Abstract
In the present communication, we studied the effect of light stress on pigment contents, net photosynthetic rate, electrolyte leakage, malondialdehyde concentration and various antioxidant enzymes such as superoxide dismutase (SOD), catalase (CAT), glutathione reductase (GR), and ascorbate peroxidase (APX) during acclimatization of micropropagated Tylophora indica plantlets. Pigment (Chlorophyll a, b and carotenoids) contents in ex vitro formed leaves were found significantly higher compared to the in vitro formed ones. In vitro plantlets (day 0) exhibited a low photosynthetic activity, but with the emergence of new leaves a significant increase in net photosynthetic rates was observed. Changes in the activity of the antioxidant enzymes system were also observed during the critical days of acclimatization. Plantlets showed increased levels of SOD production, indicating its preventive mechanism of membrane oxidation and damage to biological molecules in high light (HL) acclimatized plantlets. The CAT activity increased at both low lights (LL) and HL during the whole period of acclimatization. Likewise, photoexposure of plantlets at LL and HL showed elevated activity of GR and APX against 0 day plantlets.
Introduction
In vitro culture, one of the key tools of plant biotechnology, has emerged as a powerful technique in the production of true-to-type plants as well as in genetic manipulation studies. The most important and critical step in micropropagation of plants is the period of transition during the process of hardening from in vitro to ex vitro environment. Generally, in vitro conditions which promote rapid growth, shoot proliferation and plantlet development, result in certain abnormal plant characteristics (Kozai Citation1991; Pospisilova et al. Citation1997). Under in vitro condition, plantlets are exposed to low photosynthetic photon flux density (PPFD). Once in the greenhouse, PPFD is much higher and plants become very susceptible to photoinhibition. Beside photoinhibition, plants also suffer due to the differences between in vitro and ex vitro relative humidity. Synergic action of high irradiance and water stress reduces the capacity of a photosynthetic system to utilize incident radiation, and causes oxidative stress through the formation of reactive oxygen species (ROS). These include superoxide radicals (), singlet oxygen (1O2), hydrogen peroxide (H2O2) and hydroxyl radical (OH?), which cause tissue injury (Foyer et al. Citation1994). Plants have evolved various protective mechanisms to eliminate or reduce ROS. ROS are highly reactive in the absence of any protective mechanism. They can seriously disrupt normal metabolism through oxidative damage to membrane lipids, proteins, pigments and nucleic acids. In plant cells, one such protective mechanism is an antioxidant system, composed of both non-enzymatic and enzymatic antioxidants. The non-enzymatic antioxidants are generally small molecules, such as ascorbate and glutathione, that occur in the aqueous phase (intracellular fluid), whereas lipophilic antioxidants (tocopherols and carotenoids) are active in cell membranes (Noctor and Foyer Citation1998; Smirnoff and Wheeler Citation2000; Munne-Bosch and Alegre Citation2002). In the enzymatic ROS-scavenging pathways, superoxide dismutase (SOD; EC 1.15.1.1) converts
directly to hydrogen peroxide (H2O2). Furthermore, the accumulation of H2O2 is restricted through the action of catalase (CAT; EC 1.11.1.6) or by the ascorbate-glutathione cycle, where ascorbate peroxidase (APX; EC 1.11.1.11) reduces it to H2O. Finally, glutathione reductase (GR; EC 1.6.4.2) catalyzes the NADPH-dependent reduction of oxidized glutathione (GSSG) to the reduced glutathione (GSH) (Noctor et al. Citation2002). However, no report is available on the status of these protective enzymatic systems during ex vitro establishment of micropropagated plantlets of Tylophora indica under different light intensity. In fact, ultimate success of micropropagation technology, either for research experiments or for commercial scale, depends largely upon the successful control over transplanting with high survival rate. Quite understandably, the process of acclimatization (or hardening) continues to be a major bottleneck in successful field transfer. Thus, the aims of this work were to investigate the effects of light intensity on photosynthetic activity, pigment composition, electrolyte leakage, malondialdehyde content and activities of antioxidant enzymes, namely, superoxide dismutase (SOD), catalase (CAT), glutathione reductase (GR), and ascorbate peroxidase (APX) during ex vitro acclimation of Tylophora indica plantlets.
Materials and methods
Plant materials and growth conditions
Tylophora indica plantlets were micropropagated by the method described by Faisal et al. (Citation2007). After four weeks of rooting, uniform young plantlets with six–seven leaves were removed from the culture medium, washed gently under running tap water and transferred to plastic pots containing sterile soilrite. The pots were placed in a growth room maintained at 25 ± 2°C with light intensity of 50 µmol m−2 s−1 (low light; LL) and 300 µmol m−2 s−1 (high light; HL) on a 16:8 h photoperiod. Leaf samples were collected at transplanting (day 0) and after 7, 14, 21 and 28 days and frozen in liquid nitrogen for further analysis.
Photosynthetic pigments and photosynthesis
The chlorophylls (a and b) and carotenoids were extracted using the method of Mackinney (Citation1941) and Maclachlan and Zalik (Citation1963), respectively. A total of 0.5 g fresh leaf tissues from inter-vienal areas were ground in 5 ml of 80% (v/v) acetone with the help of mortar and pestle. The suspension was filtered with Whatman filter paper number 1. Absorbance of the solution was then measured at 645 and 663 for chlorophylls and at 480 and 663 nm for carotenoid on a UV-vis spectrophotometer (UV 1700 Pharma-Spec, Shimadzu, Japan). The chlorophyll and carotenoid contents were estimated using the formula given by Arnon (Citation1949). Net photosynthetic rate (P N ) of in vitro regenerated plantlets was measured weekly (0, 7, 14, 21 and 28 days) in fully expanded leaves using a portable Infra Red Gas Analyzer (IRGA, LICOR-6400, Lincoln, NE, USA) at PPFD of 50 and 300 µmol m−2s−1. The net photosynthetic rate was expressed as µmol CO2 m−2s−1.
Electrolyte leakage
Electrolyte leakage was used to assess membrane permeability, as described by Lutts et al. (Citation1995). Samples were washed three times with double distilled water (DDW) to remove the surface contamination. The young leaves were cut using a punch plyer and placed in a closed vial containing 10 ml of DDW and incubated on a rotatory shaker for 24 h; subsequently, the electrical conductivity of the solution (EC1) was determined. Samples were autoclaved at 120°C for 20 min and their electrical conductivity (EC2) was noted after cooling the solution at room temperature. The electrolyte leakage was calculated as
Evaluation of lipid peroxidation
Malondialdehyde concentration was determined by a modified version of the method described by Cakmak and Horst (Citation1991). A total of 0.5 gram of fresh tissue was ground in 10 ml 0.1% (w/v) TCA (trichloroacetic acid) and centrifuged at 15,000 rpm for 5 min. One ml of the supernatant taken in a separate test tube was added with 4.0 ml of 0.5% (w/v) TBA made in 20% (w/v) TCA. The mixture was heated at 95°C for 30 min, cooled quickly in an ice bath and centrifuged at 12,000 rpm for 5 min. The absorbance of the supernatant was read at 532 nm on a spectrophotometer and corrected for non-specific turbidity by subtracting the absorbance at 600 nm. The value obtained was used for calculations by the following formula:
where ε is the specific extinction coefficient (= 155 mM−1cm−1), V is the volume of extraction medium, W is the fresh weight of leaf, A600 is the absorbance at 600 nm wavelength, and A532 is the absorbance at 532 nm wavelength.
Antioxidant enzyme extraction and assay
For determination of antioxidant enzyme activities, 0.5 g of fresh leaf samples were homogenized in 2.0 ml of potassium phosphate buffer containing 1% (w/v) polyvinylpyrrolidone (PVP), 1% (w/v) Triton X-100 and 5.5% (w/v) of EDTA using pre-chilled mortar and pestle. The homogenate was filtered through four layers of cheesecloth and centrifuged at 15,000 rpm for 20 min. The supernatant was used for enzyme assays. All the steps of extraction procedure were carried out at 4°C in dark conditions.
Superoxide dismutase (SOD)
SOD (EC 1.15.1.1) activity was assayed by monitoring the inhibition of photochemical reduction of nitroblue tetrazolium according to the method described by Dhindsa et al. (Citation1981) with slight modifications. SOD activity in the supernatant was assayed by its ability to inhibit the photochemical reduction of nitroblue tetrazolium. The reaction mixture contained 0.5 M potassium phosphate buffer (pH 7.5), 0.1 mM EDTA, 13 mM methionine, 63 mM NBT, 1.3 mM riboflavin and enzyme extracts in test tubes, which were incubated under the light of a 15W fluorescent lamp (Philips, India) at 25°C. Identical tubes, which were not illuminated, served as blanks. After illumination for 15 min, absorbance was measured at 560 nm. One unit of SOD activity was defined as the amount of enzyme which inhibited the photoreduction of NBT to blue formazan by 50%, and SOD activity of the extracts was expressed as enzyme units (IU) mg−1 protein min−1.
Catalase (CAT)
CAT (EC 1.11.1.6) activity in the leaves was determined by the method of Aebi (Citation1984). The assay mixture contained 50 mM potassium phosphate buffer (pH 7.0) and 100 µl enzyme extract in a total volume of 3 ml. The reaction was started by adding 10 mM H2O2. CAT activity was determined by monitoring the disappearance of H2O2 by measuring a decrease in absorbance at 240 nm. Activity was calculated by using extinction coefficient 0.036 mM−1 cm−1 and expressed as enzyme units (IU) mg−1 protein min−1. One unit of enzyme determines the amount of CAT necessary to decompose 1 µmol of H2O2 per min. at 25°C.
Glutathione reductase (GR)
GR (EC 1.6.4.2) activity was measured by the method of Foyer and Halliwell (Citation1976) as modified by Rao (Citation1992) based on glutathione-dependent oxidation of NADPH at 340 nm. The assay mixture contained 50 mM potassium phosphate buffer (pH 7.5), 1.0 mM EDTA, 0.2 mM NADPH, and 0.5 mM GSSG. The reaction was started by the addition of enzyme extract and allowed to run for 5 min at 25°C. Corrections were made for any GSSG oxidation in the absence of NADPH. The activity was calculated using extinction coefficient 6.2 mM−1 cm−1 and expressed in enzyme units (IU) mg−1 protein min−1. One unit of enzyme determines the amount necessary to decompose 1 µmol of NADPH per min at 25°C.
Ascorbate peroxidase (APX)
APX (EC 1.11.1.11) activity was determined by the method of Nakano and Asada (Citation1981). The reaction mixture contained 50 mM potassium phosphate buffer (pH 7.5), 0.5 mM ascorbate, 0.1 mM H2O2, 0.1 mM EDTA and enzyme extract. The reaction was started by addition of H2O2. APX activity was measured by the decrease in absorbance at 290 nm within 1 min and calculated by using its extinction coefficient 2.8 mM−1 cm−1 and expressed in enzyme units (IU) mg−1 protein min−1. One unit of enzyme determines the amount necessary to decompose 1 µmol of substrate consumed per min at 25°C. The protein content in enzymatic extracts was determined using Bradford reagent (Bradford Citation1976) using bovine serum albumin as standard.
Statistical analysis
All the experiments were repeated three times. The results were analyzed statistically using SPSS ver. 11 (SPSS Inc., Chicago, USA). The significance of differences among means was carried out using Tukey's test at p<0.05. The results presented are the means±SE of three experiments.
Results
Pigments and photosynthesis
Photoexposed regenerants of T. indica with low (50 µmol m−2 s−1) and high (300 µmol m−2 s−1) PPFD showed considerable increase in photosynthetic pigments (Chl a, b) and carotenoid contents throughout the acclimatization period (A–C). Exposure to varying light intensities stimulated the continuous generation of Chl a and b for the entire period of acclimatization. T. indica has been found to be compatible with low light for the generation of photosynthetic pigments, which is clearly evident with 15% increase over high light exposure at day 28. Nevertheless, the acclimatized plantlets showed a linear increase of 3.3–55% at low and 12–47% at high light for Chl a against day 0 plantlets. Likewise, Chl b also showed the gradient outline of 28–57% at low and 31–36% at high PPFD. With high light exposure, the plantlets showed positive response for increased carotenoid pigments (C). Compared to day 0 plantlets, about 25% increase in carotenoid contents has been observed at high light acclimatized plantlets at day 28. Plantlets acclimatized for two weeks found positive for carotenoid pigments, which further enhanced from 15–27% at low and 35–55% at high light exposure. From the first week of transplantation, the positive measurements of photosynthesis were evaluated (D). The appearance of new leaves induces the net synthetic rate. However, saturation was exhibited by the plantlets acclimatized at low light.
Figure 1. Changes in the level of Chl a content (A), Chl b content (B), carotenoids content (C) and net photosynthetic rate P N (D)in micropropagated plantlets of T. indica acclimatized at PPFD 50 and 300 µmol m−2 s−1 for 28 days. Bars represent the mean±SE (n=5). Bars denoted by the same letter are not significantly different (p =0.05) using Tukey's tests.
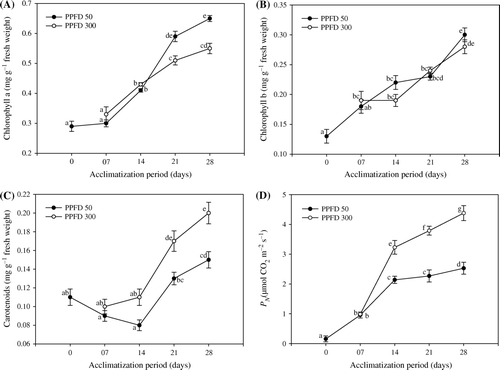
Electrolyte leakage and malondialdehyde concentration
shows the changes in electrolyte leakage and malondialdehyde concentration in leaves of T. indica plantlets. The malondialdehyde concentration and electrolyte was found higher in HL treated plantlets than LL light grown plants. The maximum accumulation of MDA and electrolyte leakage was observed after the first week of acclimatization (A, B).
Figure 2. Changes in the level of Electrolyte leakage (A) and MDA concentration (B) in micropropagated plantlets of T. indica acclimatized at PPFD 50 and 300 µmol m−2 s−1 for 28 days. Bars represent the mean±SE (n=5). Bars denoted by the same letter are not significantly different (p =0.05) using Tukey's tests.
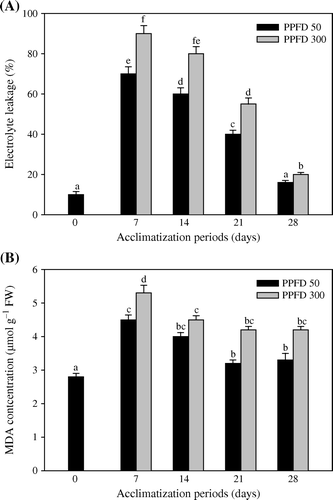
Enzymatic activities
A time-dependent variation in the SOD generation has been noticed in the acclimatized plantlets of T. indica. Increase in the SOD activity has been noticed in plantlets acclimatized at high light intensities (A). Furthermore, the level of catalase increased during the whole period of acclimatization (B). The effect of high PPFD is clearly evident with the elicited catalase activity at the termination day. Likewise, photoexposure of the plantlets with both photosynthetically active radiations (PAR), elevated the level of GR against the day 0 plantlets (A). Nonetheless, the APX activity increased with varying periods of acclimatization (B). At low and high light, day 28 plantlets showed 64 and 72% increased peroxidase activity, respectively, over control.
Figure 3. Activities of superoxide dismutase (A) and catalase (B) in micropropagated plantlets of T. indica acclimatized at PPFD 50 and 300 µmol m−2 s−1 for 28 days. Bars represent the mean±SE (n=5). Bars denoted by the same letter are not significantly different (p =0.05) using Tukey's tests.
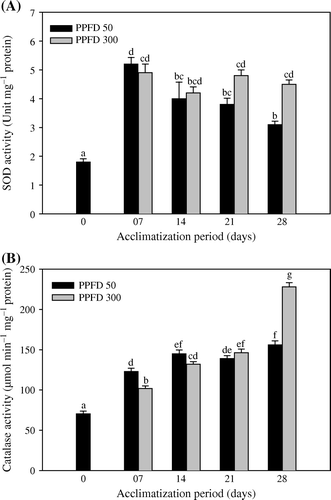
Figure 4. Activities of glutathione reductase (A) and ascorbate peroxidase (B) in micropropagated plantlets of T. indica acclimatized at PPFD 50 and 300 µmol m−2 s−1 for 28 days. Bars represent the mean±SE (n=5). Bars denoted by the same letter are not significantly different (p =0.05) using Tukey's tests.
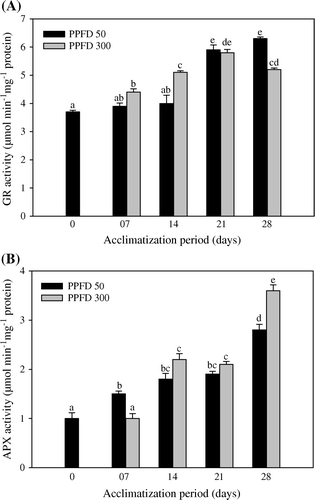
Discussion
In the present study, significant increase in chlorophyll a and b with low light exposure in T. indica plantlets suggested the induction of chlorophyll synthesis enzymes indispensable for chlorophyll biosynthesis. Increase in Chlorophyll a and b content with the formation of new leaves during the acclimatization is in accordance with a report on Rubus (Donnelly and Vidaver Citation1984). The observed variation in chlorophyll content due to high PPFD is an indication of plant under stress. The micropropagated plantlets also exhibited increased level of carotenoids after the first week of acclimatization. An increase in carotenoid level specifies that plants sustained the light stress. Such an increase in carotenoids further reflects the functional response of photosynthetic apparatus to the different light environment, since the photo protective role of carotenoids against photooxidative damage is well documented (Donnelly and Vidaver Citation1984; Young Citation1991; Huylebroeck et al. Citation2000). Plantlets of T. indica exhibited an elevated level of net photosynthetic rate during the first week of acclimatization. A significant increase in net photosynthetic rate was observed with the appearance of new leaves during acclimatization. Similarly, induction in P N activities with the emergence of new leaves was also observed by Huylebroeck et al. (Citation1998) in Calathea lousae and Spathiphyllum floribundum. However, low P N activity in day 0 plantlets is a consequence of in vitro conditions in which the down regulation of photosynthesis occurs due to CO2 depletion in culture vessels.
Plants scavenge ROS through the antioxidant system, employing the enzymes and non-enzymatic components of sub cellular compartments. Such a defense system may be inadequate during over production of ROS (Foyer et al. Citation1994). The generation of ROS could be indicated by an increase in the amount of MDA concentration and electrolytic leakage in the present study, which supports our hypothesis that high light increases the breakdown of lipid membrane. Tolerance to light stress is generally accompanied by an increase in levels of antioxidants, and the activities of antioxidant enzymes (Allen Citation1995), protecting the photosystem from ROS. T. indica demonstrated diverse responses to antioxidant enzymes indicating that oxidative stress is an influential component of light stress. In response, the cellular machinery generates a free radical scavenger (SOD) which effectively prevents membrane oxidation and damage to biological molecules. An increased level of SOD thus suggests a plant protective mechanism against oxidative stress. Micropropagated plantlets also showed an increased catalase activity suggesting it plays a more important role than SOD against ROS generation. Such responses further emphasize the successful acclimatization of plantlets which responded meaningfully to reduce photoinhibition. Increased catalase activity also suggests its role in the photorespiratory detoxification of hydrogen peroxide through the mitochondrial electron transport system (Scandalios Citation1990). The increased level of SOD and catalase in combination would lower the H2O2 levels. In plants, most of the oxygen scavenging activity at high light occurs in chloroplast owing to the presence of ascorbate-glutathione cycle. In photosynthetic tissues, ascorbic acid-dependent antioxidants such as APX and GR are predominantly localized in the chloroplast, which is the major site of H2O2 production in leaves (Foyer et al. Citation1997). However, the activity of antioxidant enzymes in other cell compartments may also be important for ROS scavenging. Increase in antioxidative enzyme activities other than the chloroplast region is indicative of the formation of ROS in mitochondria and peroxisome of different cellular components. The increase in APX activity of T. indica plantlets further suggests chloroplast-based detoxification of ROS via Mahler pathway, as 90% of total leaf APX is located in chloroplast (Gillham and Dodge Citation1986). GR is considered to be a key enzyme responsible for monitoring the reduced form of glutathione pool. During acclimatization, T. indica plantlets showed increased GR activity at high PPFD, which clearly indicates that GR plays an important role in ROS scavenging. Furthermore, the increased GR activity is an indication of maintenance of the pool of glutathione in a reduced state.
It seems that the T. indica plantlets showed different physiological and biochemical behavior in ex vitro conditions. The results of the study suggest that the micropropagated plantlets developed functional photosynthetic machinery along with the auxiliary biochemical pathways to reduce oxidative stress during the acclimatization period.
Acknowledgements
The authors gratefully acknowledge the Department of Science and Technology, Government of India, New Delhi, for the award of a SERC Fast Track Scheme (Project No. SR/FT/L-067/2006) to MF and for providing research support under the DST-FIST (2005) programme.
References
- Aebi , H. 1984 . Catalase in vitro methods . Enzymology , 105 : 121 – 126 .
- Allen , R. 1995 . Dissection of oxidative stress tolerance using transgenic plants . Plant Physiol. , 107 : 1049 – 1054 .
- Arnon , DI. 1949 . Copper enzymes in isolated chloroplasts. Polyphenoloxidase in Beta vulgaris . Plant Physiol. , 24 : 1 – 15 .
- Bradford , MM. 1976 . A rapid and sensitive method for quantitation of microgram quantities of protein utilizing the principle of protein-dye-binding . Annals Biochem. , 72 : 248 – 254 .
- Cakmak , I and Horst , WJ. 1991 . Effect of aluminium on lipid peroxidation, superoxide dismutase, catalase and peroxidase activities in root tips of soybean (Glycine max) . Physiol Plant. , 83 : 463 – 468 .
- Dhindsa , PS , Plumb-Dhindsa , P and Thorpe , TA. 1981 . Leaf senescence: Correlated with increased levels of membrane permeability and lipid peroxidation and decreased levels of superoxide dismutase and catalase . J Exp Bot. , 32 : 93 – 101 .
- Donnelly , DJ and Vidaver , WE. 1984 . Pigment content and gas exchange of red raspberry transferred in vitro and ex vitro . J Am Soc Hort Sci. , 109 : 177 – 181 .
- Faisal , M , Ahmad , N and Anis , M. 2007 . An efficient micropropagation system for Tylophora indica: An endangered, medicinally important plant . Plant Biotech Rep. , 1 : 155 – 161 .
- Foyer , CH and Halliwell , B. 1976 . The presence of glutathione reductase in chloroplast: A proposed role in ascorbic acid metabolism . Planta , 133 : 21 – 25 .
- Foyer , CH , Lelandais , M and Kunert , KJ. 1994 . Photooxidative stress in plants . Physiol Plant. , 92 : 696 – 717 .
- Foyer , CH , Lopez-Delgado , H , Dat , JF and Scott , IM. 1997 . Hydrogen peroxide and glutathione-associated mechanisms of acclamatory stress tolerance and signaling . Physiol Plant. , 100 : 241 – 254 .
- Gillham , DJ and Dodge , AD. 1986 . Hydrogen peroxide scavenging systems in pea chloroplasts . Planta , 167 : 246 – 251 .
- Huylebroeck , JMV , Piqueras , A and Debergh , PC. 1998 . Photosynthesis and carbon metabolism ion leaves formed prior and during acclimatization of micropropagated plants . Plant Sci. , 134 : 21 – 30 .
- Huylebroeck , JMV , Piquerasm , A and Deberghm , PC. 2000 . The evolution of photosynthetic capacity and the antioxidant enzymatic system during acclimatization of micropropagated Calathea plants . Plant Sci. , 155 : 59 – 66 .
- Kozai , T. 1991 . “ Micropropagation under autotrophic conditions ” . In Micropropagation: Technology and application , Edited by: Debergh , PC and Zimmermann , R . 447 – 469 . The Netherlands : Kluwer Academic Publishers .
- Lutts , S , Kinet , J.M and Bouharmont , J. 1995 . Changes in plant response to NaCl during development of rice (Oryza sativa L.) varieties differing in salinity resistance . J Exp Bot. , 46 : 1843 – 1852 .
- Mackinney , G. 1941 . Absorption of light by chlorophyll solution . J Biol Chem. , 140 : 315 – 322 .
- Maclachlan S , Zalik S . 1963 . Plastid structure, chlorophyll concentration and free amino acid composition of chlorophyll mutant barley . Can J Bot . 1053 1062 .
- Munne-Bosch , S and Alegre , L. 2002 . Interplay between ascorbic acid and lipophilic antioxidant defences in chloroplasts of water-stressed Arabidopsis plants . FEBS Lett. , 524 : 145 – 148 .
- Nakano , Y and Asada , K. 1981 . Hydrogen peroxide is scavenged by ascorbate specific peroxidase in spinach chloroplasts . Plant Cell Physiol. , 22 : 867 – 880 .
- Noctor , G and Foyer , CH. 1998 . Ascorbate and glutathione: Keeping active oxygen under control . Ann Rev Plant Physiol. , 49 : 249 – 279 .
- Noctor , G , Gomez , L , Vanacker , H and Foyer , CH. 2002 . Interactions between biosynthesis, compartmentation and transport in the control of glutathione homeostasis and signaling . J Exp Bot. , 53 : 1283 – 1304 .
- Pospisilova , J , Catsky , J and Sestak , Z. 1997 . “ Photosynthesis in plants cultivated in vitro ” . In Handbook of photosynthesis , Edited by: Pessaraki , M . 525 – 540 . New York : Marcel Dekker .
- Rao , MV. 1992 . Cellular detoxifying mechanisms determine age dependent injury in tropical plants exposed to SO2 . J Plant Physiol. , 140 : 733 – 740 .
- Scandalios , G. 1990 . Response of plant antioxidant defense genes to environmental stress . Adv Genet , 28 : 1 – 41 .
- Smirnoff , N and Wheeler , GL. 2000 . Ascorbic acid in plants: Biosynthesis and function . Crit Rev Plant Sci. , 19 : 267 – 290 .
- Young , A.J. 1991 . The protective role of carotenoids in higher plants . Physiol Plant. , 83 : 702 – 708 .