Abstract
The effect of Azospirillum brasilense Sp245 on the micropropagation of three fruit rootstocks: Mr.S 2/5 plum (Prunus cerasifera×P. spinosa), GF 677 hybrid (Prunus persica×P. amigdalus), and MM 106 apple (Northen Spy×M1) was assessed. Rooted shoots were treated with 3×107 of Sp245 cells during transplantation from in vitro cultures to the acclimatization phase. After 60 days, growth parameters were positively affected by Sp245 inoculum. In the case of Mr.S 2/5, an increase in rootstock stem length and node number by 37% and 42%, respectively, compared to the control was noted. In the case of GF 677, the bacterial inoculum increased stem length and node number by up to the 75% and 65%, respectively, compared to the control. The inoculum did not exert on MM 106 for both parameters suggesting that the effects of Sp245 could depend on a specific clone-microbe association. In all cases, however, a higher vigor, consistent with a wider leaf area, was present in the inoculated plantlets demonstrating that the use of Azospirillum can significantly contribute to optimize plant performance during the phase of adaptation of plants to post-vitrum conditions.
Introduction
Micropropagation is a sophisticated technique for the rapid multiplication of plants; its great commercial potential is due to the rate of propagation, the elevated plant quality standards and the ability to produce disease-free plants (Ahmed et al. Citation2001). Moreover, it is also considered an efficient method of propagating large numbers of genetically uniform plants (Honda and Kobayashi Citation2004). On the other hand, micropropagation is not recommended for certain plant species due to serious problems concerning specific steps including explant sterilization and media manipulation that are adopted to induce shoot proliferation and rooting. The acclimatization phase of micropropagated rooted plantlets is a crucial step that can often invalidate the success of micropropagation, making the plantlet production a cost-intensive process. Although several studies have been performed in order to improve the in vitro propagation technique, difficulties are still being encountered in achieving satisfactory plant acclimatization. Microplants maintained in vitro under aseptic conditions are susceptible to biotic and abiotic stresses when transferred to a post-vitro environment (Vestberg et al. Citation2004). As a result, significant losses can occur at acclimatization due to the vulnerable physiological status of microplants (Grunewaldt-Stoecker Citation1997). For instance, the aseptic environment can frequently induce microplants to develop very thin cuticles and non-functional stomata, bringing about desiccation of plants during the acclimatization phase (Preece and Sutter Citation1991). In these conditions, even a weak saprophyte can become dangerously pathogenic for these plants causing damping-off and death (Williamson et al. Citation1997). Particularly, fresh-rooted plants show frail and inefficient roots, stomata with hampered closure mechanisms, a lower cuticle production and may consequently lead to plant dehydration followed by death. The management of the environmental conditions prior to or during the acclimatization phase makes it possible to reduce these losses, gradually adapting plants to the external environmental factors (Van Huylenbroeck and Debergh Citation1996). So far during industrial scale-up, a possible solution to reduce deleterious effects due to all these numerous variables is based on the use of fertilizers and fungicides that improve plant growth and protection but mean increased costs for the producer.
An efficient control of post-vitro plant production should be obtained through a combination of high quality plantlets and good cultivation practices. During the last decade several studies performed on the application to plants of root-colonizing microorganisms have made it possible for a significant increase of the adaptive capacity of micropropagated plants in post-transplant conditions. Such microorganisms may be widely used to influence plant yield and quality due to their ability to induce plant resistance to different environmental stresses. Rhizobacteria and mycorrhizal fungi have been found to increase the performance of plants under stress conditions (Bethlenfalvay et al. Citation1997; Lazarovits and Nowak Citation1997). Induced resistance responses to microbial inoculants are well documented in plants grown in vivo (Benhamou et al. Citation1996; Hunt and Ryals Citation1996; Kloepper et al. Citation1997). Moreover, induction of stress resistance in plant propagules produced in vitro prior to transplanting is the primary target of several research groups attempting utilization of microbial inoculants in micropropagation (Hooker et al. Citation1995; Nowak et al. Citation1995; Balla et al. Citation1997; Lazarovits and Nowak Citation1997; Murphy et al. Citation1997; Wilhelm et al. Citation1997).
Biotization is a metabolic response of in vitro-grown plant material to a microbial inoculant(s), leading to the developmental and physiological changes enhancing biotic and abiotic stress resistance of the derived propagules (Nowak Citation1998). In vitro bacterization of potato plantlets has been shown to enhance their transplant stress tolerance thereby eliminating the need for an expensive greenhouse hardening step, which even now is commonly used by pre-elite seed potato producers. Plants bacterized in vitro with P. fluorescens strains CHA0 and IP10 were found to have a significantly higher fresh shoot weight compared to non-bacterized plants in the same system (Duffy et al. Citation1999). Arbuscular mycorrhizal fungi (AMF) have also been shown to reduce drought stress (Nelson Citation1987) and increase disease resistance (Dehne Citation1982).
Free-living root bacteria exerting beneficial effects on plant growth are known as plant growth promoting rhizobacteria (PGPR). Several Azospirillum brasilense strains have PGPR activity through the production of phytohormones, effects on plant cell membranes, nitrogen acquisition, and phosphate solubilization among other mechanisms (Bashan et al. Citation2004). Azospirillum, a non-specific associative nitrogen-fixing rhizobacteria, has been extensively used as inoculum for crop phytostimulation entailing nitrogen fixation and phytohormone production, particularly auxin-related compounds such as indole-3-acetic acid (IAA) (Steenhoudt and Vanderleyden Citation2000; Dobbelaere et al. Citation2001). A. brasilense has been applied as an inoculant to improve the efficiency of crop cultivation of a wide variety of crops in different growth and climatic conditions (Okon and Labandera-Gonzales Citation1994). An improvement in the ability of water resource management in plants under osmotic stress was evident in wheat seedlings inoculated with A. brasiliense Sp245 (Creus et al. Citation1998). In a study by Carletti (Citation2002), PGPR strains (Azospirillum spp., Azotobacter spp., Bacillus spp., Bradyrhizobium spp., Pseudomonas spp.) were selected following their application to different plant species of agronomic interest (barley, jojoba, tomato, pepper and periwinkle), under different growth conditions (hydroponic, in vitro culture, acclimatization phase) and inoculation methods (bacterial culture, supernatant or suspended cells). The most evident response was obtained by immersion of the basal part of shoots in the bacterial inoculant during an induction period of 24 h. Acclimatization of micropropagated plants inoculated with PGPR (particularly with A. brasilense) showed that such bacteria improve root development with a greater root surface area and root mass production (Carletti Citation2002). Therefore, the large scale application of this plant growth promoting bacteria to crops as an inoculant is attractive as it could lead to a substantial reduction of use of chemical fertilizers and fungicides, which are an important source of environmental pollution (Dobbelaere et al. Citation2003).
This work aims at evaluating the effectiveness of a method based on the application of A. brasilense cells to micropropagated plantlets at the time of trasplanting from in vitro culture to acclimatization conditions. In particular, the study focuses on the assessment of the effect of the bacterial inoculum on three different fruit tree rootstocks by evaluating plant growth and plant health at the end of post-vitrum acclimatization both in growth-chamber and greenhouse trials, respectively.
Materials and methods
Bacterial strain and cultivation
Stock cultures of A. brasilense Sp245 wild-type strain (Baldani et al. Citation1986) stored at −80°C in 20% glycerol, were grown overnight at 30°C and 120 rpm in Nutrient broth medium (Oxoid) supplemented with Ampicillin (100 µg l−1) at 30°C for 24 h. Cells were harvested by centrifugation (10 min at 4,300 g, at 4°C), washed in sterile water and resuspended in 0.9% NaCl solution and adjusted to 107 CFU ml−1 at OD600 confirmed by plating as reported by Michiels et al. (Citation1991), prior being applied as the inoculum.
Micropropagated plantlets
In vitro-derived shoots (0.5–0.7 cm in length) of Mr. S2/5 clone (Prunus cerasifera×P. spinosa) were propagated under controlled in vitro conditions on MS proliferation medium (Murashige and Skoog Citation1962) supplemented with 1 mg l−1 thiamine, 100 mg l−1 myoinositol, 30 g l−1 sucrose, 0.6 mg l−1 6-benzylaminopurine (BA), 0.2 mg l−1 giberellic acid (GA3), 0.06 mg l−1 indolebutyric acid (IBA), pH 5.3, and 6 g l−1 agar (Fluka). Explants were then moved to an elongation MS medium containing 0.2 mg l−1 BA and 200 mg l−1 activated charcoal prior to be transferred to rooting medium enriched with 0.6 mg l−1 IBA.
In vitro-derived shoots (0.5–0.7 cm in length) of GF 677 (Prunus persica×P. amygdalus) were propagated in vitro on DKW proliferation medium (Driver and Kuniyuki Citation1984) supplemented with 1 mg l−1 thiamine, 100 mg l−1 myoinositol, 30 g l−1 sucrose, 2.0 mg l−1 BA, pH 5.3, 6 g l−1 agar (Fluka) and 4 g l−1 Carrogenin (Frimulsionm UF Cisalpina Food, Bergamo, Italy). Shoots were then elongated for 10 days on culture medium following reduction of BA to 0.2 mg l−1. Single shoots were then moved to rooting medium containing 0.6 mg l−1 IBA and 5 µg/l spermin and spermidin (polyamides).
In vitro-derived shoots (0.5–0.7 cm in length) of MM 106 (Northen spy×M1) were propagated in vitro on DKW proliferation medium (Driver and Kuniyuki Citation1984) supplemented with 1 mg l−1 thiamine, 100 mg l−1 myoinositol, 30 g l−1 sucrose, 10 g/l sorbitol; 150 mg/l fluoroglucinol, 2.0 mg l−1 BA, 0.2 mg/l GA3, 0.06 mg/l IBA pH 5.3, 6 g l−1 agar (Fluka) and 4 g l−1 carragenin (Frimulsionm UF Cisalpina Food, Bergamo). Shoots were then elongated as for GF 677 (P. persica×P. amygdalus) (see above) following which single shoots were moved to rooting medium containing 0.8 mg l−1 IBA and 5 µg/l spermin and spermidin.
For all three rootstocks, the cuttings were made up of apexes 15 mm in length comprising of three apical leaves. These were collected under aseptic conditions and transferred to culture jars containing agarized substrate for rooting. During the first week of the rooting phase, explants were kept in the dark. Mother plants and explants were then maintained in a growth chamber at a temperature of 24°C±1°C with a photoperiod of 16 h of light and 8 h of darkness and a light intensity 45±5 µmol m−2 sec−1 during proliferation and rooting. Rooted seedlings were used for subsequent tests for acclimatization. Each treatment applied to the above-mentioned rootstocks was replicated 20 times and glass tubes (20×160 mm), each containing one explant and 10 ml of substrate, were arranged in a randomized design.
Twenty days post rooting the plantlets were inoculated with A. brasilense Sp245 (106 cells/plant) and transplanted from axenic to in vivo conditions, using 12 ml pots (one plant/pot) filled with organic substrate (peat TKS1 Flora Gard, 34% organic carbon, 0.2% organic nitrogen, 60% organic matter, pH 5–6). Rooted plantlets were inoculated at the basal level with 3 ml (107 cells/ml) A. brasilense Sp245 or by root dipping in 50 ml (107 cells/ml) bacterial suspension for 24 h or for 48 h prior to trasplantation. The presence of bacteria was weekly monitored at rhizospheric level by plating as reported in Russo et al. (Citation2005). During the first week of acclimatization pots were placed in plastic boxes (simulating a tunnel condition) covered with a glass lid and maintained in a growth chamber at 24±2°C with 16 h photoperiod provided by cool white lamps (45±5 µmol m−2 s−1). Throughout this period, the glass cover was gradually pierced to allow the acclimatization of plants by reducing the relative humidity (RH). On alternate days, the pots were saturated with distilled water. Non-inoculated plants served as a control. Each treatment was replicated at least 20 times and the pots were arranged in a randomized complete design. Root number, root length, fresh root weight, node number, stem length and fresh stem weight were evaluated after 30 days of acclimatization (50-day-old plants). Plants were than transferred from growth chamber to greenhouse conditions (highest day temperature of 18°C lowest overnight temperature of 10°C) and periodically watered. Stem length and node number were assessed again at the end of the experiment (80-day-old plants). Plant growth conditions and data gathered during rooting and acclimatization phases are summarized in .
Statistical analysis
All experiments were performed at least in triplicate. The data were subjected to analysis of variance (ANOVA) with CoHort Software, 2003. In all bar diagrams each data point was marked as ‘a’ or ‘b’ or ‘ab’ indicating significant/insignificant differences between two data sets resulting from a multiple range test. The letter ‘a’ indicates the higher value of the bar diagram. Differences were considered to be significant at the 95% (or higher) confidence level.
Results and discussion
The transplantation from axenic to in vivo conditions is a critical phase of the in vitro propagation technique in which plants are subjected to numerous environmental stresses that may lead to significant plant loss. The aim of this work was the identification and evaluation of a novel method by using microrganisms to improve large-scale plant micropropagation of fruit rootstock clones. The presence of A. brasilense Sp245 was weekly monitored at rhizospheric level as reported in Russo et al. (Citation2005) attesting the persistence of 103–104 CFU/ml (data not shown).
The positive effects of A. brasilense Sp245 on the acclimatization phase of Mr.S 2/5, GF 677 and MM 106 fruit tree rootstocks are reported as follows.
Mr.S 2/5 clone
Large scale production of Mr.S 2/5 is usually performed by in vitro propagation technique and it is therefore of great commercial interest. shows results based on plant growth parameters following 30 days of acclimatization in growth chamber: root length, root weight, node number, stem length and stem weight were positively affected by Azospirillum. In particular the stem length of treated Mr.S 2/5 plants was 43% higher than the non-inoculated plants. This may possible be due to the production of indole-3-acetic acid (IAA), a phytohormone by Azospirillum brasilense Sp245 and reported as major contributing factor affecting plant growth and development by rhizobacteria. When released at the root level, this hormone can induce root development consequently increasing the overall plant nutritional uptake (Hassan et al. Citation2009). Furthermore, this observation is consistent with the recognition of the vital role of auxin in regulating stem elongation (Sachs Citation1965). An example of such a function is shown in cooled tulip bulbs wherein the internode elongation is promoted by exogenous treatment with IAA (Saniewski et al. Citation2006).
Figure 2. Root length, root weight, node number, stem length and stem weight were positively affect by Sp245, in 50-day-old Mr.S 2/5 plants (30 days after inoculum). C = control. The data were subjected to one-way ANOVA (p<0.05).
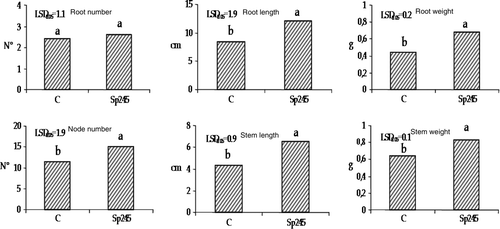
In the present study, 50-day-old plants were transferred to greenhouse conditions and at the end of acclimatization phase (corresponding to 80-day-old plants, 60 days post inoculation) exhibited significant differences between inoculated and uninoculated explants, at both stem length and node number, as shown in . Sixty days post inoculation, the positive effects of A. brasilense Sp245 on Mr.S 2/5 plum rootstocks indicated the permanence of certain growth-related compounds. Azospirillum spp. exhibited positive chemotaxis towards root exudates (Heinrich and Hess Citation1985) and colonized roots externally and internally by penetrating into the intercellular spaces so as to ensure long-term survival and persistent beneficial effects (Patriquin and Dobereiner Citation1978; Levanony and Bashan Citation1989; Vande Broek et al. Citation1993; Russo et al. Citation2005). During acclimatization phase, the inoculated Mr.S 2/5 plants showed a marked meristem apical activity when compared to the control, even though the shoot tips of control plantlets entered a dormant stage while Sp245-treated plant shoots demonstrated active apical growth for a significantly longer duration producing new nodes and leaves ().
Figure 3. Stem length and node number were positively affected by Sp245, in 80-day-old Mr.S 2/5 plants (60 days after inoculation. C = control. The data were subjected to one-way ANOVA (p<0.05). On the left, differences in plant growth and survival, between inoculated and uninoculated plants are compared.
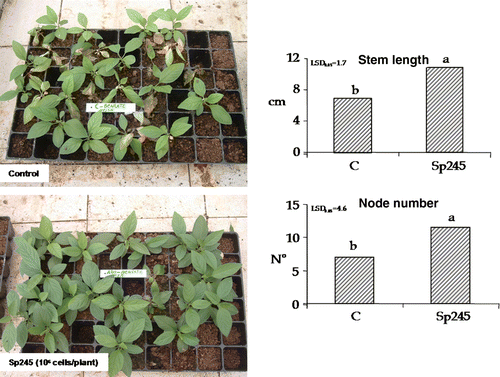
This might be one of the major factors that determine stem length of treated plants which was significantly greater as compared to the control. Moreover in this case, the beneficial effect of A. brasilense Sp245 could be correlated again due to the bacterial production of IAA at root level. Evidently, the apical buds of potentially generative plants contained less auxins than apical buds of plants which remained in the vegetative state (Teltscherová et al. Citation1976). All auxins play an important role in the elongation of plant cells, tropism, apical dominance, root formation and root elongation but indole-3-acetic acid is the most common natural auxin that demonstrates all these effects collectively therefore greatly influences plant physiology (Hassan et al. Citation2009). On this basis the effect of Azospirillum on meristem apical activity represents an important feature since inoculated plants could achieve the plant commercially viable length more rapidly, thus determining a remarkable reduction in terms of costs on an industrial scale.
Compared to inoculated plants, the uninoculated plants were of inferior quality, showing smaller and pale green leaves and higher rate of mortality. Approximately 50% of the uninoculated plants could not survive the described conditions versus 10% mortality rate of the inoculated plants (). The increased survival of plants treated with the bacterium can also possibly be related to the biocontrol activity of Azospirillum (Somers et al. Citation2005; Russo et al. Citation2008) since all the plants used in the experiment were not treated with fungicides nor with fertilizers, as is generally practiced during plant multiplication procedures in nurseries. In a subsequent experiment, 20-day-old plants rooted in a proper standard medium were transplanted from axenic to in vivo conditions (under tunnel, see Material and methods) and were inoculated at basal level with a bacterial suspension of A. brasilense Sp245. Plants were maintained in contact with the bacterial suspension for a brief period (30 sec). In addition, the contact time was increased to 24 and 48 h and the results from these differential dipping times were used to assess if the time of contact between roots and the bacterial suspension revealed a more pronounced beneficial effect. Thirty days post acclimatization significant differences in the growth parameters were observed between the treated plants when compared to the control. Greater vigor and larger leaf surface area were detected in all plants treated with A. brasilense Sp245. Initially, these results were simply assessed by a visual inspection, and appeared to be independent from the contact time of the plants with the bacterial suspension (30 s, 24 h, and 48 h). Consequently the number and weight of fresh roots were positively affected by Sp245 () suggesting that this rhizobacteria can induce plant growth promotion during the acclimatization phase of micropropagation.
Figure 4. Root number and root weight were positively affected by Sp245, in 50-day-old Mr.S 2/5 plants, 30 days after inoculum. (C = control. 0 h, 24 h, 48 h = plants dipped 30 sec, 24 or 48 h in 107 cells/ml Sp245 suspension. The data were subjected to two-way ANOVA (p<0.05).
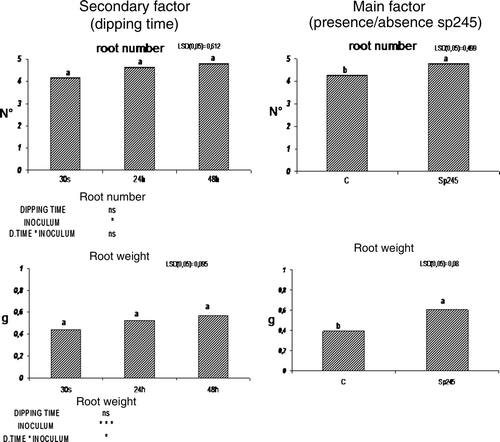
The two-way statistical analysis has highlighted an interaction between the main factor (presence/absence of A. brasilense Sp245) and the secondary factor (dipping time) (). Data on weight of fresh roots clearly suggested that the effect exerted by Azospirillum on fresh root weight was proportional to the dipping time in the bacterial suspension. This relationship in turn could be explained by a likely increase in the number of microorganisms that adhere to the plant tissue thereby bringing about a more pronounced effect of the inoculum. These effects are in accordance with Carletti (Citation2002), who reported the best response with a larger root surface area and greater root mass production of micropropagated plants by immersion of the basal part of shoots in a bacterial suspension (particularly A. brasilense) for a period of 24 h. At 50 days, the plants were transferred to greenhouse conditions for the final acclimatization stage. At the end of this phase (corresponding to 80-day-old plants, 60 days post inoculation) inoculated and uninoculated explants exhibited significant differences regarding fresh root weight, node number, stem length, fresh stem weight, as shown in .
Figure 5. Fresh root weight, node number, stem length, and fresh stem weight were positively affected by Sp245, in 80-day-old Mr.S 2/5 plants, 60 days after inoculum. (C = control. 0 h, 24 h, 48 h = plants dipped 30 sec, 24 or 48 h in 107 cells/ml Sp245 suspension. The data were subjected to two-way ANOVA (p<0.05).
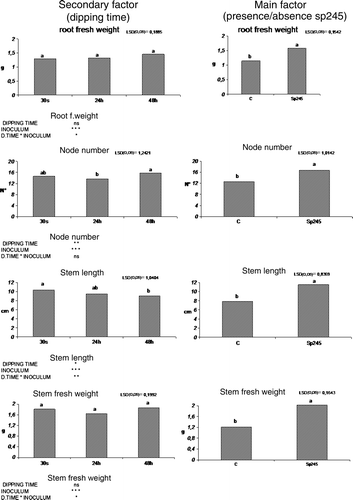
Interestingly, for fresh root weight, stem length and fresh stem weight two-way statistical analysis pointed out the interaction between the main factor (presence/absence of A. brasilense Sp245) and the secondary factor (dipping time) (). Similarly, data on fresh root weight showed that increasing dipping time in the bacterial suspension also increased the effect exerted by A. brasilense Sp245 on the weight of fresh roots. This observation, however, was inversely proportional with regard to the stem length and fresh stem weight. In fact, it was observed that an increase in the dipping time encouraged greater development of roots even though the effect was proved to be detrimental to stem growth and development. In the case of uninoculated control plants there were no differences observed after dipping in sterile water for different periods of time (). The treatment with the bacterium had a positive impact on the rapid development of the apical meristem when inoculation was performed during transplantation of in vitro propagated Mr.S 2/5. Plants showed an increase in bud apical activity of approximately 100% in all cases (). In particular, shoot tips of control plantlets precociously entered in a dormant stage while Azospirillum-treated plants showed apical growth for a much longer time producing new nodes and leaves. These effects, in accordance with what was reported by Carletti (Citation2002), could also depend on the fixation of atmospheric nitrogen, which this microorganism is capable of doing under conditions of microaerobiosis and low nitrogen levels. Although Azospirillum proliferates under both anaerobic and aerobic conditions it is preferentially a microaerophile bacterium both in the presence and absence of combined nitrogen in the medium (Okon and Itzigsohn Citation1995). Under microaerobic conditions and low nitrogen levels, Azospirillum can convert atmospheric nitrogen into ammonium through the action of nitrogenase complex (Steenhoudt and Vanderleyden Citation2000).
Figure 6. Percentage of actively growing buds and interaction between the main (presence/absence of A. brasilense Sp245) and secondary (dipping time) factors.
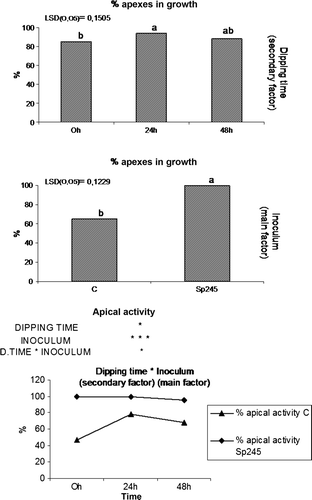
In order to determine if the beneficial effect played by A. brasilense Sp245 during the acclimatization of Mr.S 2/5 was limited not only to the propagation of this single species, other fruit tree rootstocks, GF 677 clone, which belongs to the same family of Drupaceae and MM 106, family Pomaceae, were also treated with the inoculum. MM 106 is different physiologically and morphologically from the other two clones.
GF 677 clone
The fruit tree rootstock GF 677 was inoculated with A. brasilense Sp245, applying the bacterial suspension at basal level or by dipping of the plants for a few seconds.
After 30 days, a significant effect was noted between control plants and plants inoculated with Sp245 in terms of root length, root weight and stem length (). The effect of a different method of inoculation was also evaluated in this case wherein either the entire plant was dipped in the bacterial suspension or by applying A. brasilense Sp245 at the basal level, directly on the roots. As seen in , both these two different treatments also gave positive responses compared to the control, but no significant differences were observed between the two different methods of bacterial inoculation. Significant differences between inoculated and uninoculated plantlets were observed in respect to the stem length and node number in 80-day-old plants (60 days post inoculation) ().
MM 106 clone
In the case of MM 106-inoculated plantlets (), the biomass production was significantly higher in respect to uninoculated control. Additionally, root weight and stem weight were positively affected by Sp245, in 50-day-old MM 106 plants (30 days after inoculation). No significant effects of Sp245 were observed on stem length and node number in 80-day-old MM 106 plants (60 days after inoculum). The more pronounced effects of Sp245 on Mr.S 2/5 and GF677 rootstocks in respect to MM 106, suggest that the beneficial effects of Sp245, at nursery level, depend on a specific clone-microbe association.
Figure 9. Root weight and stem weight were positively affected by Sp245, in 50-day-old plants (30 days after inoculum) MM 106. C = control. The data were subjected to one-way ANOVA (p<0.05).
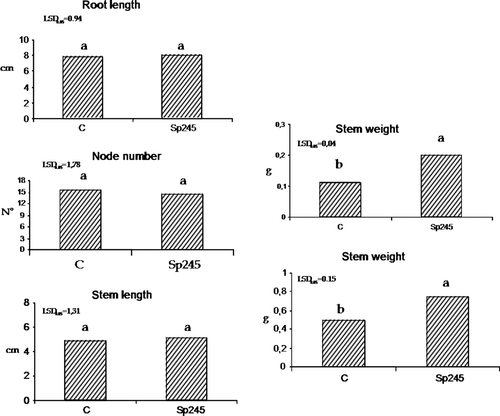
In conclusion, the proposed biotization of rootstocks of fruit tree (Mr.S 2/5 and GF 677, Drupaceae; MM 106, Pomaceae) with A. brasilense Sp245 as suspended cells could not only improve the quality of the resulting plants but could also lead to the production of vigorous plants with an evident resistence to biotic and abiotic stresses. Results are relevant expecially in the case of the Mr.S 2/5 clone that is at the moment one of the most widely commercialized plum rootstock produced by means of in vitro propagation. The use of this plant/microbe system could allow an appreciable reduction in the current use of chemical fertilizers and chemical fungicides leading to a reduction of environmental pollution normally created by the intensive greenhouse plants cultivations. This method could also significantly reduce the production time making the micropropagation technique highly competitive with the existing traditional plant propagation techniques. The main object of our future work is to investigate the molecular signaling that is likely to underline this interaction.
References
- Ahmed , Z , Akhter , F , Shamsul Haque , M , Banu , A , Motiur Rahman , M and Faruquzzaman , AKM. 2001 . Novel micropropagation system . J Biolog Sci. , 1 ( 11 ) : 1106 – 1111 .
- Baldani , VLD , Alvarez , MA , Baldani , JI and Döbereiner , J. 1986 . Establishment of inoculated Azospirillum spp. in the rhizosphere and in roots of field grown wheat and sorghum . Plant Soil. , 90 : 35 – 46 .
- Balla , I , Vrrtesy , J and Ktives-Pechy , K. 1997 . “ Acclimatation results of micropropagated black locust (Robinia pseudoacacia L.) improved by use of microorganisms ” . In Pathogen and microbial contamination management in micropropagation , Edited by: Cassells , AL . 351 – 354 . Dordrecht, NL : Kluwer Academic Publishers .
- Bashan , Y , Holguin , G and de-Bashan , LE. 2004 . Azospirillum-plant relationships: Physiological, molecular, agricultural, and environmental advances (1997–2003) . Can J Microbiol. , 50 ( 8 ) : 521 – 577 .
- Benhamou , N , Kloepper , J W and Quadt-Hallman , A. 1996 . Induction of defence-related ultra-structural modifications in pea root tissues inoculated with endophytic bacteria . Plant Physiol. , 112 : 919 – 929 .
- Bethlenfalvay , GJ , Andrade , G and Azcòn-Aguilar , C. 1997 . Plant and soil response to mycorrhizal fungi and rhizobacteria in nodulated or nitrate-fertilized peas (Pisum sativum L.) . Biol Fert Soils. , 24 : 164 – 168 .
- Carletti S. 2002 Use of plant growth-promoting Rhizobacteria in plant micro propagation Available on: www.ag.auburn.edu .
- Creus , CM , Sueldo , RJ and Barassi , CA. 1998 . Water relations in Azospirillum inoculated wheat seedlings under osmotic stress . Can J Bot. , 76 ( 2 ) : 238 – 244 .
- Dehne , HW. 1982 . Interactions between VAM fungi and plant pathogens . Phytopathology. , 72 : 1115 – 1119 .
- Dobbelaere S , Croonenborghs A , Thys A , Ptacek D , Vanderleyden J , Dutto P , Labandera-Gonzalez C , Caballero-Mellado J , Aguirre JF , Kapulnik Y , Brener S , Burdman S , Kadouri D , Sarig S , Okon Y . 2001 Response of agronomically important crops to inoculation with Azospirillum Aust J Plant Physiol. 28 : 871 879
- Dobbelaere , S , Vanderleyden , J and Okon , Y. 2003 . Plant growth-promoting effects of diazotrophs in the rhizosphere . CRC Crit Rev Plant Sci. , 22 : 107 – 149 .
- Driver , JA and Kuniyuki , AH. 1984 . In vitro propagation of Paradox walnut rootstock . HortScience. , 19 : 507 – 509 .
- Duffy , EM , Hurley , EM and Cassells , AC. 1999 . Weaning performance of potato microplants following bacterization and mycorrhization . Potato Res. , 42 : 521 – 527 .
- Grunewaldt-Stoecker , G. 1997 . “ Problems with the health of in vitro propagated Anthurium spp. and Phalaenopsis hybrids ” . In Pathogen and microbial contamination management in micropropagation , Edited by: Cassells , AC . 363 – 370 . Dordrecht, NL : Kluwer Academic Publishers .
- Hassan , E , Hossein , AA and Abolfazl , AA. 2009 . Evaluation of plant growth hormones production (IAA) ability by Iranian soils rhizobial strains and effects of superior strains application on wheat growth indexes . World Appl Sci J. , 6 ( 11 ) : 1576 – 1584 .
- Heinrich , D and Hess , D. 1985 . Chemotactic attraction of Azospirillum lipoferum by wheat roots and characterization of some attaractants . Can J Microbiol. , 31 : 26 – 31 .
- Hunt , MD and Ryals , JA. 1996 . Systemic acquired resistance signal transduction . Crit Rev Plant Sci. , 15 : 583 – 606 .
- Honda , H and Kobayashi , T. 2004 . Large-scale micropropagation system of plant cells . Adv Biochem Eng Biotechnol. , 91 : 105 – 134 .
- Hooker , JE , Black , KE , Perry , RL and Atkinson , D. 1995 . Arbuscular mycorrhizal fungi induced alteration to root longevity of poplar . Plant Soil. , 172 : 327 – 329 .
- Kloepper , JW , Tuzun , S and Zehnder , GW. 1997 . Multiple disease protection by rhizobacteria that induce systemic resistance-historical perspective . Phytopathology. , 87 : 136 – 137 .
- Lazarovits , G and Nowak , J. 1997 . Rhizobacteria for improvement of plant growth and establishment . HortScience. , 32 : 188 – 192 .
- Levanony , H and Bashan , Y. 1989 . Localization of specific antigens of Azospirillum brasilense Cd in its exopolysaccarhide by immuno-gold staining . Curr Microbiol. , 18 : 145 – 149 .
- Michiels , KW , Croes , CL and Vanderleyden , J. 1991 . Two different modes of attachment of Azospirillum brasilense Sp7 to wheat roots . J Gen Microbiol. , 137 : 2241 – 2246 .
- Murashige , T and Skoog , F. 1962 . A revised medium for rapid growth and bioassays with tobacco tissues cultures . Physiol Plant , 15 : 473 – 497 .
- Murphy , J , Mark , L and Periappuram , C. 1997 . “ Microbial characterisation and preparation of inoculum for in vitro mycorrhization of strawberry in autotrophic culture ” . In Pathogen and microbial contamination management in micropropagation , Edited by: Cassells , AL . 345 – 350 . Dordrecht, NL : Kluwer Academic Publishers .
- Nelson , CE. 1987 . “ The water relations of vesicular-arbuscular mycorrhizal systems ” . In Ecophysiology of V A mycorrhizal plants , Edited by: Safir , GR . 71 – 91 . Boca Raton, FL : CRC Press .
- Nowak , J. 1998 . Benefits of in vitro ‘biotization’ of plant tissue cultures with microbial inoculants . In Vitro Cell Dev Biol Plant. , 34 : 122 – 130 .
- Nowak , J , Asiedu , SK and Lazarovits , G. 1995 . “ Enhancement of in vitro growth and transplant stress tolerance of potato and vegetable plants cocultured with a plant growth promoting rhizobacterium ” . In Ecophysiology and photosynthetic in vitro cultures , Edited by: Carre , E and Chagvardieff , P . 173 – 180 . Aix-en-Provence, , France : CEA .
- Okon , Y and Itzigsohn , R. 1995 . The development of Azospirillum as a commercial inoculant for improving crop yields . Biotechnol Adv. , 13 ( 3 ) : 415 – 424 .
- Okon , Y and Labandera-Gonzales , CA. 1994 . Agronomic application of Azospirillum: An evaluation of 20 years of worldwide field inoculation . Soil Biol Biochem. , 26 : 1591 – 1601 .
- Patriquin , DG and Dobereiner , J. 1978 . Light microscopy observations of tetrazolium-reducing bacteria in the endorhizosphere of maize and other grasses in Brazil . Can J Microbiol. , 24 : 734 – 747 .
- Preece , JE and Sutter , EG. 1991 . “ Acclimation of micropropagated plants to the greenhouse and field ” . In Micropropagation, technology and application , Edited by: Debergh , PC and Zimmerman , RH . 71 – 93 . Dordrecht, NL : Kluwer Academic Publishers .
- Russo , A , Felici , C , Toffanin , A , Gotz , M , Collados , C , Barea , JM , Moenne-Loccoz , Y , Smalla , K , Vanderleyden , J and Nuti , MP. 2005 . Effect of Azospirillum inoculants on arbuscolar mycorrhizza establishment in wheat and maize plants . Biol Fertil Soils. , 41 : 301 – 309 .
- Russo , A , Vettori , L , Felici , C , Fiaschi , G , Morini , S and Toffanin , A. 2008 . Enhanced micropropagation response and biocontrol effect of Azospirillum brasilense Sp245 on Prunus cerasifera L. clone Mr.S 2/5 plants . J Biotechnol. , 134 : 312 – 319 .
- Sachs , RM. 1965 . Stem elongation . Annu Rev Plant Physiol. , 16 : 73 – 96 .
- Saniewski , M , Ueda , J , Miyamoto , K and Okubo , H. 2006 . Auxin transport and stem growth in tulips: The role of epidermis, cortex and pith . Acta Horticult. , 673 : 199 – 205 .
- Somers , E , Ptacek , D , Gysegom , P , Srinivasan , M and Vanderleyden , J. 2005 . Azospirillum brasilense produces the auxin-like phenylacetic acid by using the key enzyme for indole-3-Acetic acid biosynthesis . Appl Environ Microbiol. , 71 ( 4 ) : 1803 – 1810 .
- Steenhoudt , O and Vanderleyden , J. 2000 . Azospirillum, a free-living nitrogen-fixing bacterium closely associated with grasses: Genetic, biochemical and ecological aspects . FEMS Microbiol Rev. , 24 : 487 – 506 .
- Teltscherová , L , Pavlová , L and Pleskotová , D. 1976 . Changes in the content of endogenous auxins in apical buds of Chenopodium rubrum L. induced with respect to the endogenous rhythm in capacity to flower . Biologia Plantarum. , 19 ( 3 ) : 205 – 211 .
- Van Huylenbroeck , JM and Debergh , PC. 1996 . Physiological aspects in acclimatization of micropropagated plantlets . Plant Tissue Cult Biotech. , 2 : 136 – 141 .
- Vande Broek , A , Michielis , J , Van Gool , A and Vanderleyden , J. 1993 . Spatial-temporal colonization patterns of Azospirillum brasiliense on the wheat root surface and expression of bacterial nifH gene during association . Mol Plant-Microbe Interact. , 6 : 592 – 600 .
- Vestberg , M , Kukkonen , S , Saari , K , Parikka , P , Huttunen , J , Tainio , L , Devos , N , Weekers , F , Kevers , C , Thonart , P , Lemoine , MC , Cordier , C , Alabouvette , C and Gianinazzi , S. 2004 . Microbial inoculation for improving the growth and health of micropropagated strawberry . Appl Soil Ecol. , 27 : 243 – 258 .
- Wilhelm , E , Arthofer , W and Schafleitner , R. 1997 . “ Bacillus subtilis an endophyte of chestnut (Castanea sativa) as antagonist against chestnut blight (Cryphonectaria parasitica) ” . In Pathogen and microbial contamination management in micropropagation , Edited by: Cassells , AC . 331 – 337 . Dordrecht, NL : Kluwer Academic Publishers .
- Williamson , B , Cooke , DEL , Duncan , JM , Leifert , C , Breese , WA and Shattock , RC. 1997 . “ Fungal infections of micropropagated plants at weaning: A problem exemplified by downy mildew in Rubus at weaning ” . In Pathogen and microbial contamination management in micropropagation , Edited by: Cassells , AC . 309 – 320 . Dordrecht, NL : Kluwer Academic Publishers .