectively, in comparison with control seedlings (b). Catalase activity decreased by 2.8-fold and 7.4-fold in N. glutinosa seedlings inoculated with TMV and ToMV when compared to control seedlings (b). In N. tabacum cv. xanthi-nc inoculation of both viruses decreased the catalase activity by 1.2-fold decline in comparison with control seedlings (b).
Hydrogen peroxide estimation and localization
Spectrophotometric estimation of endogenous H2O2 showed enhanced generation of hydrogen peroxide in incompatible hosts than in compatible hosts after inoculation with tobamoviruses. Inoculation of tomato seedlings with TMV or ToMV increased H2O2 concentration by 2.9- and 1.4-fold in comparison with control seedlings (c). An increase of 1.5-fold or 3-fold in H2O2 concentration was observed in TMV or ToMV-inoculated bell pepper seedlings when compared with control seedlings (c). Both TMV and ToMV inoculation to N. glutinosa and N. tabacum cv. xanthi-nc seedlings showed an increase of 2.8-fold and 2.3-fold H2O2 concentration in comparison to control seedlings (c and c).
Based on the spectrophotometric estimation of endogenous H2O2 a time interval of 24 h was used to study the H2O2 localization. The percent of H2O2 localized cells was highest in the incompatible hosts in comparison to compatible host at 24 hpi of tobamoviruses. Tomato seedlings and bell pepper seedlings showed 59 and 52% H2O2 localization after inoculation with TMV and ToMV, respectively ( and ). It was observed that the N. glutinosa showed 71 and 68% H2O2-localized cells after inoculation with TMV and ToMV, respectively. In the case of N. tabacum cv. xanthi-nc, inoculation of tobamoviruses showed 64% (TMV) and 62% (ToMV) H2O2 localized cells ( and ).
Figure 5. Localization of hydrogen peroxide in tissues of tomato and bell pepper leaves on inoculation with TMV and ToMV at 24 hpi.
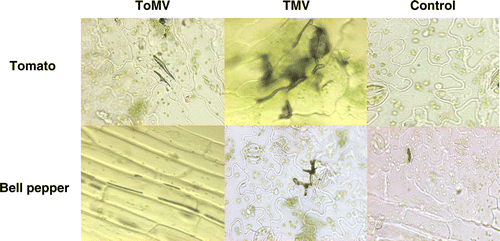
Figure 6. Localization of hydrogen peroxide in tissues of Nicotiana glutinosa and Nicotiana tabacum cv. xanthi leaves on inoculation with TMV and ToMV at 24 hpi.
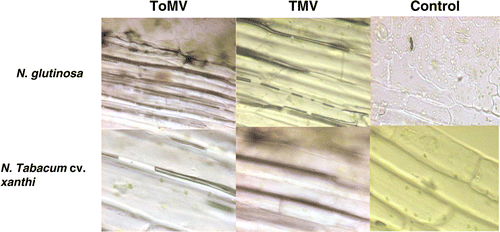
Table 1. Quantitative assessment of hydrogen peroxide (H2O2) localization in the tissues of tomato, bell pepper and N. glutinosa and N. tabacum cv. xanthi seedlings inoculated with TMV and ToMV at 24 hpi.
Endogenous SA quantification
Leaf samples were collected 24 hpi. Endogenous levels of SA was more in tomato leaves inoculated with TMV (23.5±0.9 ng) in comparison with control and ToMV-inoculated leaves. Similarly, ToMV-inoculated bell pepper leaves (4.6±0.14 ng) showed slightly more levels of SA when compared to control and TMV-inoculated leaves. Inoculation of TMV (21.4±0.65 ng) or ToMV (25.6±0.67 ng) showed significantly higher levels of endogenous SA level in N. xanthi-nc plants. The leaves of N. glutinosa inoculated with TMV (5.7±0.23 ng) showed more accumulation of endogenous SA when compared to ToMV-inoculated leaves (a).
Estimation of oxidative stress (lipid peroxidation and protein oxidation)
Tomato plants inoculated with TMV (39±0.47 nanomoles) at 24 hpi showed higher levels of lipid peroxides in comparison with ToMV-inoculated tomato seedlings. TMV-inoculated bell pepper seedlings showed lower levels of lipid peroxidation in comparison with ToMV (70±0.36 nanomoles). Both N. glutinosa and N. tabacum cv. xanthi-nc seedlings showed more or less similar levels of lipid peroxides in TMV- or ToMV-inoculated plants (b)
Inoculation of TMV to tomato or ToMV to bell pepper showed more protein oxidation as evident by quantifying the carbonyl proteins (CO-Proteins) in comparison with ToMV-tomato and TMV-bell pepper interactions. Inoculation of N. glutinosa with TMV showed more protein oxidation level (20±0.93 nanomoles) in comparison with ToMV-inoculated seedlings. Contrastingly, ToMV inoculation (25±0.94 nanomoles) to N. tabacum cv. xanthi-nc seedlings showed more protein oxidation levels when compared to TMV-inoculated seedlings (c).
Discussion and conclusion
Inoculation with TMV and ToMV induced a slight increase in the amount of peroxidase activity when compared to uninoculated seedlings in both bell pepper and tomato, respectively. Resistant ones (incompatible) had greater peroxidase activity when compared to susceptible ones (compatible), with infected plants having higher peroxidase levels than noninfected plants. In order to ensure that the activity of isozymes observed on gels reflected the changes found in peroxidase enzymes were assayed spectrophotometrically in extracts. Similar induction in total peroxidase activity was noticed in cucumber mosaic virus and zucchini yellow mosaic virus-infected Cucumis sativus and Cucurbita pepo plants (Riedle-Bauer Citation2000). Similar studies were conducted by Kiraly et al. (Citation2002), using two local lesion hosts of tobacco mosaic virus (TMV), namely in wild-type Xanthi-nc tobacco and in NahG transgenic tobacco, the latter of which is not able to accumulate salicylic acid (SA) and therefore is unable to develop systemic acquired resistance (SAR). The majority of antioxidant enzymes studied were less active in uninfected NahG tobacco than in Xanthi-nc. After infection, a greater accumulation of superoxide and H2O2, and a more intensive necrotization was positively correlated with the reduced capability of NahG leaf tissue to detoxify reactive oxygen species (Kiraly et al. Citation2002).
Oxidative stress in compatible virus-host plant interactions was studied in cucumber mosaic virus and zucchini yellow mosaic virus-infected Cucumis sativus and Cucurbita pepo plants. Furthermore, in infected plants an induction of superoxide dismutases, catalases, total peroxidases and ascorbate peroxidases was observed. All the peroxidase isoforms detected in Cucumis sativus and Cucurbita pepo, however, not only functioned as radical scavengers but also catalyzed the formation of H2O2. Thus, it can be presumed that the enhancement of peroxidases contributes to the oxidative stress in systemic plant-virus interactions. By way of oxidation of indole-3-acetic acid, upregulated peroxidases might also be responsible for growth reductions and malformations in virus-infected plants (Riedle-Bauer Citation2000). Catalase is an enzyme directly involved in the detoxification of H2O2. The decline in catalase activity in P. vulgaris infected with WClMV was similar to that reported for tobacco infected with TMV. Catalase (CAT) activity has been shown to decline following virus infection of a susceptible host and this is believed to lead to increased H2O2 levels, which might in turn lead to the induction of SAR (Neuenschwander et al. Citation1995).
Another well-known defense mechanism involves the generation of reactive oxygen species (ROS) following infection. ROS have been implicated as signaling intermediates during the PCD that typically occurs locally around sites of infection by incompatible pathogens (Hancock et al. Citation2002). Locally increased activity of enzymes associated with the generation of ROS has been detected in resistant tobacco (Nicotiana tabacum) cultivars infected with TMV (Sagi and Fluhr Citation2001). Increased levels of enzymes associated with the detoxification of ROS have also been demonstrated in bean (Phaseolus vulgaris) plants undergoing compatible infection with White clover mosaic virus (Clarke et al. Citation2002). Increases in ROS during incompatible infections with TMV have been detected directly using paramagnetic resonance spectroscopy (Fodor et al. Citation2001) and ROS-reactive fluorescent probes (Allan et al. Citation2001). In the latter case, a ROS burst was detected in tobacco epidermal cells within seconds of application of TMV to the outside of leaves of various tobacco cultivars. Interestingly, ROS bursts occurred with both resistant and susceptible tobacco cultivar-TMV isolate combinations (Love et al. Citation2005). In contrast to incompatible reactions, little is known about the involvement of ROS in symptom development and, pathogenesis in compatible plant-virus interactions. Some recent works showed that increased levels of ROS could also contribute to the symptom development and pathogenesis in compatible plant-virus interactions, as described recently in plum pox virus (PPV) susceptible peach plants (Hernandez et al. Citation2006).
Maximum expression of H2O2 was noticed in incompatible seedlings and its reduced expression in the compatible hosts recorded in the present study are in accordance with the suggestion that HR and HR-related pathways are more important in resistance against host-specific plant viruses. It is reported that H2O2 from the oxidative burst acts as a trigger for cell death, which also makes it a source of signal molecules (Gechev and Hille Citation2005). When the total number of cells showing H2O2 localization in each test seedling were considered, it was observed that the number of cells localized in incompatible hosts were greater than the cells localized in the compatible hosts. Occurrence of cell death as a result of H2O2 accumulation and requirement of a threshold quantity of H2O2 for occurrence of cell death has been reported (Greenberg Citation1996). Earlier, Doke and Ohashi (Citation1988) reported that high concentrations of H2O2 were noticed in the resistant tobacco plants inoculated with tobacco mosaic virus, H2O2 appeared responsible for an intensive HR in resistant tobacco plants inoculated with TMV. H2O2 has been reported to act upstream of SA (Neuenschwander et al. Citation1995) in tobacco plants expressing systemic acquired resistance.
Our results correlated with the results obtained by the Xi et al. (Citation2007), whose study showed that synergistic effects between CMV and tobacco necrosis virus (TNV) revealed the changes in the extent of lipid peroxidation and contents of H2O2. The results revealed that synergistic infection by CMV- and TNV-induced higher increases in the levels of malonyldialdehyde (MDA), hydrogen peroxide (H2O2) and more decline in the activities of catalase than singly infected ones. Both peroxidase and superoxide dismutase activities increased rapidly for the first 10 days post inoculation (dpi) in doubly-infected plants and then declined, whereas the enzyme activities continued to increase after 10 dpi in singly infected plants and had higher enzyme activities in the late stages than that of co-infected plants (Xi et al. Citation2007). The inoculation of TNV and CMV individually showed the increase in H2O2 levels. Similarly, the MDA level was more in the doubly infected than those of singly infected plants. The catalase activities declined in all the virus infected plants following inoculation. In contrast with what was observed for CAT activities, activities of POX in infected N. benthamiana plants tended to increase in the first 10 dpi for viruses-infected plants. After that, POX activities of TNV/CMV inoculated plants declined steadily, whereas the activities of TNV singly-inoculated plants declined until day 15 and increased continuously thereafter. The response of CMV-infected plants was laggard, while the POX activities increased gradually (Xi et al. Citation2007).
The quantification of SA revealed that, in incompatible interactions, the accumulation is more than those of the compatible interactions. The central role of salicylic acid in mediating defense against a wide variety of biotrophic pathogens is now well established (Alvarez Citation2000). Increasing evidence indicates that these responses are also important in defense during both incompatible and compatible virus infections, and that they appear to function through an NPR1-independent pathway (Murphy and Carr Citation2002; Takahashi et al. Citation2002). These responses can also restrict the spread of some RNA viruses during compatible infections. Chivasa et al. (Citation1997) postulated a virus-specific defense pathway in which reduced electron flow through the mitochondrion in response to increased SA levels stimulates the generation of reactive oxygen species (ROS). These act as signaling intermediates stimulating defense responses (Gilliland et al. Citation2003; Singh et al. Citation2004). There are a number of reports that virus infection of both compatible and incompatible hosts stimulates SA signaling. Markers for SA signaling (e.g., PR-1), are up-regulated during systemic infections by several RNA viruses (Huang et al. Citation2005). CaMV infection stimulated SA-dependent expression of PR-1, PR-2, and PR-5 in susceptible Arabidopsis ecotypes (Laird et al. Citation2004). In Arabidopsis, CaMV infection stimulates expression of markers for at least three defense-signaling pathways (Love et al. Citation2005), each with different kinetics of activation. The effect of these responses on virus multiplication or spread was uncertain, although genetic knockout of the NADPH oxidase, which abolished the rapid systemic generation of H2O2 and the activation of ROS-responsive markers, did not significantly alter susceptibility to CaMV (Love et al. Citation2007).
The significant increase in peroxidase, endogenous hydrogen peroxide, salicylic acid, lipid peroxidation and protein oxidation and a decrease in catalase activity upon challenge inoculation with viruses could be considered as a marker for studying plant-virus interactions in compatible and incompatible interactions.
References
- Allan , AC , Lapidot , M , Culver , JN and Fluhr , R. 2001 . An early tobacco mosaic virus-induced oxidative burst in tobacco indicates extracellular perception of the virus coat protein . Plant Physiol. , 126 : 97 – 108 .
- Alvarez , ME. 2000 . Salicylic acid in the machinery of hypersensitive cell death and disease resistance . Plant Molec Biol. , 44 : 429 – 442 .
- Apel , K and Hirt , H. 2004 . Reactive oxygen species: metabolism, oxidative stress, and signal transduction . Ann Rev Plant Biol. , 55 : 373 – 399 .
- Bestwick , CS , Brown , IR , Bennett , MHR and Mansfield , JW. 1997 . Localization of hydrogen peroxide accumulation during the hypersensitive reaction of lettuce cells to Pseudomonas syringae pv phaseolicola . Plant Cell. , 9 : 209 – 221 .
- Bethke , PC and Jones , RL. 2001 . Cell death of barley aleurone protoplasts is mediated by reactive oxygen species . Plant J. , 25 : 19 – 29 .
- Bradford , MM. 1976 . A rapid and sensitive method for the quantification of microgram quantities of protein utilization of the principle of protein-dye binding . Analyt Biochem. , 72 : 248 – 254 .
- Candilo , M , Faccioli , G , Grassi , G and Faeti , V. 1992 . Effect of tomato mosaic virus (ToMV) on yield of machine-harvested processing tomatoes . Phytopathol Medit. , 31 ( 1 ) : 32 – 36 .
- Chen , Z , Riigliano , JW and Klessig , DF. 1993 . Purification and characterization of a soluble salicylic acid binding protein from tobacco . Proc Natl Acad Sci USA , 90 : 9533 – 9537 .
- Chittoor , JM , Leach , JE and White , FF. 1999 . “ Induction of peroxidase during defense against plant pathogens ” . In Pathogenesis related proteins in plants , Edited by: Dutta , SK and Muthukrishnan , S . 291 Boca Raton, FL : CRC press .
- Chivasa , S , Murphy , AM , Naylor , M and Carr , JP. 1997 . Salicylic acid interferes with tobacco mosaic virus replication via a novel salicylhydroxamic acid-sensitive mechanism . Plant Cell. , 9 : 547 – 557 .
- Clarke , SF , Guy , PL , Burritt , DJ and Jameson , PE. 2002 . Changes in the activities of antioxidant enzymes in response to virus infection and hormone treatment . Physiol Plantarum , 114 : 157 – 164 .
- Doke , N and Ohashi , Y. 1988 . Involvement of an 0,- generating system in the induction of necrotic lesions on tobacco leaves infected with tobacco mosaic virus . Physiolog Mo1ec Plant Pathol. , 32 : 163 – 175 .
- Escudero , J. 1996 . Survey of viruses affecting pepper (Capsicum annuum L.) in southern Puerto Rico . J Agric Univ Puerto Rico. , 80 : 77 – 80 .
- Fodor , J , Hideg , E , Kecskes , A and Kiraly , Z. 2001 . In vivo detection of tobacco mosaic virus-induced local and systemic oxidative burst by electron paramagnetic resonance spectroscopy . Plant Cell Physiol. , 42 : 775 – 779 .
- Frew , JE , Jones , P and Scholes , G. 1983 . Spectrophotometric determination of hydrogen peroxide and organic hydropheroxides at low concentrations in aqueous solution . Analyt Chimica Acta. , 155 : 139 – 150 .
- Gechev , TS and Hille , J. 2005 . Hydrogen peroxide as a signal controlling plant programmed cell death . J Cell Biol. , 168 ( 1 ) : 17 – 20 .
- Gilliland , A , Singh , DP , Hayward , JM , Moore , CA , Murphy , AM and Carr , JP. 2003 . Genetic modification of alternative respiration has differential effects on Antimycin A-induced versus salicylic acid-induced resistance to tobacco mosaic virus . Plant Physiol. , 132 : 1518 – 1528 .
- Goy , PA , Felix , G , Metraux , JP and Meins , F Jr. 1992 . Resistance to disease in the hybrid Nicotiana glutinosa x Nicotiana debneyi is associated with high levels of beta-1, 3-glucanase, chitinase, peroxidase and polyphenoloxidase . Physiolog Molec Plant Pathol. , 41 : 11 – 21 .
- Greenberg , JT. 1996 . Programmed cell death: a way of life for plants . Proc Natl Acad Sci USA , 93 : 12094 – 12097 .
- Hammerschmidt , R , Nuckles , E and Kuc , J. 1982 . Association of enhanced peroxidase activity with induced systemic resistance of cucumber to Colletotrichum lagenarium . Physiolog Plant Pathol. , 20 : 73 – 82 .
- Hancock , JT , Desikan , R , Clarke , A , Hurst , RD and Neill , SJ. 2002 . Cell signalling following plant/pathogen interactions involves the generation of reactive oxygen and reactive nitrogen species . Plant Physiol Biochem. , 40 : 611 – 617 .
- Havir , EA and Mettale , NA. 1987 . Biochemical and developmental characterization of multiple form of catalase in tobacco leaves . Plant Physiol. , 84 : 450 – 455 .
- Hernandez , JA , Diaz-Vivancos , P , Rubio , M , Olmos , E , Ros-Barcelo , A and Martínez-Gomez , P. 2006 . Long-term PPV infection produces an oxidative stress in a susceptible apricot cultivar but not in a resistant cultivar . Physiolog Plantarum. , 126 : 140 – 152 .
- Huang , Z , Yeakley , JM , Garcia , EW , Holdridge , JD , Fan , J and Whitham , SA. 2005 . Salicylic acid-dependent expression of host genes in compatible arabidopsis-virus interactions . Plant Physiol. , 137 : 1147 – 1159 .
- Kiraly , Z , Barna , B , Kecskes , A and Fodor , J. 2002 . Down-regulation of antioxidative capacity in a transgenic tobacco, which fails to develop acquired resistance to necrotization caused by tobacco mosaic virus . Free Radical Res. , 36 : 981 – 991 .
- Laird , J , Armengaud , P , Laval , V , Giuntini , P and Milner , JJ. 2004 . Inappropriate annotation of a key defence marker in Arabidopsis: will the real PR-1 please stand up? . Planta. , 219 : 1089 – 1092 .
- Love , AJ , Laval , V , Geri , C , Laird , J , Tomos , AD , Hooks , MA and Milner , JJ. 2007 . Cauliflower mosaic virus protein P6 is a suppressor of RNA silencing . J Gen Virol. , 88 : 3439 – 3444 .
- Love , AJ , Yun , BW , Laval , V , Loake , GJ and Milner , JJ. 2005 . Cauliflower mosaic virus, a compatible pathogen of arabidopsis, engages three distinct defense-signaling pathways and activates rapid systemic generation of reactive oxygen species . Plant Physiol. , 139 : 935 – 948 .
- Mohammadi , M and Kazemi , H. 2002 . Changes in peroxidase and polyphenol oxidase activities in susceptible and resistant wheat heads inoculated with Fusarium graminearum and induced resistance . Plant Sci. , 162 : 491 – 498 .
- Montalbini , P , Buonaurio , R and Umesh-Kumar , NN. 1995 . Peroxidase activity and isoperoxidase pattern in tobacco leaves infected with tobacco necrosis virus and other viruses inducing necrotic and non necrotic alterations . J Phytopathol. , 143 : 295 – 301 .
- Murphy , AM and Carr , JP. 2002 . Salicylic acid has cell-specific effects on Tobacco mosaic virus replication and cell-to-cell movement . Plant Physiol. , 128 : 552 – 563 .
- Neuenschwander , U , Vernooij , B , Friedrich , L , Uknes , S , Kessmann , H and Ryals , J. 1995 . Is hydrogen peroxide a second messenger of salicylic acid in systemic acquired resistance? . Plant J. , 8 : 227 – 233 .
- Olson , PD and Varner , JE. 1993 . Hydrogen peroxide and lignification . Plant J. , 4 : 887 – 892 .
- Otte , O and Barz , W. 1996 . The elicitor-induced oxidative burst in cultured chickpea cells drives the rapid insolubilization of two cell wall structural proteins . Planta. , 200 : 238 – 246 .
- Raskin , I , Turner , IM and Melander , WR. 1989 . Regulation of heat-production in the inflorescences of an arum lily by endogenous salicyclic acid . Proc Natl Acad Sci USA. , 86 ( 7 ) : 2214 – 2218 .
- Riedle-Bauer , M. 2000 . Role of reactive oxygen species and antioxidant enzymes in systemic virus infections of plants . J Phytopathol. , 148 : 297 – 302 .
- Sagi , M and Fluhr , R. 2001 . Superoxide production by plant homologues of the gp91(phox) NADPH oxidase. Modulation of activity by calcium and by tobacco mosaic virus infection . Plant Physiol. , 126 : 1281 – 1290 .
- Singh , DP , Moore , C A , Gilliland , A and Carr , JP. 2004 . Activation of multiple antiviral defence mechanisms by salicylic acid . Molec Plant Pathol. , 5 : 57 – 63 .
- Takahashi , H , Miller , J , Nozaki , Y , Takeda , M , Shah , J , Hase , S , Ikegami , M , Ehara , Y and Dinesh-Kumar , SP. 2002 . RCY1, an Arabidopsis thaliana RPP8/HRT family resistance gene, conferring resistance to Cucumber mosaic virus requires salicylic acid, ethylene and a novel signal transduction mechanism . Plant J. , 32 : 655 – 667 .
- Tenhaken , R , Levine , A , Brisson , LF , Dixon , RA and Lamb , C. 1995 . Function of the oxidative burst in hypersensitive disease resistance . Proc Natl Acad Sci USA. , 92 : 4158 – 4163 .
- Vranova , E , Atichartpongkul , S , Villarroel , R , Montagu , MV , Inze , D and Camp , WV. 2002 . Comprehensive analysis of gene expression in Nicotiana tabacum leaves acclimated to oxidative stress . Proc Natl Acad Sci USA. , 99 ( 16 ) : 10870 – 10875 .
- Waterworth , HE and Hadidi , A. 1998 . “ Economic losses due to plant viruses ” . In Plant virus disease control , Edited by: Hadidi , A , Khetarpal , RK and Koganezawa , H . 1 – 13 . St Paul, Minnesota : American Phytopathological Society Press. .
- Xi , D , Feng , H , Lan , L , Du , J , Wang , J , Zhang , Z , Xue , L , Xu , W and Li , H. 2007 . Characterization of synergy between Cucumber mosaic virus and Tobacco necrosis virus in Nicotiana benthamiana . J Phytopathol. , 155 ( 9 ) : 570 – 573 .
- Yagi , K. 1976 . Simple fluorimetric assay for lipid peroxide in blood plasma . Biochem Med. , 15 : 212 – 216 .