Abstract
We studied the structure of newly found entomogenous galls in three Indian species of Selaginella, and the biochemical changes during gall development as well as their seasonal abundances in 10 squares of 1.5 m2 under two differing habitat conditions, open and covered. Adult wasps (Cynipidae) initiate two types of gall formation by oviposition, spherical galls on vegetative shoots and elongated, club-shaped strobilar galls on reproductive shoots. Galls are anatomically and biochemically different from the unaffected shoots. Vegetative shoot apices bear more galls (84.6%) than reproductive shoot apices (15.4%). Gall frequency is significantly higher in covered (94.8%) than in open habitat (5.2%), and its seasonal peak occurs earlier in the former (43.1% during the rainy season) than in latter habitat (52.8% in autumn). We discuss the relationship between relative species density and gall seasonality as well as the possible role of certain environmental factors that make covered habitats more favorable to the gall inducer.
Abbreviations
lc | = |
larval chamber |
ep | = |
epidermis |
ct | = |
cortex |
st | = |
stele |
nt | = |
nutritive tissue |
cvb | = |
cortical vascular bundle |
n | = |
nucleus |
ne | = |
nuclear envelope |
cw | = |
cell wall |
l | = |
lomasomes |
Introduction
Plant galls are familiar objects of natural history. They are pathologically developed cells, tissues and organs of plants, which mostly develop by hypertrophy and hyperplasy, usually under the influence of a parasitic organism, like viruses, bacteria, fungi, algae, higher plants, nematodes, mites or insects (Mani Citation1964). About 132,930 types of galls are actually known; they are found on algae, fungi, lichens, bryophytes, pteridophytes, gymnosperms and angiosperms (Espírito-Santo and Fernandes Citation2007). Brancroft (Citation1914) reported bud-like tuberous vegetative propagules both at the apices of strobili and vegetative branches of Selaginella chrysocaulos (Hook. and Grev.) Spring, S. lyallii Spring, S. vogelii Spring and S. bulbilifera Baker ex A.Br. from India. However, these propagules seem to be in fact entomogenous galls, which are not frequently found in pteridophytes (Mani Citation1964; Fernandes Citation1987). For example, only one of 35 plant galls collected on Fiji Island is from pteridophytes (Mani and Jayaraman Citation1987). Fossil evidence of various levels of interaction between pteridophytes and arthropods dates back to the Devonian period, and suggests in some cases complex associations (Scott et al. Citation1985). The first and only record of anatomically documented entomogenous galls on fossil ferns was reported from the Upper Carboniferous (Labandeira and Phillips Citation1996). Similarly, very few galls are known to occur in the extant pteridophytes (Strasburger Citation1873; Houard Citation1908; Giesenhagen Citation1909 ,Citation1919; Docters van Leeuwen-Reijnvaan Citation1910 ,Citation1921, Docters van Leeuwen-Reijnvaan and Docters van Leeuwen Citation1926; Docters van Leeuwen Citation1938; Alston Citation1945; Lawton and MacGarvin Citation1985; Kraus et al. Citation1993; Bera et al. Citation1994; Bera and Ghorai Citation1997 ,Citation1999). Alston (Citation1945) reported Cecidomyiid galls on Selaginella pentagona Spring and S. roxburghii (Hook. and Grev.) Spring.
The present paper deals with newly described entomogenous galls in three Indian species of Selaginella – Selaginella monospora Spring, S. bisulcata Spring, and S. chrysorrhizos Spring from Darjeeling Himalaya, India. An unidentified species of the wasp genus Cynips L. (Hymenoptera: Cynipidae) initiates galling in all three species of Selaginella. Some questions have been posed. Do the galls present some structural or biochemical similarity, because they are induced by the same Cynips species? Does gall formation in the strobili affect the reproductive biology of Selaginella? Does seasonal gall abundance differ among species of Selaginella and between covered and open habitats? To answer these questions, we investigated the morphological and anatomical ultrastructure of the galls, biochemical changes during gall development, and ecological aspects as seasonal changes in their abundance under two differing habitat conditions, covered and uncovered (= open) by a thicket of the fern Diplopterygium (Gleicheniaceae).
Materials and methods
Study site
This study was carried out during March–October 2002–2003 in the hilly slopes of Lebong, 5 km southwest of the city of Darjeeling (26°30′05′′, 26°27′10′′ N; 88°53′00′′, 87°59′30′′ E) at elevations between 1200–1850 m a.s.l. Five climatic seasons () can be recognized within this region: winter normally extends from mid-November to mid-February, spring from the end of February to March, summer from April to mid-June, rainy from mid-June to August, and autumn from September to October. Continuous rain, clouds, fog and mist mark the rainy season. The clean sky and sunny days are frequent in autumn. The summer exhibits a cloudy sky throughout the region with variation in sunlight brightness. The other seasons are moderately cloudy while winter is very cool and frequent snowfall occurs.
Table 1. Average (2002–2003) seasonal climatological data of Darjeeling, India. Relative humidity is over 85% and wind velocity over 1.8 km/h throughout the year.
Altitude is the prominent factor in determining five major types of vegetation in Darjeeling Himalaya (Dash Citation1947): tropical and plain vegetation (300–500 m), subtropical (500–1200 m), subtemperate (1200–1850 m), temperate (1850–3200 m) and subalpine vegetation (3200–3800 m). Mixed deciduous forests consisting of important subtropical species such as Schima wallichii Choisy, Phyllanthus emblica L., and Gmelina arborea Roxb. characterize the subtemperate vegetation of the sampling site at lower elevation, while at higher elevation, temperate species, e.g. Leucosceptrum canum Smith, Daphne involucrata Wall. and Edgeworthia gardneri Meissn. are common. Another typical member of this vegetation is the fern Diplopterygium sp., which serves as shade plant for the studied species of Selaginella.
Material collection, gall development and microscopy
Selaginella plants, both with and without galls, were collected and packed in moist closed plastic bags for transport. Their external morphology was analyzed, taking into account the gall form, their size at various developmental stages, and their position on the plant. Leaves on galls and on normal shoots were also studied. To rear the gall insects, mature galls were enclosed by polyethylene bags in situ. Gall development was studied through careful in situ field observations. Shoot apices of Selaginella plants were searched for an egg of the wasp by a powerful magnifying glass and the tagged branches were left for constant observations. The different developmental stages of the galls were studied by splitting of the tagged galls at regular intervals, and the behavior of the cynipid larva was observed under a stereoscopic microscope. For anatomical studies, thin (20 µm) serial transverse sections were made of both the galls and unaffected stems. The sections were gradually passed through different grades of alcohol and stained properly by saffranin and light green, washed in xylol, mounted on glass slides in Canada balsam, and observed under a compound Leitz laborlux microscope. For Scanning Electron Microscopy (SEM), materials were fixed with 2.5% glutaraldehyde in 0.1M sodium phosphate buffer (pH 7.2) for about 16 h at 4°C. After rinsing with the above buffer, the samples were dehydrated in a graded ethanol series and finally put into 3-methyl-butyl-acetate. Samples were critical point dried, mounted on the aluminum stubs using a double adhesive tape and sputter coated with a 35 nm thick film of gold (Trivedi and Bhandari Citation1999). The stubs were investigated under a scanning electron microscope (S-440, Leica Leo, Cambridge, UK) and photographs were taken by the High Resolution Record Unit (HRRU) through a 35 mm automatic camera. Samples of the nutritive tissue of the galls were prepared for observation under a JEOL JEM 100 cx transmission electron microscope following the method of Buschmann et al. (Citation2002).
Methodologies in biochemistry
For a quantitative estimation of different biochemicals of the cynipid galls, these were graded into three developmental stages of the gall-causing insect namely larval, pupal and mature stage. Total sugar, soluble protein, free amino acids, total phenol and chlorophyll a, b and total chlorophyll were estimated following the methods employed by Somogyi (Citation1945); Lowry et al. (Citation1951); Lee and Takahashi (Citation1966); Malik and Singh (Citation1980) and Lichtenthaler and Wellburn (Citation1983), respectively. Healthy plant parts were collected at the same shoot level from the respective species of Selaginella so that they were at comparable developmental stages. Estimations of DNA (deoxyribonucleic acid) and RNA (ribonucleic acid) for the three gall stages of Selaginella monospora were performed following the method of Weislander (Citation1979) and Malik and Singh (Citation1980), respectively, and compared with young, uninfested control shoots.
Sampling design and statistical analyses
For the study of seasonal abundance of cynipid galls, the study area (Darjeeling Himalaya) was divided into two habitats: (a) Area covered by a thicket of Diplopterygium (covered habitat), and (b) an uncovered area (open habitat). Ten squares of 4×4 ft (=1.486 m2) were sampled for each habitat and during each of the four seasons (spring, summer, rainy and autumn). Sampling consisted counting the number of plants with and without galls, the number of vegetative and fertile plants, and the number of vegetative and strobilar galls for each of the three species of Selaginella. Applied statistics are given within the text (F-values for ANOVA, t for t-tests or post-hoc multiple comparisons, and χ2 for Chi-square tests), and were performed in Sigma Stat 3.11.
Results
Cynipid galls (a–d) were noted in three Indian species of Selaginella, namely S. monospora, S. bisulcata and S. chrysorrhizos from Darjeeling, India, within a particular microenvironment, i.e. within and around the undisturbed bushy thickets of Diplopterygium sp. The gall causer is an unidentified wasp species of the genus Cynips (Hymenoptera: Cynipidae)
Figure 1. Selaginella spp. (a) and (b) Galls on vegetative shoot apices of S. monospora and S. bisulcata, respectively. (c) A mature gall on vegetative shoot apex of S. monospora. (d) Strobilar galls of S. monospora.
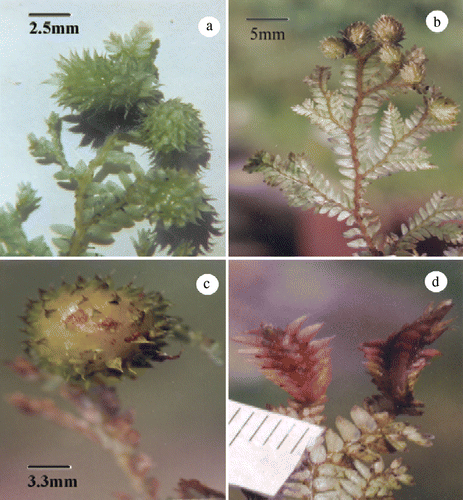
Gall development
The adult wasp causes injury to the vegetative shoot apex or strobilar apex during oviposition that initiates the gall formation. Generally, the wasp deposits only one egg per hole-wound. At the wound spot, a secondary meristem starts growing first around the egg, which is covered entirely by the plant tissue within a period of 24–30 days and 51–65 days in the rainy season and summer, respectively. At this stage, the gall could be recognized only after careful observation, because of its insignificant size (1.5 mm in diameter). In the egg stage, the gall is a compact mass leaving almost no space inside. After hatching, the small white larva of 1.5–2.2 mm length and 0.9 mm width (k) starts feeding on the nutritive gall tissue (c), pupates within the chamber created by its feeding activity, and after attaining maturity, leave the gall through an exit hole, the ostiole.
Figure 2. Selaginella sp. (a) Cross-section of normal stem showing epidermis (ep), cortex (ct) and stele (st) under SEM. (b) and (c) Cross-sections of cynipid gall under Light microscope and SEM, respectively. Note larval chamber (lc), nutritive tissue (nt), cortical vascular bundles (cvb), epidermis (ep). (d) and (e) Details of cortical vascular bundle (cvb) in cynipid gall (SEM). (f) A leaf of vegetative gall showing awns (an) (SEM). (g) Cell wall profile in nutritive tissue of mature gall (TEM). Note thick cell wall (cw) and lomasomes (l). Scale bar = 1 µm. (h) Aggregation of sub cellular materials in pupal gall (TEM). Scale bar = 1 µm. (i) Cross-section of strobilar galls showing larval chamber (lc) without any spore. (j) Hypertrophied and lobed nucleus (n) in nutritive tissue of larval gall (TEM). Scale bar = 1 µm. (k) Gall split open showing cynipid larva.
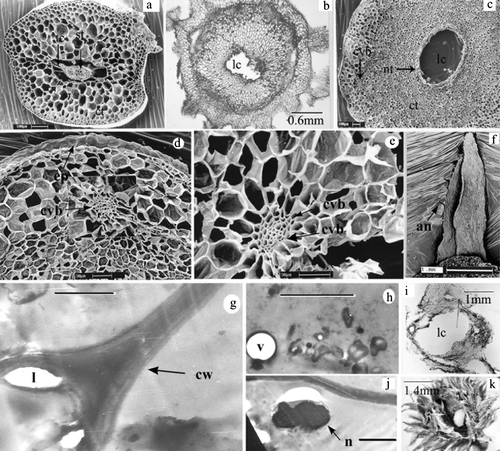
External morphology
Both the vegetative shoot apices and reproductive structures (strobili) of Selaginella spp. may be modified into galls. The vegetative galls and strobilar galls differ morphologically among distinct species of Selaginella (). Vegetative galls are oval-circular (a–c), while strobilar galls are elongated club-shaped structures (d). Each type of gall possesses numerous, closely spaced leaves (a, c, d). The leaves on the gall are uniform in size, relatively broad and shorter than normal leaves and possess very distinctive awns (f). Aging of galls is generally manifested with an increase in size (), hardening and a change in color from green to light brown to deep brown. Finally, the upper part of the gall turns into pink and sometimes into red (c).
Table 2. Dimension of oval-circular, vegetative shoot apex galls and elongated, club-shaped strobilar galls in three different species of Selaginella.
Internal morphology
The internal morphology of a normal shoot of Selaginella spp. differs from the gall in many respects. The former one shows a one cell thick superficial epidermis, a thick parenchymatous cortex without intercellular spaces, and endodermal cells that are modified into radially elongated trabeculae by means of which the steles remain attached to the cortex (a). In contrast, the galls consist of a central larval cavity followed by parenchymatous nutritive tissue, a sclerotic zone, cortex and epidermis (b, c). In the galled stem, the central stelar cylinder of a normal ungalled stem is completely obliterated (b, c), while in the cortical region, many new vascular bundles appear (c, d, e). Transected normal strobili show a central axis with a single stele, surrounded by sporangia, while transected strobilar galls show a single larval cavity without any sporangia (i).
Ultrastructural modifications
Ultrastructural observations of the nutritive zone of cynipid galls of Selaginella monospora at all stages showed cytological specialization with a hypertrophied and lobed nucleus (j), fragmented vacuoles, concentrated cell organelles (h), degenerative cytoplasm (h) and cell wall thickening with lomasomes (g).
Biochemical investigation
The biochemical profile of gall tissue differs from that of the normal ungalled (uninfected) tissue and among the three species of Selaginella ( and ). Total chlorophyll, total sugar and total protein contents of normal and galled tissue differ significantly (F=14.2, 21.0 and 25.1, respectively, all p<0.05) at all gall stages in each of the three species. The content of chlorophyll a, chlorophyll b, total chlorophyll, and total soluble protein is lower in galled tissue than in uninfected tissue and decreased with the subsequent stages of the gall ( and ). In contrast, total sugar, free amino acids and total phenol content increased in successive stages of the gall (). However, in ungalled tissue, total sugar content decreased after reaching its maximum in the pupal stage, free amino acid content increased from the larval to the mature stage, while total phenol content decreased from larval to mature stage (). As a result of these tendencies, mature gall tissue had a higher sugar and total phenol content than uninfested tissue at the same stage. Concentrations of DNA and RNA were significantly lower in the ungalled tissue than in the cynipid galls (t=13.5 and t=77.5, respectively, both p <0.05), especially at the larval and pupal stage ().
Figure 3. Chlorophyll a and b content (mg/g dry weight) of normal (white columns) and galled (gray columns) tissues at three developmental stages of three species of Selaginella. Mean±SD, n=5.
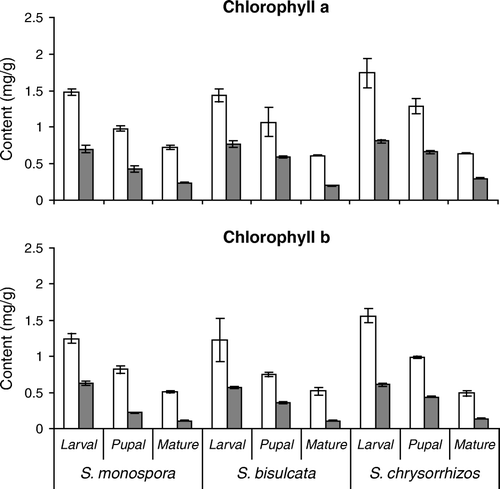
Figure 4. Total sugar, protein, free amino acid and phenol content (mg/g dry weight) of normal (white columns) and galled (gray columns) tissues at three developmental stages of three species of Selaginella. Mean±SD, n=5.
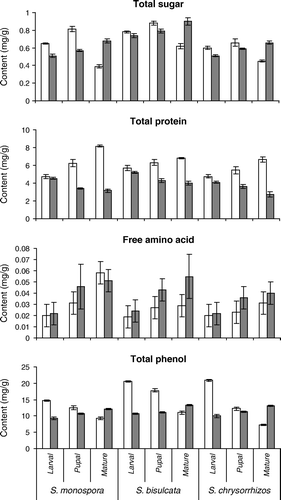
Table 3. Estimation of nucleic acid (DNA, RNA) of ungalled and galled tissue of Selaginella spp. Means in µg/g fresh weight±SE, n=3. Different letters indicate significant differences (p<0.05).
The biochemical profile varies among species. S. chrysorrhizos had significantly higher chlorophyll content than S. monospora and S. bisulcata in normal tissue at the age of larval and pupal stage of gall development (F=6.2 and 12.3, respectively, p<0.05) and in the galled tissue at pupal and mature stage of development (F=562.5 and 221.7, p<0.001; ). Sugar and protein content were higher in S. bisulcata than in S. monospora and S. chrysorrhizos in the larval stage of normal tissue (F=215.8 and 27.1, respectively, p<0.001, ). In normal tissue at the larval gall stage, phenol content was significantly lower in S. monospora than in the other two species (F=1043.2, p<0.001, ), while the concentration of amino acids did not vary among species.
Gall abundance and seasonality
The occurrence of cynipid galls within and around the Diplopterygium thickets at Darjeeling Himalaya varies considerably among habitats, species and seasons ( and ). In covered habitat, 63.3% of all plants were infested (74.8% of S. monospora, 66.0% of S. bisulcata, and 12.1% of S. chrysorrhizos) while in open habitat only 6.1% were gall-bearing (7.8% of S. monospora, 5.8% of S. bisulcata, and 3.1% of S. chrysorrhizos). The proportions of gall-bearing and gall-free plants differed significantly among species (χ2=27.4, p<0.001), and for each of the species between the two habitats (S. monospora, χ2=150.0, S. bisulcata, χ2=97.3, and S. chrysorrhizos, χ2=18.8, all p<0.001). S. monospora bore most of the galls in both habitats (63.3% in covered habitat and 63.8% in open habitat), followed by S. bisulcata (34.4%, and 27.8%), while only 2.3% of all galls in the covered habitat and 8.3% in the open habitat were found on S. chrysorrhizos. In the covered habitat, frequency of gall-bearing plants (and total gall number) was seasonal for all three species (all F>160.7, p<0.001), with significantly higher values in the rainy season than the rest of the year (Holm-Sidak multiple comparison, all t>3.01, p<0.05). In the open habitat, with about nine times fewer galled plants, seasonality of gall density shifted to a significant peak in autumn (all t>4.2, p<0.05). Vegetative galls in both habitats accounted for 86.4% of all galls in S. monospora, and 85.7% of S. bisulcata, while in S. chrysorrhizos, no strobilar galls were observed. However, the proportion of strobilar galls between both habitats did not differ from the proportion of vegetative galls (all χ2<1.33, p<0.05).
Figure 5. Seasonal density of gall-free (white) and gall-bearing (gray) plants of three species of Selaginella in dependence on habitat type at Lebong, Darjeeling Himalaya, India (2002–2003), Mean±SD, n=10.
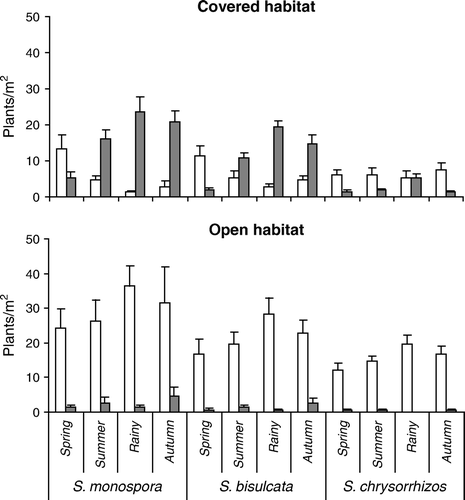
Figure 6. Seasonal density of vegetative galls (white) and strobilar galls (gray) of three species of Selaginella in dependence on habitat type at Lebong, Darjeeling Himalaya, India (2002–2003), Mean±SD, n=10. The scale for open habitat is ten times smaller than for covered habitat. S. chrysorrhizos had no strobilar galls.
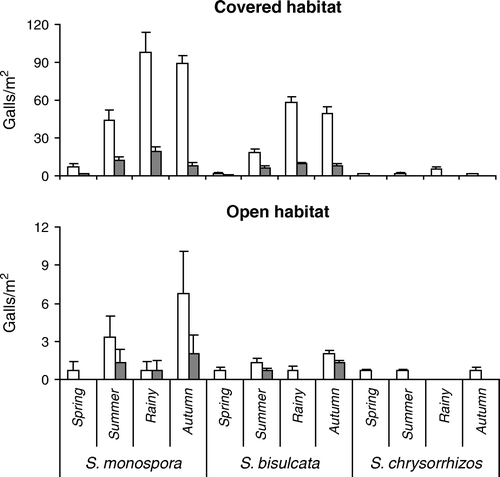
Discussion
A gall is essentially the product of a mutual interaction of a plant and a gall-inducing agent. In the present study, oviposition by the adult wasp on the shoot apex followed by feeding injury of its larva possibly stimulates the plant tissue to develop the galls. Newly formed, cortical vascular bundles in the cynipid galls of Selaginella functionally replace the central vascular bundles (stele) of the normal stem of Selaginella that have been obliterated in the cynipid gall. The nutritive tissue around the larval chamber and cortical vascular bundles are considered to be developed due to the influence of the gall insect on the host plant.
In no case did the galled strobili of Selaginella contain spores. This phenomenon may be due to the consumption of the early sporogenous tissues by the cynipid larva, which is not definitely known but in any case galls will have an important bearing on the reproductive biology of Selaginella spp.
The abundance of cynipid galls was higher in covered habitat than in open habitat at Darjeeling. This discrepancy in gall occurrence may be due to the rain, which hinders ovipositon and survival of the eggs in the open habitat. Besides, the bushy thickets of Diplopterygium provide a warm-humid and undisturbed microhabitat to the cynipid wasps for their safe feeding niche, increased survival and greater level of infestation. Interspecific variations in seasonal frequency of cynipid galls among the three Indian species of Selaginella were mainly due to their different relative abundance () in the study area, and not a consequence of differences in their biochemical profiles.
The relative decrease of assimilatory pigments during the gall development might be the consequence of an increasing production of gall tissue with less pigment in which chloroplasts are usually restricted to external cortical layers, and of the negative impact of the feeding cynipid larvae on chlorophyll synthesis leading to a lower photosynthetic efficiency in gall systems. The latter might be either due to higher chlorophyllase activity or due to the decreased chlorophyll synthesis (Chandraguru et al. Citation1987). The present TEM studies have shown depletion of chloroplasts and cellular materials in different stages of gall development. Similar observations have been recorded earlier in Mimusops galls (Raman and Ananthakrishnan Citation1983).
The concentration of total sugar is higher in the normal (uninfected) young shoot of Selaginella than that in the galled tissue (). Such difference can be explained by the fact that a cynipid gall cannot synthesize starch when the larva is alive because it produces amylase that inhibits the synthetic activity of phosphorylase (Raman Citation1993). When the larva stops feeding, the concentration of total sugar increases gradually from pupal to mature stage of gall development. Raman and Ananthakrishnan (Citation1983) showed a similar kind of sugar accumulation in the later stages of the ontogeny of thrips induced leaf galls.
Phenol content of the healthy tissue was higher than in the larval stage of the gall of Selaginella spp. Cynipid larvae are capable of feeding phenol-rich tissue because of enzymes present in their saliva leading to a lower phenol content in the galls (Raman Citation1993). Once the cynipid larvae stop feeding, phenol concentration of galls increases again (Feeny Citation1969). The increased accumulation of phenols in pupal and mature stages of cynipid galls may be due to the enhanced synthesis by the host plant through Shikimic acid pathway or the action of peroxidase on phenolics (Chandraguru et al. Citation1987), and may possibly reduce the nutritional availability of sugar and soluble protein (Janzen Citation1977). A cynipid gall is an autolytic system (Bronner Citation1992), and cynipid larvae secrete protease enzyme that hydrolyze the protein, explaining the observed sharp decrease of protein content in the larval and subsequent gall stages of all three species of Selaginella, and the increase in free amino acid content (Molliard Citation1913). At the larval stage free, amino acids are utilized by the larvae and with subsequent development of the gall, they remain accumulated as unutilized product. Nutritive gall tissue has a higher concentration of nuclear and ribosomal RNA and soluble amino products (Raman and Ananthakrishnan Citation1983). High concentration of DNA and RNA in the larval and pupal stages of the cynipid gall is known to be due to intense protein synthesis (Bronner Citation1977), and its decrease in the mature stage of the gall is a consequence of the decline of protein synthesis. From the present study, it is clear that the vegetative propagules reported by Brancroft (Citation1914) on several species Indian Selaginella were, in fact, entomogenous galls.
The structure of a gall depends primarily on the organ on which it is formed, the plant species, and the gall inducing organism. Although all galls in this study were caused by the same gall wasp and shared some morphological similarities, gall shape differed between vegetative and strobilar shoots, and among plant species. Gall formation in strobilar shoots negatively affected the reproductive biology by annulling any spore production. Gall frequencies differed among species, habitats and seasons, and were significantly higher in covered than in open habitat, and most abundant during the rainy season. Gall biochemistry varied significantly among species, indicating a different sensitivity of the three species of Selaginella to the impact of the gall wasp. More extensive and profound studies of pteridophyte galls would be enlightening, since their relative anatomical simplicity in comparison to angiosperm galls facilitates the investigations and could provide a model system for a better understanding of the intricate interactions between insects and plants.
Acknowledgements
We thank Professor Radhanath Mukhopadhyay, Department of Botany, Burdwan University, for identifying the different species of Selaginella, and Dr Narayan Ghorai, Department of Zoology, Presidency College, Kolkata, for identifying the gall-causing insect.
References
- Alston , AGH. 1945 . An enumeration of the Indian species of Selaginella . Proc Nat Inst Sci India. , 11 ( 3 ) : 211 – 235 .
- Bera , S and Ghorai , N. 1997 . On the occurrence of mite induced stem gall in Odontosoria chinensis (L) J. Smith (Dennstaedtiaceae) from Darjeeling, West Bengal . Indian Fern J. , 14 : 103 – 105 .
- Bera , S and Ghorai , N. 1999 . On the occurrence of mite gall caused by Trioza fletcheri (Eriophidae) on Angiopteris evecta Hoff. (Angiopteridaceae) from Andaman Islands . Indian Fern J. , 16 : 15 – 18 .
- Bera , S , Ghorai , N and Raut , SK. 1994 . Cynipid gall in Selaginella monospora Spring . Indian Fern J. , 11 ( 12 ) : 146 – 149 .
- Brancroft , N. 1914 . Notes on vegetative reproduction in some Indian Selaginellas . Ann Bot. , 28 ( 112 ) : 685 – 693 .
- Bronner , R. 1977 . Contributions a l’étude histoquimique des tissus nourriciers des zoocecidies . Marcellia. , 40 : 1 – 134 .
- Bronner , R. 1992 . Biology of insect induced galls. The role of nutritive cells in the nutrition of cynipids and cecidomyiids , 119 – 140 . New York : Oxford University Press .
- Buschmann , H , Potter , U and Beeching , J. 2002 . Ultrastructure of Cassava root studied by TEM and SEM . Microscopy Analysis. , 25 : 9 – 11 .
- Chandraguru , T , Ananthakrishnan , TN and Gopinathan , T. 1987 . Age correlated biochemical changes in two thrips-induced galls . Curr Sci. , 56 : 283 – 287 .
- Dash AJ. 1947 . Bengal District Gazetteers, Darjeeling . Government of West Bengal, India .
- Docters van Leeuwen-Reijnvaan , WJ. 1910 . Über die Entwicklung einiger Milbengallen . Ann Jard Bot Buitenzorg. , 23 : 142
- Docters van Leeuwen-Reijnvaan , WJ. 1921 . ber die von Eriophyes pauropus Nal. an verschiedenen Arten von Nephrolepis gebildeten Blattgallen . Ann Jard Bot Buitenzorg , 31 : 85
- Docters van Leeuwen-Reijnvaan J , Docters van Leeuwen WM. 1926 . The Zoocecidia of the Netherlands East Indies . Batavia .
- Docters van Leeuwen , WM. 1938 . Manual of Pteridology , 192 – 195 . The Hague : Martinus Nijhoff. Zoocecidia .
- Espírito-Santo , MM and Fernandes , GW. 2007 . How many species of gall-inducing insects are there on earth, and where are they? . Ann Entomol Soc Amer. , 100 : 95 – 99 .
- Feeny , PP. 1969 . Inhibitory effect of oak leaf tannins on larval growth and the hydrolysis of proteins by trypsin . Phytochemistry , 8 : 2119 – 2126 .
- Fernandes , GW. 1987 . Gall forming insects: Their economic importance and control . Revta Bras Entomol. , 31 : 379 – 398 .
- Giesenhagen , K. 1909 . Über zwei Tiergallen an Farnen . Ber Deutschen Bot Ges. , 22 : 327
- Giesenhagen , K. 1919 . Entwicklung der Milbengalle an Nephrolepis biserrata Schott . Jahrb f wiss Bot. , 63 : 66
- Houard C. 1908 . Les Zoocecidies des plantes d'Europe et du Bassin de la Méditerranée , Paris . 1 .
- Janzen DH. 1977 . Why are there so many species of insects? Proc 15th Int Cong Ent, Washington 84 96 .
- Kraus , JE , Montenegro , G and Kim , AJ. 1993 . Morphological studies on entomogenous stem galls of Microgramma squamulosa (Kaulf.) Sota Polypodiaceae . Amer Fern J. , 83 : 120 – 128 .
- Labandeira , CC and Phillips , TL. 1996 . A Late Carboniferous petiole gall and the origin of homometabolous insects . Proc Natl Acad Sci USA. , 93 : 8470 – 8474 .
- Lawton , JH and MacGarvin , M. 1985 . Interaction between bracken and its insect herbivores . Proc Roy Soc Edinburgh. , 86B : 125 – 131 .
- Lee , YP and Takahashi , T. 1966 . An improved colorimetric determination of amino acids with the use of ninhydrin . Ann Biochem. , 14 : 71
- Lichtenthaler , HK and Wellburn , AR. 1983 . Determinations of total carotenoids and chlorophylls a, and b of leaf extracts in different solvents . Biochem Soc Trans. , 60 : 531 – 550 .
- Lowry , OH , Rosebrough , NJ , Farr , A and Randall , RJ. 1951 . Protein measurement with folin phenol reagent . J Biol Chem. , 103 : 256 – 275 .
- Malik , CP and Singh , MB. 1980 . Plant enzymology and histoenzymology , 286 New Delhi : Kalyani Publishers .
- Mani , MS. 1964 . Ecology of plant galls , 2 – 56 . The Hague, , Netherlands : Dr. Junk Publishers .
- Mani , MS and Jayaraman , P. 1987 . On some plant galls from the Fiji Islands . Proc Indian Acad Sci (Anim Sci) , 96 ( 2 ) : 81 – 115 .
- Molliard , M. 1913 . Recherches physiologiques sur les galles . Rev Gener Bot. , 25 : 225 – 252 .
- Raman A. 1993 . Chemical ecology of phytophagous insects . Chapter 15. Chemical Ecology of Gall insect-host plant interactions: Substances that influence the nutrition and resistance of insect and the growth of galls . New Delhi : Oxford and IBH Publishing Company Pvt Ltd 229 243 .
- Raman , A and Ananthakrishnan , TN. 1983 . Studies on some thrips (Thysanoptera: Insecta) induced galls. 2. Fine structure of the nutritive zone . Proc Indian Nat Sci Acad. , 49 : 525 – 561 .
- Scott , AC , Chaloner , WG and Paterson , S. 1985 . Pteridophyte-arthropod interactions in the fossil record . Proc Royal Soc Edinburgh. , 86B : 113 – 140 .
- Somogyi , MJ. 1945 . A new reagent for determination of sugar . J Biol Chem. , 160 : 161
- Strasburger , E. 1873 . Einige Bemerkungen über Lycopodiaceae. Die Bulbillen und Pseudobulbillen der Selaginella . Bot Ztg. , 31 : 105
- Trivedi , A and Bhandari , NN. 1999 . SEM Studies on the ontogeny of asexual reproductive bodies of Thamnostylum piriforme (Brain.) Von Arx and Upadhyay . Phytomorphology , 49 ( 3 ) : 325 – 331 .
- Weislander , LA. 1979 . A simple method to recover intact high molecular weight RNA and DNA after electrophoretic separation in low gelling temperature agarose gels . Ann Biochem. , 98 : 305