Abstract
The efficacy of intercropping of the medicinal plant zimmu (Allium sativum L.×Allium cepa L.) and an antagonistic bacterium Burkholderia sp. strain TNAU-1 was evaluated separately and in combination for control of Aspergillus flavus infection and aflatoxin B1 contamination in groundnut (Arachis hypogaea L.) under field conditions. The Burkholderia sp. strain TNAU-1 inhibited the growth of all the toxigenic and non-toxigenic isolates of A. flavus in vitro and the inhibition zone ranged from 3.1–11.3 mm. A talc-based powder formulation of the Burkholderia sp was developed for field application. When groundnut seeds were treated with the formulation of Burkholderia sp., significant increases in root length, shoot length and seedling vigor were observed. Zimmu intercropping (once in every three rows of groundnut) alone significantly reduced the A. flavus infection and aflatoxin contamination in groundnut. Seed treatment at 10 g/kg or soil application at 2.5 kg/ha on 30, 45, 60 days after sowing with the formulation of Burkholderia sp. also resulted in significant reduction in the infection by A. flavus and aflatoxin B1 contamination in kernels. The maximum percentage reduction in aflatoxin B1 content was achieved by the combination of zimmu intercropping, seed treatment and soil application of Burkholderia sp. Seed treatment followed by soil application with the formulation of Burkholderia sp. recorded the highest pod yield in both the field trials. Zimmu intercropping or seed treatment or soil application with formulation of Burkholderia sp. significantly reduced the population of A. flavus in the soil. Application of Burkholderia sp. through seed or soil resulted in high colonization of Burkholderia sp. in rhizosphere soil, and the rhizosphere population increased with increase in the age of the crop. A colony PCR assay was developed using primers made to Burkholderia sp. specific sequence of the ribosomal DNA for accurate detection and confirmation of Burkholderia sp.
Introduction
Aflatoxins are toxic secondary metabolites produced by certain strains of Aspergillus flavus Link ex Fries and Aspergillus parasiticus Speare (Woloshuk and Prieto Citation1998). These compounds are known to be potent animal hepatocarcinogens and are suspected to be carcinogens of humans (Williams et al. Citation2004). Currently, 18 different types of aflatoxins have been identified, with aflatoxin B1, B2, G1, G2, M1, and M2 being the most common. Of these, Aflatoxin B1 (AFB1) and G1 (AFG1) occur most frequently, with AFB1 being the most potent (Mishra and Das Citation2003). Dietary intake of aflatoxin leads to the disease aflatoxicosis and the risk posed by aflatoxin depends on the level and type of aflatoxin in the diet, the strain of animal and its nutritional status (Williams et al. Citation2004). Due to concern for the potential effects of aflatoxins on human health even a low level of contamination is important and hence most countries have extensive monitoring programmes and legislation that restrict marketing of aflatoxin-contaminated grains and products (Van Egmond Citation1989). Most countries have established maximum tolerance levels for total aflatoxin ranging from 4–20 µg/kg. The US Food and Drug Administration has set an aflatoxin limit of 20 ppb for foods and for most feeds and feed ingredients. The European Union has enacted a very stringent aflatoxin tolerance threshold of 2 µg/kg aflatoxin B1 and 4 µg/kg total aflatoxins for nuts and cereals for human consumption (Bankole and Adebanjo Citation2003).
Groundnut (Arachis hypogaea L.) with its geocarpic nature of fruit development is highly vulnerable to invasion by aflatoxigenic fungi and subsequent contamination by aflatoxins. The fungi invade groundnut seeds at pre-harvest stage, during post-harvest curing and drying and in storage (Goncalez et al. Citation2008). Pre-harvest aflatoxin contamination is a major problem in the tropics, which limits marketability and causes economic losses. Drought at the end of the cropping season facilitates aflatoxin contamination of groundnut (Wilson and Stansell Citation1983). Infection of groundnut by root-knot nematodes (Meloidogyne arenaria) can lead to an increase in aflatoxin kernels when the plants are subjected to drought stress during pod maturation (Timper et al. Citation2007).
A great deal of effort has been devoted to developing methods for reducing aflatoxin contamination in groundnut. A few A. flavus-resistant genotypes have been developed but the level of resistance in them was not sufficient to protect the kernels from aflatoxin contamination under all conditions (Waliyar et al. Citation1994; Waliyar et al. Citation2008; Nigam et al. Citation2009). The proliferation of A. flavus that occurs in groundnut kernels during storage is predominantly as a result of infection that occurred in the field (Dorner and Cole Citation2002). Hence pre-harvest elimination of aflatoxin is considered to be the most rational and economic approach to control aflatoxin in groundnut (Dorner Citation2008). Cultural practices, such as adjustments of sowing and harvesting dates, application of gypsum, maintenance of optimum water relations in the crop through irrigation, control of pod- and seed-feeding insects, optimum timing of digging and harvest, avoidance of mechanical damage during cultivation, harvest and post-harvest handling, and rapid post-harvest drying and maintenance of low temperature and relative humidity (RH) in storage can be effective to a certain extent in preventing aflatoxin contamination in groundnut (Xue et al. Citation2003). Although some of the fungicides are found effective in preventing the growth of A. flavus (Latif et al. Citation1985; Badii and Moss Citation1988; Brenneman et al. Citation1993), chemical control is neither practical nor economical. Sandosskumar et al. (Citation2007) demonstrated that intercropping of groundnut with the medicinal plant zimmu (Allium sativum L.×Allium cepa L.) is capable of significantly reducing the population of A. flavus in soil and subsequent aflatoxin contamination in groundnut kernels. Several biocontrol agents have been reported to control aflatoxin in groundnut (Cotty Citation1990; Dorner et al. Citation2003; Dorner Citation2004). This approach is based on the use of non-toxigenic strains of A. flavus and/or A. parasiticus to compete with the naturally occurring toxin-producing strains in soil (Dorner et al. Citation1992; Chourasia and Sinha Citation1994; Dorner Citation2004; Dorner and Lamb Citation2006). However, adequate precautions must be taken while selecting the non-toxigenic strains for biological control. The selected strains must be incapable of producing toxins, and competitive under field conditions. Dorner et al. (Citation1992) demonstrated that application of different non-aflatoxigenic strains of A. parasiticus to soil reduced pre-harvest aflatoxin contamination when peanuts were exposed to late-season drought and temperature stress. However, one of those strains (NRRL 18991) was subsequently shown to produce o-methylsterigmatocystin (OMST), the immediate biosynthetic precursor to aflatoxin B1 (Brown et al. Citation1999). An ultraviolet (UV)-induced mutant of the strain NRRL 18786, which was effective in reducing aflatoxin contamination (Dorner et al. Citation1992), was found to produce versicolorin A. Tran-Dinh et al. (Citation1999) suggested that, if genetic exchange can occur, the introduction of a more aggressive non-toxigenic isolate as a biocontrol agent could result in a recombinant with both increased aggressiveness and toxigenicity. Hence, plant growth-/soil health-promoting native antagonistic microorganisms offer great potential as alternatives to chemical pesticides, which are frequently associated with environmental pollution. Vijayasamundeeswari et al. (Citation2007) isolated a bacterial strain from corn kernels that inhibit the growth of A. flavus and promote plant growth. The antagonistic bacterial strain was identified as Burkholderia sp. based on phenotypic and biochemical characteristics and 16S rRNA gene sequence analysis. In the present study, a talc-based powder formulation of Burkholderia sp. was developed and its efficacy in the control of A. flavus infection and aflatoxin contamination in groundnut either alone or in combination with zimmu intercropping was demonstrated under field conditions.
Materials and methods
Fungal culture
The fungus, A. flavus, was isolated from infected groundnut (Arachis hypogaea L.) kernels and maintained on potato dextrose agar (PDA) (Difco Laboratories, Detroit, MI, USA) medium under laboratory conditions. The Aspergillus cultures were identified up to species level using a taxonomic key and species descriptions (Singh et al. Citation1991). The isolates were analyzed for their aflatoxin B1 production potential in potato dextrose broth following the method of Karthikeyan et al. (Citation2009).
Antagonist
Burkholderia sp. strain TNAU-1, which is known to inhibit the growth of Aspergillus flavus and other phytopathogenic fungi in vitro (Vijayasamundeeswari et al. Citation2007) was obtained from the culture collection of the Department of Plant Pathology, Tamil Nadu Agricultural University, Coimbatore, India. The antagonistic bacterium was Gram-negative, aerobic, straight rod, further characterized by positive reactions in motility, gelatin hydrolysis, catalase reaction, oxidase reaction, and utilization of galactose, glucose, inositol and sorbitol, and negative reactions in production of fluorescent pigments and starch hydrolysis. It was found to belong to the genus Burkholderia on the basis of phenotypic characteristics and phylogenetic analysis of 16S rRNA gene sequences using CLUSTAL-W multiple sequence alignment program (Thompson et al. Citation1994). The antagonist Burkholderia sp. strain TNAU-1 was compatible with other beneficial rhizobacteria including Bacillus megaterium var. phosphaticum strain PBS, Rhizobium strain TNAU14, Glucanoacetobacter diazotrophicus, Azospirillum brasiliense strain 204, Azospirillum lipoferum strain SP7. The antagonist was cultured in Petri plates on PDA medium under laboratory conditions.
In vitro screening of Burkholderia sp. strain TNAU-1 against A. flavus
In vitro inhibition of mycelial growth of A. flavus was assayed by dual culture technique. Burkholderia sp. strain TNAU-1 was streaked on a Petri plate (1 cm from the edge) containing PDA medium. Simultaneously, an 8-mm-diameter mycelial disc cut from a seven-day-old culture of A. flavus was placed on the center of the Petri plate. PDA medium inoculated with the fungus alone served as control. The plates were incubated at room temperature (28±2°C) for seven days. At the end of incubation period, the zone of inhibition (mm) was recorded by measuring the distance between the edges of the fungal mycelium and the antagonistic bacterium. Three replications were carried out for each treatment. The experiment was repeated once with three replications for each treatment.
Development of talc-based powder formulation of Burkholderia sp. strain TNAU-1
All manipulations were carried out under sterile conditions. Talc-based powder formulation of Burkholderia sp. strain TNAU-1 was developed as described by Vidhyasekaran and Muthamilan (Citation1995). Ten grams of carboxymethylcellulose was mixed with 1 kg of talc (hydrated magnesium silicate) and the pH was adjusted to 7.0 by adding calcium carbonate. The mixture was then autoclaved at 121°C for 30 min for two consecutive days. Burkholderia sp. strain TNAU-1 was grown in potato dextrose broth for 48 h at 28±2°C on a rotary shaker. Four hundred milliliters of bacterial suspension containing 9×108 CFU ml-1 was added to 1 kg of the talc mixture and thoroughly mixed. The formulation was packed with 35% moisture content in polythene bags, sealed and stored at room temperature (28±2°C). The survival of Burkholderia sp. in the prepared formulation was evaluated at different time intervals by dilution plating.
Seedling vigor test
The plant growth promoting activity of Burkholderia sp. strain TNAU-1 on groundnut was tested by using the roll towel method (International Seed Testing Association Citation1996). The seeds were treated with the formulation at 10 g/kg and incubated for 6 h at room temperature. The seeds were then placed on coarse blotter paper sheets and covered with a moistened blotter and rolled. The roll was kept on a butter paper sheet and rolled as a bundle and incubated in a growth chamber at 25°C with 80% RH. After 15 d of incubation, percent of germination was recorded and root length and shoot length were measured. Vigor index was calculated by multiplying percent plant stand with sum of shoot and root length.
Field trials
Two field trials were conducted during July–October, 2007, one at the Agricultural Research Station, Bhavanisagar, Tamil Nadu, India, and another trial at the Agricultural Research Station, Vridhachalam, Tamil Nadu, India. The susceptible groundnut cultivars, CO-3 (Bunch type; duration 115–120 days) and VRI-2 (Bunch type; duration 100–105 days) obtained from the Department of Oilseeds, Tamil Nadu Agricultural University, Coimbatore, Tamil Nadu, were used in the field trials. The trials were laid out in a randomized block design with seven treatments and three replications with a plot size of 5×4 m and with a spacing of 30×15 cm. The treatments comprised as follows:
-
Zimmu intercropping (once in every three rows of groundnut, one week prior to sowing of groundnut with a plant to plant spacing of 45 cm);
-
Seed treatment with talc-based formulation of Burkholderia sp. strain TNAU-1 at the rate of 10 g/kg;
-
Soil application of talc-based formulation of Burkholderia sp. strain TNAU-1 at the rate of 2.5 kg/ha on 30, 45, 60 days after sowing (DAS);
-
Seed treatment (10 g/kg) followed by soil application (2.5 kg/ha) with talc-based formulation of Burkholderia sp. on 30, 45, 60 DAS;
-
Zimmu intercropping + seed treatment (10 g/kg) + soil application (2.5 kg/ha) with talc-based formulation of Burkholderia sp. on 30, 45, 60 DAS;
-
Seed treatment with carbendazim at the rate of 2 g/kg;
-
Untreated control.
The population of A. flavus and Burkholderia sp. in rhizosphere soil, kernel infection by A. flavus and aflatoxin B1 content in groundnut kernels were determined as described below. Pod yield and other growth parameters were also recorded. Oil content in groundnut kernels was estimated using Oxford 4000 NMR analyzer (Oxford Instruments, UK).
Assessment of A. flavus and Burkholderia sp. population in rhizosphere soil
The initial populations of A. flavus as well as population of A. flavus and Burkholderia sp. after 60 and 110 days (prior to harvest) after sowing were determined by dilution plate technique. Soil samples were collected with the help of an auger at 5 cm soil depth (Nahdi Citation1996). Four grams of soil sample were added to 100 ml of sterile distilled water in a 250 ml conical flask and shaken for 10 min on a rotary shaker at 150 rpm. From this suspension, a series of dilutions were made. One ml of the soil suspension was mixed with 19 ml of sterilized PDA medium and poured in to a sterile 9-mm-diameter Petri dish. The plates were incubated at room temperature (28±2°C) for five to seven days and A. flavus or Burkholderia sp. colonies that developed were counted.
Detection of Burkholderia sp. by colony PCR
Burkholderia sp. specific forward primer (5′ CGAACGGGTGAGTAATAC 3′) and reverse primer (5′ GCTGGCACGTAGTTAGC3′) for PCR assays were designed based on the nucleotide sequence (GenBank accession number EU560426) and synthesized by Operon Company (Operon Biotechnologies, Cologne, Germany) in salt-free status. PCR was under taken in 20 a µl volume consisting of 5 mM each dNTPs, 20 pmol of each primers, 0.5 U of Taq DNA polymerase and single loop of bacterial colony. The reaction was carried out in a Palm-Cycler Model CGI-960 (Corbett Research, Mortlake, NSW, Australia) programmed with initial denaturation at 94°C for 3 min, followed by 35 cycles of 94°C for 1 min, 59°C for 1 min, 72°C for 1 min, and a final extension step at 72°C for 10 min. Following amplification, 10 µl of each PCR product was electrophoresed on 2% agarose gel in TAE buffer. The DNA fragments in the gel were stained with ethidium bromide, visualized under ultraviolet light, and recorded with an AlphaImager 2000 (Alpha Innotech, San Leandro, CA).
Assessment of kernel infection by A. flavus
Plants were harvested from each plot and pods were removed and dried in the shade for three to four days. After drying, pods were hand-shelled and 100 seeds were selected randomly and tested in the laboratory to determine the percentage of infection by A. flavus. Seeds were surface sterilized by soaking in 0.1% aqueous solution of mercuric chloride for 3 min, rinsed with sterile distilled water and placed on filter paper in 10 cm-diameter sterile Petri dishes and incubated at 25°C. To maintain high humidity 1–2 ml of sterile distilled water was added everyday during the first five days. After six days of incubation, the number of seeds contaminated by A. flavus was counted (Waliyar et al. Citation1994).
Analysis of aflatoxin B1 content in groundnut
Pod samples of approximately 1 kg were drawn from each plot and the pods were thoroughly mixed. From each 1 kg sample, three 100 g sub-samples were drawn and shelled. Aflatoxin B1 content in the samples was determined by indirect competitive ELISA following the method of Reddy et al. (Citation2001).
Statistical analysis
The data were statistically analyzed by Duncan's Multiple Range Test (DMRT). The package used for analysis was IRRI STAT version 92-1 developed by the International Rice Research Institute Biometrics Unit, Philippines.
Results
The Burkholderia sp. strain TNAU-1 inhibited the growth of all the toxigenic and non-toxigenic isolates of A. flavus in dual culture on PDA medium and the inhibition zone ranged from 3.1 mm (AFG47) to 11.3 mm (AFG1) (). A talc-based powder formulation of the Burkholderia sp. strain TNAU-1 was developed for field application.
Table 1 In vitro antagonistic activity of Burkholderia sp. strain TNAU-1 against aflatoxigenic and non-aflatoxigenic isolates of Aspergillus flavus.
The survival of Burkholderia sp. strain TNAU-1 in the prepared formulation was evaluated by dilution plating. The bacterial strain TNAU-1 survived well in the talc-based powder formulation during up to one month of storage under room temperature. The formulation had a bacterial load of 15.9×108 CFU/g at the time of preparation (). The number of living bacteria in the talc formulation was 8.8×108 CFU/g after one month of storage at room temperature. During the first 30 days after preparation the population of Burkholderia sp. strain TNAU-1 in the formulation dropped approximately 45%.
Table 2. Population of Burkholderia sp. strain TNAU-1 in the talc-based powder formulation.
The plant growth promoting activity of Burkholderia sp. strain TNAU-1 on groundnut was tested by using the roll towel method. When groundnut seeds were treated with the formulation of Burkholderia sp. strain TNAU-1, significant increases in root length, shoot length and seedling vigor were observed ().
Table 3. Effect of seed bacterization with the formulation of Burkholderia sp. strain TNAU-1 on seed germination and seedling vigor of groundnut (cv. CO-3).
Kernel infection by A. flavus
The effect of seed treatment or soil application with Burkholderia sp. strain TNAU-1 or zimmu intercropping in controlling A. flavus infection in groundnut is shown in and . The maximum kernel infection (65%) by A. flavus was recorded when untreated groundnut seeds were sown in the A. flavus infested soil. Zimmu intercropping alone significantly reduced the A. flavus infection in groundnut. In the treatment of zimmu intercropping, colonization of groundnut kernels by A. flavus was not observed in Trial I, and 2% infection was observed in Trial II. Seed treatment or soil application with Burkholderia sp. strain TNAU-1 also resulted in significant reduction in A. flavus infection over control.
Table 4. Effect of seed treatment or soil application with the formulation of Burkholderia sp. strain TNAU-1 or intercropping with zimmu on Aspergillus flavus infection, aflatoxin B1 concentration, oil content and pod yield of groundnut (cv. VRI-2) under field conditions (Trial I).
Table 5. Effect of seed treatment or soil application with the formulation of Burkholderia sp. strain TNAU-1 or intercropping with zimmu on Aspergillus flavus infection, aflatoxin B1 concentration, oil content and pod yield of groundnut (cv. CO-3) under field conditions (Trial II).
Aflatoxin contamination
The maximum AFB1 content of 71.4 and 92.4 g/kg in Trial I and Trial II, respectively, was recorded when untreated groundnut seeds were sown in the A. flavus infested soil ( and ). All the treatments showed significant reduction in AFB1 content in groundnut over control. Zimmu intercropping alone significantly reduced the AFB1 content (97% reduction) in groundnut compared to untreated control in both the trials. Seed treatment with Burkholderia sp. strain TNAU–1 resulted in 95% reduction in aflatoxin contamination over control in both the trials. Soil application with Burkholderia sp. strain TNAU-1 powder formulation also exhibited significant reduction (90–92% reduction) in AFB1 content in groundnut kernels over control. AFB1 content was significantly reduced in the treatment of zimmu intercropping + seed treatment + soil application of Burkholderia sp. strain TNAU-1 by 99% in Trial I and 97% in Trial II.
Pod yield
Control plots recorded pod yields of 1255 kg/ha and 2975 kg/ha in Trial I and Trial II, respectively ( and ). Seed treatment followed by soil application with the powder formulation of Burkholderia sp. strain TNAU-1 recorded the highest pod yield of 1821 kg/ha and 3223 kg/ha in Trial I and Trial II, respectively. The pod yield was less in zimmu intercropped plots compared to that in control in Trial II, probably because of reduction in population size of groundnut plants. No significant differences in oil content and 100 seed weight were observed between the treatments ( and ).
Population of A. flavus in rhizosphere soil
Treatment of groundnut with Burkholderia sp. strain TNAU-1 resulted in significant reduction in the population density of A. flavus in rhizosphere soil. At the time of sowing, untreated control soil contained A. flavus population of 2×103 and 1.1×103 in Trial I and Trial II, respectively ( and ). Zimmu intercropping or seed treatment or soil application or seed treatment followed by soil application with Burkholderia sp. strain TNAU-1 completely suppressed the population of A. flavus 60 DAS and at the time of harvest (110 DAS).
Table 6. Population of A. flavus and Burkholderia sp. in rhizosphere soils sampled at different time intervals after treatment (Trial I).
Table 7. Population of A. flavus and Burkholderia sp. in rhizosphere soils sampled at different time intervals after treatment (Trial II).
Population of Burkholderia sp. in rhizosphere soil
Application of Burkholderia sp. strain TNAU-1 through seed or soil resulted in high colonization of Burkholderia sp. in rhizosphere soil ( and ). A colony PCR assay was developed for accurate detection and confirmation of Burkholderia sp. Primers made to Burkholderia sp. specific sequence of the ribosomal DNA were used to amplify the internal transcribed Spacer (ITS) regions from randomly picked bacterial colonies. The Burkholderia sp. specific primers resulted in the amplification of a 417-bp fragment from all the Burkholderia sp. strain TNAU-1 colonies in colony PCR (). There was no cross-reactivity with purified genomic DNA from any other bacterial isolates that were tested: Xanthomonas oryzae pv. oryzae, Pseudomonas fluorescens, Bacillus subtilis and Azospirillum brasiliense. The high sensitivity of PCR enables quick and accurate detection of Burkholderia sp. in soil.
Figure 1. Detection of Burkholderia sp. by polymerase chain reaction. Lane 1, DNA ladder; Lanes 2–7, Burkholderia sp. strain TNAU-1 colonies from the experimental field; Lane 8, Xanthomonas oryzae pv. oryzae; Lane 9, Pseudomonas fluorescens; Lane 10, Bacillus subtilis; Lane 11, Azospirillum brasiliense; Lane 12, Purified DNA from Burkholderia sp. strain TNAU-1.
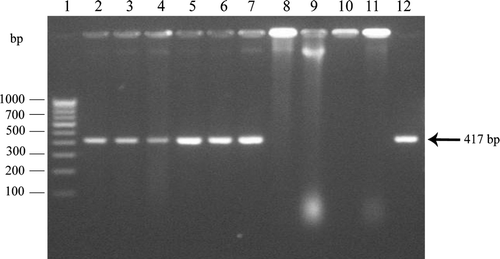
Discussion
The process of infection of peanut kernels by A. flavus and subsequent production of aflatoxin is quite complex and different from other soil-borne diseases (Thakur et al. Citation2003). The level of A. flavus population in soil is important in relation to infection of groundnut pods and seeds. These levels vary greatly from one region to the other (Griffin Citation1970) and are influenced by soil type and crop rotation (Joffee and Lisker Citation1970; Nahdi Citation1996). Nahdi (Citation1996) reported that populations of A. flavus were significantly higher in the pod-zone than in the field soil and increased with maturation of the crop, and significantly high populations of A. flavus were recorded at 0–5 cm depth than at 5–10 cm. The results of the present study indicated that zimmu intercropping alone significantly reduced A. flavus infection and aflatoxin contamination in groundnut kernels. There was a sharp decline in the population of A. flavus in the rhizosphere soil collected from groundnut plants intercropped with zimmu. The root exudates released from zimmu in the rhizosphere might have resulted in inhibition of development of A. flavus population and subsequent afaltoxin contamination (Sandosskumar et al. Citation2007). Such allelopathic effects of root exudates of plants have also been reported by other authors (Michel et al. Citation1996; Yu Citation1999; Agrawal and Tripathi Citation2003). Michel et al. (Citation1996) demonstrated that planting cowpea between tomato reduced the bacterial wilt in tomato in Taiwan. Adhikari and Bansyat (Citation1998) observed significant reduction in bacterial wilt in intercropping/rotation with maize, okra, cowpea and resistant line of tomato (CL1131) in Nepal. Agrawal and Tripathi (Citation2003) reported that the population of Fusarium udum decreased in plots of pigeonpea intercropped with sorghum, and the reduction in wilt incidence in pigeonpea was attributed to fungitoxic exudates secreted by sorghum roots. Yu (Citation1999) demonstrated that intercropping tomato with Chinese Chive (Allium tuberosum) plants significantly reduced the population of Pseudomonas solanacearum and suppressed the occurrence of bacterial wilt of tomato.
Significant reduction in the population of A. flavus in soil was also recorded in groundnut when the formulation of Burkholderia sp. strain TNAU-1 was applied through seed or soil. Species belonging to the genus Burkholderia have been widely used as biocontrol agents against many phytopathogenic fungi, such as Pythium aphanidermatum, Pythium ultimum, Fusarium sp., Phytophthora capsici, Botrytis cinerea and Rhizoctonia solani (Hebbar et al. Citation1998; Heydari and Misaghi Citation1998; Cain et al. Citation2000; Li et al. Citation2002). Watanabe et al. (Citation2000) reported that Burkholderia sp. isolate 87-11 obtained from basidiospore of Lentinus lepideus showed antagonistic activity against Pythium aphanidermatum and Rhizoctonia solani. In the present study, seed treatment or soil application with Burkholderia sp. strain TNAU-1 completely suppressed the population of A. flavus in rhizosphere soil 60 DAS and at the time of harvest. The maximum percentage reduction in A. flavus and AFB1 content in groundnut kernels was achieved by the combination of zimmu intercropping, seed treatment and soil application of Burkholderia sp. strain TNAU-1. The reduction in A. flavus infection and subsequent aflatoxin production in groundnut by application of Burkholderia sp. strain TNAU-1 may be attributable to direct antagonistic effect of Burkholderia sp. strain TNAU-1 on A. flavus. PCR technique has been widely used to detect and monitor a variety of microorganisms in the environment (Steffan and Atlas Citation1991). In the present study, a colony PCR assay was developed for accurate detection and confirmation of Burkholderia sp. The Burkholderia sp. specific primers resulted in the amplification of a 417-bp fragment from all the Burkholderia sp. colonies from the experimental plot in colony PCR. DNA from other bacterial species tested did not produce amplification products with the Burkholderia sp. specific primers. The DNA primers used in the present study are highly specific for detection of Burkholderia sp.
The results of the present study also indicated that seed treatment followed by soil application with the powder formulation of Burkholderia sp. strain TNAU-1 recorded the highest pod yield. Several strains of plant growth-promoting rhizobacteria (PGPR) are known to stimulate plant growth by secreting auxins, gibberellins and cytokinins (Dubeikovsky et al. Citation1993) and by suppression of deleterious microorganisms (Gamliel and Katan Citation1993). Several authors reported increased crop yield due to application of Burkholderia sp. even in the absence of the pathogen (Hebbar et al. Citation1998; Heydari and Misaghi Citation1998; Li et al. Citation2002). The increase in groundnut pod yield due to application of Burkholderia sp. strain TNAU-1 in the present study may be due to plant growth promoting characteristics of Burkholderia sp. strain TNAU-1. The pod yield was less in the zimmu intercropped plots compared to that in control in Trial II, probably because of reduction in population size of groundnut plants.
Due to the increasingly popular trends towards environment friendly ‘organic farming’ the necessity of finding acceptable ‘natural’ effective alternatives to ‘artificial’ chemical pesticides against plant pathogens is of great interest. Biological control of aflatoxin contamination is a promising component of the integrated management of aflatoxin contamination in groundnut. The present study clearly indicates the usefulness of a talc-based powder formulation of Burkholderia sp. strain TNAU-1 for control of A. flavus infection and aflatoxin contamination in groundnut. The results of the present study also showed that intercropping with zimmu alone is capable of significantly reducing the population of A. flavus in soil and subsequent infection and aflatoxin production in groundnut kernels. The biocontrol agent Burkholderia sp. strain TNAU-1 could be used in combination with intercropping with zimmu to provide greater protection of groundnut to kernel infection by A. flavus and aflatoxin contamination. Since zimmu is much used for its medicinal importance and also in certain culinary preparations in India, its use as intercrop may fetch additional income for the farmers.
Acknowledgements
This study was supported by the Indian Council of Agricultural Research, New Delhi.
References
- Adhikari , TB and Bansyat , RC. 1998 . Effect of crop rotation and cultivar resistance on bacterial wilt of tomato in Nepal . Can J Plant Pathol. , 20 : 283 – 287 .
- Agrawal , SC and Tripathi , AK. 2003 . Efficacy of different intercropping systems on wilt and Phytophthora blight incidence and yield of pigeonpea . Ind J Pulses Res. , 16 : 71 – 72 .
- Badii , F and Moss , MG. 1988 . The effect of the fungicides tridemorph, fenpropimorph and fenarimol on growth and aflatoxin production by Aspergillus parasiticus Speare . Lett Appl Microbiol. , 7 : 37 – 39 .
- Bankole , SA and Adebanjo , A. 2003 . Mycotoxins in food in West Africa: Current situation and possibilities of controlling it . Afr J Biotechnol. , 2 : 254 – 263 .
- Brenneman , TB , Wilson , DM and Beaver , RW. 1993 . Effects of Diniconazole on Aspergillus population and aflatoxin production in peanut under irrigated and non irrigated conditions . Plant Dis. , 77 : 608 – 612 .
- Brown , MP , Brown-Jenco , CS and Payne , GA. 1999 . Genetic and molecular analysis of aflatoxin biosynthesis . Fungal Genet Biol. , 26 : 81 – 98 .
- Cain , CC , Henry , AT , Waldo , RH , Casida , LJ and Falkinham , JO. 2000 . Identification and characteristics of a novel Burkholderia strain with broad-spectrum antimicrobial activity . Appl Environ Microbiol. , 66 : 4139 – 4141 .
- Chourasia , HK and Sinha , RK. 1994 . Potential of the biological control of aflatoxin contamination in developing peanut (Arachis hypogaea L.) by atoxigenic strains of Aspergillus flavus . J Food Sci Technol. , 31 : 362 – 366 .
- Cotty , PJ. 1990 . Effect of atoxigenic strains of Aspergillus flavus on aflatoxin contamination of developing cotton seed . Plant Dis. , 74 : 233 – 235** .
- Dorner , JW. 2004 . Biological control of aflatoxin contamination of crops . J Toxicol. , 23 : 425 – 450 .
- Dorner , JW. 2008 . Management and prevention of mycotoxins in peanuts . Food Addit Contam. , 25 : 203 – 208 .
- Dorner , JW and Cole , RJ. 2002 . Effect of application of non-toxigenic strains of Aspergillus flavus and A. parasiticus on subsequent aflatoxin contamination of peanuts on storage . J Stored Prod Res. , 38 : 329 – 339 .
- Dorner , JW , Cole , RJ and Blankenship , PD. 1992 . Use of a biocompetitive agent to control preharvest aflatoxin in drought stressed peanuts . J Food Prot. , 55 : 888 – 892 .
- Dorner , JW , Cole , RJ , Connick , WJ , Daigle , DJ , McGuire , MR and Shasha , BS. 2003 . Evaluation of biological control formulations to reduce aflatoxin contamination in peanuts . Biol Control. , 26 : 318 – 324 .
- Dorner , JW and Lamb , MC. 2006 . Development and commercial use of afla-guard, an aflatoxin biocontrol agent . Mycotoxin Res. , 21 : 33 – 38 .
- Dubeikovsky , AN , Mordukhova , EA , Kochethov , VV , Polikarpova , FV and Boronin , AM. 1993 . Growth promotion of black currant soft wood cuttings by recombinant strain P. fluorescens BSP53a synthesizing an increased amount of indole-3-acetic acid . Soil Biol Biochem. , 25 : 1277 – 1281 .
- Gamliel , A and Katan , J. 1993 . Suppression of major and minor pathogens by fluorescent pseudomonads in solarised and non-solarised soil . Phytopathology. , 83 : 68 – 75 .
- Goncalez , E , Nogueira , JH , Fonseca , H , Felicio , JD , Pino , FA and Correa , B. 2008 . Mycobiota and mycotoxins in Brazilian peanut kernels from sowing to harvest . Int J Food Microbiol. , 123 : 184 – 190 .
- Griffin , GJ. 1970 . Conidial germination and population of Aspergillus flavus in the geocarposphere of peanut . Phytopathology. , 60 : 1387 – 1391 .
- Hebbar , KP , Martel , MH and Heulin , T. 1998 . Suppression of pre- and post-emergence damping-off in corn by Burkholderia cepacia . Eur J Plant Pathol. , 104 : 29 – 36 .
- Heydari , A and Misaghi , IJ. 1998 . Biocontrol activity of Burkholderia cepacia against Rhizoctonia solani in herbicide-treated soils . Plant Soil. , 202 : 109 – 116 .
- International Seed Testing Association . 1996 . International Rules for Seed Testing . Seed Sci Technol Supp. 24 .
- Joffee AZ , Lisker KD 1970 . Effect of crop sequence and soil types on the mycoflora . Plant Soil . 32 : 393 406 .
- Karthikeyan , M , Sandosskumar , R , Mathiyazhagan , S , Mohankumar , M , Valluvaparidasan , V , Sangit , Kumar and Velazhahan , R. 2009 . Genetic variability and aflatoxigenic potential of Aspergillus flavus isolates from maize . Arch Phytopath Plant Protect. , 42 : 83 – 91 .
- Latif , N , Msihriky , N , Haggag , B , Elshayeb , N and Mabrouk , S. 1985 . Inhibition of aflatoxin production in Aspergillus flavus by pyrazolines and pyrazoles . Pest Sci. , 16 : 147 – 151 .
- Li , W , Roberts , DP , Dery , PD , Meyer , SLF , Lohrke , S , Lumsden , RD and Hebbar , KP. 2002 . Broad spectrum antibiotic activity and disease suppression by the potential biocontrol agent Burkholderia ambifaria BC-F . Crop Prot. , 21 : 129 – 135 .
- Michel , VV , Hartman , GL and Midmore , DJ. 1996 . Effects of previous crop on soil populations of Burkholderia solanacearum, bacterial wilt, and yield of tomatoes in Taiwan . Plant Dis. , 80 : 1367 – 1372 .
- Mishra , HN and Das , C. 2003 . A review on biological control and metabolism of aflatoxin . Crit Rev Food Sci Nut. , 43 : 245 – 264 .
- Nahdi , S. 1996 . Populations of Aspergillus flavus in field and pod-zone soil in groundnut fields . Indian Phytopath. , 49 : 57 – 61 .
- Nigam , SN , Waliyar , F , Aruna , R , Reddy , SV , Kumar , PL , Craufurd , PQ , Diallo , AT , Ntare , BR and Upadhyaya , HD. 2009 . Breeding peanut for resistance to aflatoxin contamination at ICRISAT . Peanut Sci. , 36 : 42 – 49 .
- Reddy , SV , Kiran Mayi , D , Uma Reddy , U , Thirumala-Devi , K and Reddy , DVR. 2001 . Aflatoxins B1 in different grades of chillies (Capsicum annum L.) in India as determined by indirect competitive-ELISA . Food Addit Contam. , 18 : 553 – 558 .
- Sandosskumar , R , Karthikeyan , M , Mathiyazhagan , S , Mohankumar , M , Chandrasekar , G and Velazhahan , R. 2007 . Inhibition of Aspergillus flavus growth and detoxification of aflatoxin B1 by the medicinal plant zimmu (Allium sativum L.×Allium cepa L.) . World J Microbiol Biotechnol. , 23 : 1007 – 1014 .
- Singh K , Frisval JC , Thrane U , Mathur SM . 1991 . An illustrated manual on identification of some seedborne Aspergilli, Fusaria, Penicillia and their mycotoxins . Danish Government Institute of Seed Pathology for Developing Countries, Hellerup, Denmark 133 .
- Steffan , RJ and Atlas , RM. 1991 . Polymerase chain reaction: Amplifications in environmental microbiology . Ann Rev Microbiol. , 45 : 137 – 161 .
- Thakur , RP , Rao , VP and Subramanyam , K. 2003 . Influence of biocontrol agents on population density of Aspergillus flavus and kernel infection in groundnut . Indian Phytopath , 56 : 408 – 412 .
- Thompson , HJ , Wilson , A , Lu , J , Singh , M , Jiang , C , Upadhyyaya , P and El-Bayoumy , K. 1994 . Comparison of the effects of an organic and an inorganic form of selenium on a mammary carcinoma cell line . Carcinogenesis. , 15 : 183 – 186 .
- Timper , P , Holbrook , C and Wilson , D. 2007 . Root vs. pod infection by root-knot nematodes on aflatoxin contamination of peanut . Commun Agric Appl Biol Sci. , 72 : 655 – 658 .
- Tran-Dinh , N , Pitt , JI and Carter , DA. 1999 . Molecular genotype analysis of natural toxigenic and non-toxigenic isolates of Aspergillus flavus and A. parasiticus . Mycol Res. , 103 : 1485 – 1490 .
- Van Egmond , HP. 1989 . Current situation on the regulations for mycotoxins. Overview of tolerances and status of the standard methods of sampling and analysis . Food Addit Contam. , 6 : 139 – 188 .
- Vidhyasekaran , P and Muthamilan , M. 1995 . Development of formulations of Pseudomonas fluorescens for control of chickpea wilt . Plant Dis. , 79 : 782 – 786 .
- Vijayasamundeeswari A , Vijayanandraj S , Mohankumar M , Paranidharan V , Velazhahan R . 2007 . Biological control of Aspergillus flavus infection and aflatoxin contamination in groundnut by using antagonistic Burkholderia gladioli . In: Proceedings of National Seminar on Molecular Plant Pathology and Biotechnology for Sustainable Crop Production. Department of Studies in Applied Botany, Seed Pathology and Biotechnology, University of Mysore, Mysore, India 52 .
- Waliyar , F , Ba , A , Hamma , H , Bonkoungou , S and Bosc , JP. 1994 . Sources of resistance to Aspergillus flavus and aflatoxin contamination in groundnut genotypes in West Africa . Plant Dis. , 78 : 704 – 708 .
- Waliyar , F , Kumar , PL , Traore , A , Ntare , BR , Diarra , B and Kodio , O. 2008 . “ Pre- and post-harvest management of aflatoxin contamination in peanuts ” . In Mycotoxins: Detection methods, management, public health and agricultural trade , Edited by: Leslie , JF , Bandyopadhyay , R and Visconti , A . 209 – 218 . Oxfordshire : CAB International .
- Watanabe , T , Tsukamoto , T and Shirata , A. 2000 . Potential of antagonistic isolate obtained from Lentinus lepideus basidiospores as a biocontrol agent . Mycoscience. , 41 : 79 – 82 .
- Williams , J , Phillips , TD , Jolly , PE , Stiles , JK , Jolly , CM and Aggarwal , D. 2004 . Human aflatoxicosis in developing countries: A review of toxicology, exposure, potential health consequences, and interventions . Am J Clin Nut. , 80 : 1106 – 1122 .
- Wilson , DM and Stansell , JR. 1983 . Effect of irrigation on aflatoxin contamination of peanuts pods . Peanut Sci. , 10 : 54 – 56 .
- Woloshuk , CP and Prieto , R. 1998 . Genetic organization and function of the aflatoxin B1 biosynthetic genes . FEMS Microbiol Lett. , 160 : 169 – 176 .
- Xue , HQ , Isleib , TG , Payne , GA , Wilson , RF , Novitzky , WP and O'Brian , G. 2003 . Comparison of aflatoxin production in normal- and high-oleic backcross-derived peanut lines . Plant Dis. , 87 : 1360 – 1365 .
- Yu , JQ. 1999 . Allelopathic suppression of Pseudomonas solanacearum infection of tomato (Lycopersicum esculentum) in a tomato-Chinese chive (Allium tuberosum) intercropping system . J Chem Ecol. , 25 : 2409 – 2417 .