Abstract
Rotten onion bulbs were collected from different markets in Assuit governorate and screened for the presence of phytopathogenic bacteria using yeast extract-dextrose-calcium carbonate medium and King's medium B. Thirty-five bacterial isolates were recovered from 15 onion bulb samples. Twenty-five bacterial isolates were classified as putative Pseudomonas aeruginosa. Ten other unidentified bacteria were isolated at the same time and designated as Gram negative bacteria and nonflourescents. None of these bacteria have been reported as being pathogenic and it is likely that these other bacteria were present as concomitant/commensal bacteria. The causal agent of onion bulb rot was identified as Pseudomonas based on phenotypic characteristics. One representative isolate of these has been further identified as a Pseudomonas aeruginosa by sequencing of the 16S rRNA gene. The bacteria have the ability to grow in a temperature range of 4–43°C, with optimal growth at 37°C. Virulence of the Pseudomonas aeruginosa was also confirmed by the production and secretion of a large variety of enzymes capable of degrading the complex polysaccharides of the plant cell wall and membrane constituents. Scanning electron micrographs indicated that the Pseudomonas aeruginosa isolates were able to abundantly colonize the internal tissue of fleshy scales of onion bulbs. The virulence of the pathogen may be attributed to biofilm formation on the plant cell wall via fibrous materials as revealed by scanning electron microscopy as well as high activity of proteases, lipase, pectinases, and alkaline phosphates. This is the first evidence showing that a Pseudomonas aeruginosa strain E can cause soft rot of onion bulbs.
Introduction
Phytopathogenic bacteria contribute to a worldwide crop loss of roughly 10–15% annually (FAO Citation2005; Strange and Scott Citation2005). Plant pathogenic bacteria impact innumerable and valuable agriculture crops, causing hundreds of millions of dollars in damage each year. Pathogenic bacteria affecting plant health are a major and chronic threat to food production and ecosystem stability worldwide (Ste'phane et al. Citation2005; Abd-Alla and Bashandy Citation2008; Abd-Alla et al. Citation2010).
Onion (Allium cepa) is one-food ingredients widely used in our gastronomy. Moreover, onion extract has been recently reported to be effective in cardiovascular disease, because of their hypocholesterolemic, hypolipidemic, anti-hypertensive, anti-diabetic, antithrombotic and anti-hyperhomocysteinemia effects, and to possess many other biological activities including antimicrobial, antioxidant, anticarcinogenic, antimutagenic, antiasthmatic, immunomodulatory, and prebiotic activities. (Corzo-Martínez et al. Citation2007).
Bacterial soft rot is most common on onions in storage or transit; however, this disease can develop on onions in the field before harvest, after heavy rains, and when leaves are drying. The main sources of inoculum are contaminated soil and crop residues. The bacteria are spread by splashing rain, irrigation water, and insects. Entry into the bulbs is only through wounds such as those caused by transplanting, mechanical injuries, or sunscald. Also, onion maggots can carry bacteria and introduce them while feeding. This disease is favored by warm, humid conditions with optimum temperature range of 20–30oC. However, during storage or transit, it can develop when temperatures are above 30°C (Kim et al. Citation2002). It is assumed that the disease occurs severely during storage because Pseudomonas aeruginosa contaminates onion bulbs during harvest by moving through wounds caused by topping, finally causing soft rot. Therefore, if onions were infected in the field and carried into the warehouse, severe damage of onion bulbs may occur. More recently, Jacobs et al. (2008) reported that the majority of isolates identified as Burkholderia cepacia (85%), Burkholderia cenocepacia (90%), and Burkholderia ambifaria (76%) were pathogenic in a detached onion bulb scale assay and caused symptoms of water-soaking, maceration, and/or necrosis. Onion is now cultivated worldwide and commonly found in vegetable markets of most countries as a vegetable for human consumption. Its value among the vegetables is second after tomato (FAO Citation2005). Annual production and storage losses in garlic and onions as result of diseases can range from a trace to 50% or more depending upon the location, environment, and the causal agent involved (Schwartz and Mohan Citation1996).
Phytopathogenic pseudomonads are worldwide in distribution and cause diseases on most major groups of higher plants (Walker et al. Citation2004; Schroth et al. Citation2006). P. aeruginosa and P. cepacia are interesting in that they are among the few plant pathogens that have been implicated as causing diseases of plants and animals (Rahme et al. Citation1995), causing damage only when plants are subjected to stress such as high temperatures and moisture (Cother et al. Citation1976; Tesoriero et al. Citation1982; Tomlinson Citation1985).
Soft rot of onion bulbs in Egypt normally is not tested for the presence of bacterial causal agent. The objectives of this study was to determine and characterize the causal agent of soft root of onion bulbs collected from different vegetable markets and their ability to produce an array of extracellular enzymes capable of degrading complex polysaccharides of the plant cell wall and membrane constituents. The effect of temperature on growth and enzymes production by P. aeruginosa was also investigated.
Material and method
Isolation of bacterial causal agents
Onion bulbs showing water-soaking or yellowish brown rot in storage condition were used for isolation. Fifteen infected onion bulbs with soft rot were collected from different vegetable markets of Assuit governorate, Egypt in April 2008. The samples were placed in sterile polyethylene bags, which were taken to laboratory and processed within 3 h after collection. Rotten onion bulbs were washed by tap water and disinfected by soaking in bleach at 1% active sodium hypochlorite for 3 min, followed by rinsing in sterile distilled water. The diseased fleshly scale tissues were cut into small pieces by using sterile surgical blade. These pieces of onion scale were ground in 1 mL of sterilized saline phosphate buffer (pH 7) using mortar and pestle. A 10-fold-dilution series was prepared from suspensions of each sample extract and 100 µL of each dilution and the undiluted extract were spread on yeast extract-dextrose-calcium carbonate (YDC) medium (Schaad Citation1988) and King's medium B (King et al. Citation1954) with three replications of each dilution. Plates were incubated for 48 h at 30°C. Colonies were re-streaked onto YDC plates for pure culture isolation. Bacterial isolates were purified and retained on YDC and KB slants at 4°C for further tests of identification.
Pathogenicity test
Healthy onion bulbs were used for the pathogenicity test. With the use of a 0.25 G syringe needle, wounds were made on an onion bulb to inoculate the causal agent. The bacterial inocula were obtained from 2-day-old cultures on KB broth medium incubated at 30°C. Bacterial cells were collected in saline phosphate buffer and adjusted to107 cfu/mL. The bacterial causal agent was inoculated longitudinally from the neck part and transversely from the outer to the inner part of the onion bulb at the level of 100 µL on wound. Inoculated onion bulbs were incubated at 30oC in a moist chamber for 7 days. Inoculated bulbs were examined daily for development of symptoms. The causal agent was reisolated from onions bulbs showing the same symptoms of decaying and browning on King's Medium B.
Characterization and identification of the causal agent
Bacterial isolates were used for identification on the basis of its phenotypic characters using Bergey's manual (Garrity et al. Citation2005). Characterization of the presumptive pathogen was carried out by subjecting the isolated bacterial colonies to various biochemical tests like Gram's staining, Ryu's test, Fluorescent on King B, growth on cetrmide medium at different temperatures (4–43oC), denitrification, nitrate reduction, Kovac's oxidase test, Levan formation, catalase test, gelatin hydrolysis, starch hydrolysis, tween hydrolysis, carbohydrate utilization, H2S production from peptone and cysteine, and arginine dehydrolase test. Each test was conducted with three replicates for each strain and repeated twice.
Sequencing of 16S rRNA gene
DNA was extracted from bacterial cultures using a SDS/CTAB lysis and phenol/chloroform extraction method (Ausubel et al. Citation1987). The 16S rRNA gene was PCR-amplified using primer pairs 16S-F 10430 = AGAGTTTGATCCTGGCTCAG and16S-R10430 = AAGGAGGTGATTCC AGCC were used to amplify a near-full length, approximately 1500 bp fragment of 16S rDNA from the isolates. Amplification of 16S rRNA fragments from genomic DNA was carried out in out in a total reaction volume of 100 µl containing: 5 µl of bacteria DNA as template, 5 µl of each Forward and reverse primer (10 µM), 50 µl Go TagR Master Mix, 2x Kit (Promega, USA) and 35 µl of nuclease-free water. The reaction was performed using Eppendorf Ag 22331 Authorized Thermal Cycler (Hamburg, Germany) at Laboratory of plant molecular biology, Department of Applied Biological chemistry, Niigata University, Niigata, Japan. The reaction conditions were: an initial denaturation at 95oC for 3 min, 35 cycles of denaturation at 94oC for 70 sec, annealing at 56oC for 40 sec, and extension at 72oC for 130 sec. A final extension was conducted at 72oC for 370 sec. PCR products were purified (MonoFas DNA Purification Kit I, GL Sciences, nc. Japan) and quantified photometrically (UV mini-1240 Shimadzu). Purified PCR products were cycle sequenced in both directions with the same forward primer and reverser primer using Applied Biosystems 3730X-1 DNA Analyzer (Fast Smack Inc. Division DNA synthesis, Kanagawa, Japan). The sequence reads were edited and assembled using BioEdit version 7.0.4 (http://www.mbio.ncsu.edu/BioEdit/bioedit.html) and clusstalw version 1.83(http://clustalw.ddbj.nig.ac.jp/top-e.html). BLAST searches were done using the NCBI server at http:// www.ncbi.nlm.nih.gov/blast/Blast.cgi. Phylogenetic trees derived from 16S rRNA gene sequence was constructed in the context of 16S rRNA gene sequences from eight different standard bacterial strains obtained from Genbank (Pseudomonas aeruginosa strain NBAII AFP-13 (HQ162487), P. aeruginosa (AF411219), P. aeruginosa (AY486370), P. halodenitrificans (X90867), P. oleovorans (Z76665), P. thermaerum (AB088116), P. resinovorans (AJ308314), and Bacillus subtilis (FJ482099)).
Media and growth conditions for enzymes production
Phytopathogenic bacterial isolates were screened for their ability to elaborate hydrolytic enzymes such as lipase, protease, Pectinolytic, and Cellulase enzyme. For the production of protease, tested bacterial isolates were grown in 50 mL of liquid medium in an Erlenmeyer flask (250 mL) containing (g/L): MgSO4.7H2O 0.2, K2HPO4 2.0, KH2PO4 2, and casein 10 (pH 8) (Chakraborty and Srinivasan Citation1993). The basal medium for lipase production consisted of (g/L): bacteriological peptone 15.0, yeast extract 5.0, NaCl 2.0, MgSO4 0.4, K2HPO4 0.3, KH2PO4 0.3, and olive oil 10.0 mL for lipase induction (Baharum et al. Citation2003). The basal medium for pectinase production consisted of (g/L): pectin 4, yeast extract 2, NH4Cl 1, MgSO4 0.5 (Gomes et al. Citation1992). Cultures were incubated in an orbital shaking incubator for 36 h at 150 rpm and 37oC. The culture broth was then centrifuged at 8000 rpm to remove cells. The clear supernatant was collected for enzymes assay.
Enzymes assay
Protease activity was assayed by a modified method of Ohara-Nemoto et al. (Citation1994). The reaction was initiated by addition of 1 mL supernatant to 2 mL of reaction mixture containing 2.7 mg of casein per mL in 50 mmol Tris-HCl (pH 8.0) which had been prewarmed at 37°C. After incubation at 37°C for 1 h, the reaction was stopped by addition of 0.5 mL of 15% ice-cold trichloroacetic acid. The reaction mixture was held on ice for 15 min and then centrifuged; 5 mL of sodium carbonate solution (500 mmol) was added to reaction mixture then 1 mL of Folin and Ciocalteu's Phenol Reagent (Dilute 10 mL of Folin and Ciocalteu's Phenol Reagent, to 40 mL with distilled water). The soluble peptide in the supernatant fraction was measured with tyrosine as the reference compound (Folin and Ciocalteu Citation1929). The absorbance at 660 nm of the sample was measured with UV-2100 spectrophotometer. One unit of enzyme activity was defined as the amount of enzyme that releases 1 µg of tyrosine per minute under the assay conditions. Controls contained autoclaved enzymes instead of active enzymes were used. Lipase activity was measured by universal titrimetric method (FadIloğlu and SÖylemez Citation1997).
The hydrolytic pectic enzymes were determined according to method of Gomes et al. (1992). A unit of activity is defined as that amount of enzyme which catalyzes the release of 1 µmol of reducing groups per minute and expressed as U/mL.
Alkaline phosphatase of bacterial culture supernatant was determined by standard assay procedure using alkaline phosphatase kit (ELI Tech, SEPPIM S.A.S. – Zone industrielle – 61500 SEES France). The reaction mixture, containing 1 mL of bacterial culture supernatant, 1 mL of 50 mmol p-nitrophenyl phosphate as substrate, and 2 mL of buffer solution of pH 10.2 (1.6 mL of 1.4 mol diethanolamine and 0.4 mL of 0.625 mol magnesium chloride) and incubated at 37°C for 1 h. The reaction was terminated by adding 1 mL of 1 N NaOH and the absorbance of p-nitrophenol formed was measured at 405 nm. Blank was prepared by using boiled bacterial supernatant. One alkaline phosphatase unit was defined as the amount of enzyme, which liberates 1 µmol of p-nitrophenol as a result of hydrolysis of p-nitrophenylphosphate (pNPP) in 1 min (Abd-Alla Citation1994).
Effects of temperature on enzyme production and stability
The effect of temperature on enzyme production was determined by incubating the culture flasks with different temperature regimes (10, 20, 30, and 40°C). For determining thermal stability, the enzyme was pre-incubated at different time ranging from 10 to 70 min at maximum temperature below the optimum and residual activity was measured under standard assay conditions.
Protein measurement
Cellular protein was determined by the method of Lowry et al. (Citation1951) and with bovine serum albumin as the standard.
Scanning electron microscopy
Small pieces of fleshy scale leaf tissue were fixed first in a 2% (w/v) glutaraldehyde solution in 50 mmol phosphate buffer at pH 7.2 and kept at room temperature (Abd-Alla et al. Citation2000). Fixed samples were washed three times in the same buffer (each time for 30 min) and post-fixed in 1% (w/v) osmium tetroxide solution in the same buffer at 4oC for 2 days. Preparations were then slowly dehydrated by passage through increasing alcohol concentrations (10, 30, 50, 70, 90, 99.5, and 100%) at 4±1°C. The samples were dried in a critical-point dryer in a CO2copper stubs with carbon cement and coated with a 150 Å layer of gold in a Joel JFC-1100 E ion Sputtering Device. Samples were examined by Joel JSM-5400 LV scanning electron microscope at 15 KV. All images were computer processed.
Nucleotide sequence accession number
The nucleotide sequences of Pseudomonas aeruginosa have been deposited in the GenBank nucleotide sequence database.
Results
Isolation and identification
Thirty-five bacterial isolates were recovered from 15 rotten onion bulbs (Allium cepa) collected from different vegetable markets of Assuit governorate on YDC and KB media (). Twenty-five of the bacterial isolates were able to produce water-soluble yellow–green pigments (pyoverdin) that fluoresce under ultraviolet light and red–brown pigments (pyorubin) on King's B medium. They produce blue–green (pyocyanin) and on King's B medium. Also, 10 other bacterial isolates with yellow colonies were isolated as the same time. All the isolates recovered from diseased onion were individually inoculated onto healthy storage and cultivated onion. Twenty-five isolates produced similar symptom on inoculated onion and caused typical symptom of rot bulb. The other 10 bacterial isolates with yellow colonies did not show any symptoms on storage and cultivated onion bulbs.
The 25 isolates were reisolated from artificially inoculated plants showing the symptoms were similar to those due to natural infections. The symptoms developed along the outer fleshy scales of onion after 7 days of artificially inoculation. Infected tissue is yellow initially, turning into brown as the disease progresses lengthwise in the bulb. Water-soaking (soft rot) developed gradually into inner part of onion bulbs (). The 25 isolates were usually Gram negative; short rod-shaped, motile, and non-spore-forming (). All of the bacterial isolates were able to grow on cetrimide medium at temperature ranged between 4 and 43oC with optimum temperature of 37oC (for physiological and biochemical characteristics see ).
Figure 2. Pathogenicity test of dry onion bulbs (Allium cepa) shows the colonization of soft rot pathogen. The infection symptoms developed along the outer fleshy scales of onion after 7 days of artificially inoculation with Pseudomonas aeruginosa (A). Water soaking (soft rot) developed gradually into inner part of onion bulbs (B).
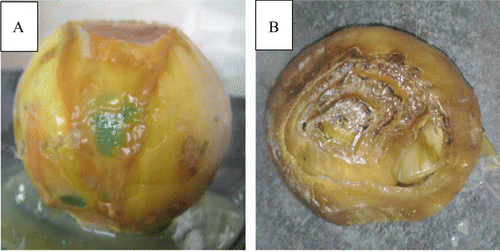
Table 1. General phenotypic characteristic of Pseudomonas aeruginosa
On the basis of phenotypic traits and the pathogenicity test revealed that the causal agent of rot onion bulbs was identified as Pseudomonas. One representative isolate E was chosen for further identification using phylogenetic analysis of 16S rRNA gene sequences. The partial 16S rRNA gene sequence of 800 base pairs of the representative isolate had a sequence with 97% similarity to Pseudomonas aeruginosa strain NBAII AFP-13 (HQ162487). A phylogenetic tree was constructed from multiple sequences alignment of 16S rRNA gene sequences ().
Figure 3. Phylogenetic tree indicates the phylogentic relationship of the isolated strain. Isolate is indicated in bold. A neighbor-joining tree was calculated using partial 16S rRNA gene sequences (800 bp) and a frequency filter included in the ARB software package. Bacillus subtilis (FJ482099) was used as out group. The scale bar indicates 10% estimated sequence difference. Accession numbers of the National Center for Biotechnology Information (NCBI) database of each strain are given in brackets.
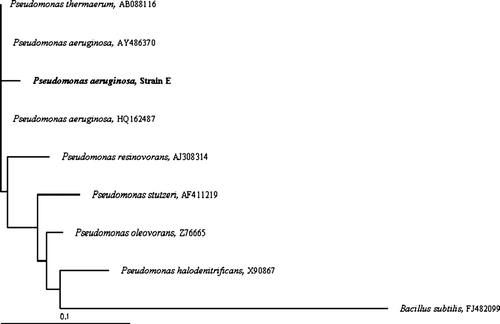
Determination of virulence factor (enzyme activity) of Pseudomonas aeruginosa
The colonies showing hydrolytic activity were subjected to quantitative enzyme production in liquid culture media. Pseudomonas aeruginosa has the ability to produce the extracelluar enzyme which thought to play an important role in plant pathology. Protease, lipase, polygalactronase, and alkaline phosphatase were detected by Pseudomonas aeruginosa isolates.
The effect of different temperatures on lipase, protease, and polygalactronase production was examined. The optimum temperature for higher lipase, protease, and alkaline phosphatase production was 30oC whereas the optimum temperature for polygalacturonase production was 40oC (). The lipase enzyme of Pseudomonas aeruginosa retained about 88% of initial activity after 10 min and loss about 57% of remaining activity after 40 min at 50oC. The protease enzyme of Pseudomonas aeruginosa retained about 72% of initial activity after 10 min and loss about 48% of remaining activity after 40 min at 55oC. The polygalacturonase enzyme of Pseudomonas aeruginosa retained about 91% of initial activity after 10 min and loss about 66% of remaining activity after 40 min at 55oC. The alkaline phosphatase enzyme of Pseudomonas aeruginosa retained about 95% of initial activity after 10 min and loss about 70% of remaining activity after 40 min at 50oC.
Visualization of pathogenicity by scanning electron microscope
In the experiments described in the previous section (Pathogenicity test) health onion scale leaf bulbs were inoculated with Pseudomonas aeruginosa and incubated at room temperature. Two days after bacterial inoculation, the bacteria began multiplying and rapidly spread through the leaf mesophyll (A). Scanning electron micrograph indicates that, Pseudomonas aeruginosa was able to abundantly colonize the intercellular space, presumably by digesting the middle lamellae and separating the plant cells from each other (C). Infected onion tissue was showing maceration and loosening as compared with uninfected onion fleshy leaf cells. At relatively early stages of the infection almost all the bacteria were found in intercellular spaces attached to cell walls, although some bacterial cells could also be observed attached to the inner surface of cell walls (A). After proliferation in the intercellular space at the site of infection, bacterial cells entered parenchyma cells, then multiplied and spread from one parenchyma to the next (B, C and D). Many of the mesophyll cells were severely damaged and contained intracellular bacteria. Bacteria in onion fleshy leaf cells were frequently associated with fine web-like, fibrous material. The bacterial cells connected to each other via fibrous structure or fimbriae (E). Bacteria appear to be attached to plant cell wall and plant cell matrix by fibrillar material (F).
Figure 5. Scanning electron micrographs show internal colonization of fleshy scale leaves cells by Pseudomonas aeruginosa (single arrows, A, C, E) 7 days after inoculation as compared with uninfected fleshy scale leaves cells (B, D, F). Note the infected onion fleshy scale leaves cells show cell wall loosening and maceration (double arrows, A, C) as compared with uninfected onion fleshy scale leave cells (B, D).
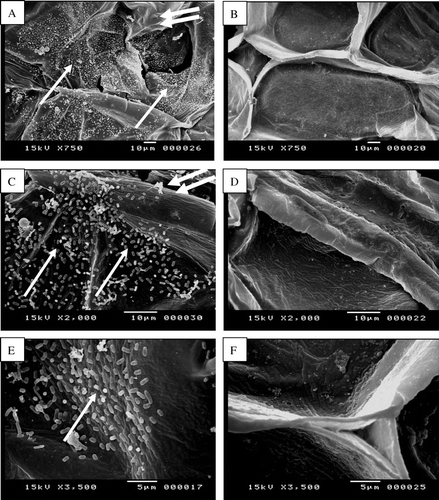
Figure 6. Scanning electron micrographs show internal colonization of onion fleshy scale leaf cells by Pseudomonas aeruginosa (A) Onion fleshy scale leaf cell showing dense bacterial population (arrow). (B) Focus on a bacterial biofilm. Note the matrix (m) embedding bacterial cells constituting a typical biofilm. (C & D) Focus on multiplication of bacterial cell in internal leaf tissues (arrows). (E) Enlargement of (D) showing the bacterial cells connected to each other via fibrous structure (arrorws). (F) Bacteria appear to be attached to plant cell wall and plant cell matrix by fibrillar material (arrows).
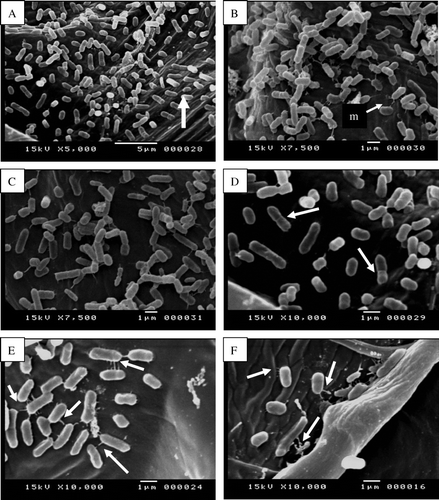
Discussion
A collection of 25 bacterial isolate obtained from the rotten onion bulbs were identified as Pseudomonas aeruginosa based on the classical phenotypic characterization and the 16S rRNA gene sequencing. This bacterium caused similar symptoms on stored onion bulbs artificially inoculated at 30°C and after 2–7 days. A similar disease on onion bulbs caused by Burkholderia cepacia was previously reported by Yi and Park (Citation1999). Sotokawa and Takikawa (Citation2004) reported that Pseudomonas marginalis, Burkholderia cepacia caused onion bulb rot in fields and marketplaces. However, Pseudomonas aeruginosa was readily distinguished from Burkholderia cepacia in terms of various biological characteristics. Pseudomonas marginalis is a known opportunistic pathogen causing soft rot on a wide range of hosts including onion, lettuce, and Chinese cabbage (Lelliott and Stead Citation1987).
Choi and Han (Citation1990) reported that Pseudomonas marginalis, Pseudomonas syringae, and Pseudomonas cepacia caused onion bulb rot in fields and marketplaces. Soft rot of onion bulbs caused by Pseudomonas marginalis stored under low temperature has been reported previously (Kim et al. Citation2002). When onions are harvested from the field, they are undercut and hand pulled. Sometimes the tops are trimmed and the bulbs are then allowed to dry for 2–7 days under field condition. Leaf wounds caused by topping during harvesting are considered as important entrance sites for the pathogen. It is assumed that the disease occurs severely during storage because Pseudomonas aeruginosa contaminates onion bulbs during harvest by moving through wounds caused by topping, finally causing soft rot (Cother and Dowling Citation1986; Gupta et al. Citation1986). Therefore, if onions were infected in the field and carried into the warehouse, severe damage of onion bulbs may occur. More recently, Jacobs et al. (Citation2008) reported that the majority of isolates identified as Burkholderia cepacia (85%), Burkholderia cenocepacia (90%), and Burkholderia ambifaria (76%) were pathogenic in a detached onion bulb scale assay and caused symptoms of water-soaking, maceration, and/or necrosis.
Pseudomonas aeruginosa PA14 was shown to infect disparate species by using the same virulence factors (Hendrickson et al. Citation2001). It is clear that virulence gene expression in biofilms requires a live poplar tree as Pseudomonas aeruginosa genes were clearly differentially expressed relative to the inert solid.
Attila et al. (Citation2007) demonstrate that Pseudomonas aeruginosa PAO1 is a pathogen for poplar trees and barley. Starkey and Rahme (Citation2009) reported that Pseudomonas aeruginosa is a pathogen for lettuce and Arabidopsis.
Scanning electron microscope observation in current research revealed that Pseudomonas aeruginosa parasitism in onion fleshy leaf starts after bacterial attachment to the plant leaf surface and entry into onion tissues by moving through wounds caused by topping during harvesting, followed by proliferation in the intercellular space. As the bacteria proliferate onion cell walls become undulated and at least some bacteria appear to penetrate through the walls. Bacteria located in the intercellular space results in maceration and autolysis of plant cells. A systemic infection results from infection of parenchyma cells and movement of Pseudomonas aeruginosa from one parenchyma cell to the next. Many of the onion flesh leaf cells were severely damaged and contained intracellular bacteria. This observation came in a good agreement with those reported by other authors (Kabashnaya et al. Citation1988; Plotnikova et al. Citation2000; Jha et al. 2005, Hao and Xie Citation2006).
Extracellular cell wall-degrading enzymes are important to pathogenic bacteria to overcome host resistance and/or to utilize organic materials in the environment. Phytopathogenic bacteria secrete four major effectors to induce pathogenicity. Phytopathogenic bacteria secrete effectors type I (proteases, lipases) and type II (Pectinate lyase, cellulose) to the intercellular spaces, or directly translocate effectors (type III and IV) into the plant cell (Alfano and Collmer Citation1996). Lipase is recruited to act on plant cell walls to promote virulence, thus providing a remarkable example of the emergence of novel functions around existing scaffolds for increased proficiency of pathogenesis during pathogen-plant coevolution (Aparna et al. Citation2009). The activity of the type of induced enzyme may be influenced by environmental factors. Their activity may be significantly diminished or destroyed by a variety of physical or chemical agents resulting in a loss of the functions performed by the enzymes. A characteristic feature of many phytopathogenic organisms is their ability to produce an array of enzymes capable of degrading the complex polysaccharides of the plant cell wall and membrane constituents. These enzymes are usually produced inductively and are extracellular, highly stable and present in infected host tissue (Bateman and Basham Citation1976). A major virulence factor in onion pathogenicity is the presence of a polygalacturonase enzyme involved in tissue maceration that is encoded by the plasmid-borne pehA gene (Gonzalez et al. Citation1997). Protease enzyme cleaves proteins into peptides. Protein degradation products such as di- and tripeptides are assimilated by bacteria. Proteases apparently coordinate with pectinate lyases to produce a synergistic increase in soft rot damage by digesting extensins, hydroxyproline-rich cell wall structural proteins, and loosening plant cell wall so that endopectinate lyases penetrate more effectively into its cellulose–pectin matrix. One of our Pseudomonas sp. isolate produced an extracellular thermostable protease. Growth of the organism and the production of protease were optimum at 30°C (Chakraborty and Srinivasan Citation1992). Yen et al. (Citation2006) reported that the optimum temperature for protease activity produced by Pseudomonas aeruginosa M-1001 was 37°C. Recent sequence analysis has revealed that Pseudomonas syringae pv. tomato strain DC3000 also encodes several potential cell wall degrading enzymes, including a polygalacturonase, a pectin lyase, and three enzymes predicted to have cellulolytic activity (Buell et al. Citation2003). The ability of soft rot bacteria to cause vegetable spoilage results mainly from their ability to produce massive amounts of enzymes.
Acknowledgements
This research was financially supported by Assuit University, Egypt.
References
- Abd-Alla , MH. 1994 . Phosphatases and the utilization of organic phosphorus by Rhizobium leguminosarium biovar viceae phosphatases . Lett Appl Microbiol , 18 : 294 – 296 .
- Abd-Alla , MH and Bashandy , SR. 2008 . Bacterial wilt and spot of tomato caused by Xanthomonas vesicatoria and Ralstonia solanacearum in Egypt . World J Microbiol Biotechnol. , 24 : 291 – 292 .
- Abd-Alla MH , Bashandy SR , Schnell S. 2010 . Occurrence of Xanthomonas axonopodis pv. phaseoli, the causal agent of common bacterial blight disease, on seeds of common bean (Phaseolus vulgaris L) in Upper Egypt . Folia Microbiol . 55 : 47 – 52 .
- Abd-Alla , MH , Kyro , HV , Yan , F , Shubert , S and Peiter , E. 2000 . Functional structure of faba bean nodules: implications for metabolite transport . J Plant Physiol. , 157 : 335 – 343 .
- Alfano , JR and Collmer , A. 1996 . Bacterial pathogens in plants: life up against the wall . Plant Cell , 8 : 1683 – 1698 .
- Aparna G , Chatterjee A , Sonti RV , Sankaranarayanan R. 2009 . A cell wall-degrading esterase of Xanthomonas oryzaerequires a unique substrate recognition module for pathogenesis on rice. Plant Cell 21 : 1860 – 1873 Advance Online Publication. doi: 10.1105/tpc.109.066886
- Attila , C , Ueda , A , Cirillo , SLG , Cirillo , JD , Chen , W and Wood , TK. 2007 . Pseudomonas aeruginosa PAO1 virulence factors and poplar tree response in the rhizosphere . Microb Biotechnol. , 1 : 17 – 29 .
- Ausubel , FM , Brent , R , Kingston , RE , Moore , DD , Smith , JA and Struhl , K. 1987 . Current protocols in molecular biology , New York : Greene Publishing Associates and Wiley-Interscience, John Wiley .
- Baharum , SN , Salleh , AB , Razak , CNA , Basri , M , Rahman , MBA and Rahman , RNZRA. 2003 . Organic solvent tolerant lipase by Pseudomonas sp. strain S5: stability of enzyme in organic solvent and physical factors affecting its production . Ann Microbiol. , 53 : 75 – 83 .
- Bateman DF , Basham HG. 1976 . Degradation of plant cell walls and membranes by microbial enzymes . In: Heitefuss R , Williams PH Encyclopedia of Plant Physiology , New York : Springer-Verlag 4 . 316 – 355 .
- Buell , CR , Joardar , V , Lindeberg , M , Joardar , V , Lindeberg , M , Selengut , J , Paulsen , IT , Gwinn , ML , Dodson , RJ Deboy , RT . 2003 . The complete genome sequence of the Arabidopsis and tomato pathogen Pseudomonas syringae pv. tomato DC3000 . Proc Natl Acad Sci USA. , 100 : 10181 – 10186 .
- Chakraborty , R and Srinivasan , M. 1992 . Production and regulation of a thermostable protease by Pseudomonas sp. B45 . Acta Microbiol Hung. , 39 : 181 – 191 .
- Chakraborty , R and Srinivasan , M. 1993 . Production of a thermostable alkaline protease by a new Pseudomonas sp. by solid substrate fermentation . J Microbiol Biotechnol. , 8 : 7 – 16 .
- Choi , JE and Han , KS. 1990 . Studies on the bacterial soft rot disease of lilliaceae crops in Korea. 4. Bacterial bulb rot of onion caused by Pseudomonas spp . Plant Pathol J. , 6 : 358 – 362 .
- Corzo-Martínez , M , Corzo , N and Villamiel , M. 2007 . Biological properties of onions and garlic . Trends Food Sci Technol. , 18 : 609 – 625 .
- Cother , EJ , Darbyshire , B and Brewer , J. 1976 . Pseudomonas aeruginosa – cause of internal brown rot of onion . Phytopathol. , 66 : 828 – 834 .
- Cother , EJ and Dowling , V. 1986 . Bacteria associated with internal breakdown of onion bulbs and their possible role in disease expression . Plant Pathol. , 35 : 329 – 336 .
- FadIloğlu , S and SÖylemez , Z. 1997 . Kinetics of lipase – catalyzed hydrolysis of olive oil . Food Res Int. , 30 : 171 – 175 .
- FAO . 2005 . The state of food insecurity in the world. Eradicating world hunger – key to achieving the Millenium Development Goals . Rome : Food and Agriculture Organization of the United Nations .
- Folin , O and Ciocalteu , V. 1929 . On tyrosine and tryptophane determinations in proteins . J Biol Chem. , 73 : 627 – 628 .
- Garrity GM , Bell JA , Lilburn T. , 2005 . Order IX. Pseudomonadales . In: Brenner DJ , Krieg NR , Staley JT , Garrity GM . Bergey's Manual of Systematic Bacteriology. , 2nd ed , vol. 2 (The Proteobacteria), part B (The Gammaproteobacteria) New York : Springer . p. 323 – 442 .
- Gomes , I , Saha , RK , Mohiuddin , G and Hoq , MM. 1992 . Isolation and characterization of cellulase-free pectinolytic and hemicellulolytic thermophilie fungus . World J Microbiol Biotechnol , 8 : 589 – 592 .
- Gonzalez , CF , Pettit , EA , Valadez , VA and Provin , EM. 1997 . Mobilization, cloning, and sequence determination of a plasmid-encoded polygalacturonase from a phytopathogenic Burkholderia (Pseudomonas) cepacia . Mol. Plant-Microbe Interact. , 10 : 840 – 851 .
- Gupta , RP , Usha , M and Pandey , UB. 1986 . Bacterial brown rot of onion in storage – a new record from India . Indian J Plant Pathol. , 4 : 184
- Hao , XJ and Xie , GL. 2006 . Internal brown rot of onion caused by an opportunistic bacterial pathogen (Pseudomonas aeruginosa) in China . J Plant Pathol. , 88 : 342 – 342 .
- Hendrickson , EL , Plotnikova , J , Mahajan-Miklos , S , Rahme , LG and Ausubel , FM. 2001 . Differential roles of the Pseudomonas aeruginosa PA14 rpoN gene in pathogenicity in plants, nematodes, insects, and mice . J Biotechnol. , 183 : 7126 – 7134 .
- Jacobs , JL , Fasi , AC , Ramette , A , Smith , JJ , Hammerschmidt , R and Sundin , GW. 2008 . Identification and onion pathogenicity of Burkholderia cepacia complex isolates from the onion rhizosphere and onion field soil . Appl Environ Microbiol. , 74 : 3121 – 3129 .
- Kabashnaya , LV , Gvozdyak , RI and Yakovleva , LM. 1988 . Pseudomonas aeruginosa an agent of onion rot in the Ukrainian SSR-USSR . Mikrobiologicheskii Zhurnal (Kiev) , 50 : 53 – 57 .
- Kim , Y-K , Lee , S-D , Choi , C-S , Lee , S-B and Lee , S-Y. 2002 . Soft rot of onion bulbs caused by Pseudomonas marginalis under low temperature storage . Plant Pathol. , 18 : 9 – 203 .
- King , EO , Ward , MK and Raney , DE. 1954 . Two simple media for the demonstration of pyocyanin and fluorescein . J Lab Clin Med. , 44 : 301 – 307 .
- Lelliott , RA and Stead , DE. 1987 . “ Methods for the diagnosis of bacterial diseases of plants ” . In Methods in plant pathology , Edited by: Preece , TF . 216 Oxford : Blackwell Scientific Publications .
- Lowry , OH , Rosebrough , NJ , Farr , AL and Randall , RJ. 1951 . Protein measurement with folin phenol reagent . J Biol Chem. , 193 : 265 – 275 .
- Ohara-Nemoto , Y , Sasaki , M , Kaneko , M , Nemoto , T and Ota , M. 1994 . Cysteine protease activity of streptococcal pyrogenic exotoxin B . J Clin Microbiol. , 40 : 930 – 936 .
- Plotnikova , JM , Rahme , LG and Ausubel , FM. 2000 . Pathogenesis of the human opportunistic pathogenPseudomonas aeruginosa PA14 in Arabidopsis . Plant Physiol. , 124 : 1766 – 1774 .
- Rahme , L , Stevens , E , Wolfort , S , Shao , J , Tompkins , R and Ausubel , FM. 1995 . Common virulence factors for bacterial pathogenicity in plants and animals . Science. , 268 : 1899 – 1902 .
- Schaad , NW. 1988 . Laboratory guide for identification of plant pathogenic bacteria , St. Paul, MN : APS Press .
- Schroth , MN , Hildebrand , DC and Panopoulos , N. 2006 . Phytopathogenic pseudomonads and related plant-associated pseudomonads . Prokaryotes. , 6 : 714 – 740 .
- Schwartz , HF and Mohan , SK. 1996 . Compedium of onion and garlic diseases , 54 St. Paul, MN : APS Press .
- Sotokawa , N and Takikawa , Y. 2004 . Occurrence of bacterial rot of onion bulbs caused by Burkholderia cepacia in Japan . J Gen Plant Pathol. , 70 : 348 – 352 .
- Starkey , M and Rahme , LG. 2009 . Modeling Pseudomonas aeruginosa pathogenesis in plant hosts . Nat Protoc. , 4 : 17 – 124 .
- Ste'phane , C , Brion , D , Jerzy , N , Christophe , C and Essaid , AB. 2005 . Use of plant growth-promoting bacteria for biocontrol of plant diseases: principles, mechanisms of action, and future prospects . Appl Environ Microbiol. , 71 : 4951 – 4959 .
- Strange , RN and Scott , PR. 2005 . Plant disease: a threat to global food security . Annu Rev Phytopathol. , 43 : 83 – 116 .
- Tesoriero , LA , Fahy , PC and Gunn , LV. 1982 . First record of bacterial rot of onion in Australia caused by Pseudomonas gladioli pv. alliicola and associated with internal browning caused by Pseudomonas aeruginosa . Austral Plant Pathol. , 11 : 56 – 57 .
- Tomlinson , DL. 1985 . Spoilage of stored onion (allium-cepa l var cepa) by Pseudomonas aeruginosa (schroeter) migula in lowland papua-new-guinea . Trop Pest Manage. , 31 : 214 – 216 .
- Walker , TS , Bais , HP , De′ziel , E , Schweizer , HP , Rahme , LG , Fall , R and Vivanco , JM. 2004 . Pseudomonas aeruginosa – plant root interactions, pathogenicity, biofilm formation, and root exudation . Plant Physiol. , 134 : 320 – 331 .
- Yen , Y-H , Pei-Ling , L , Chuan-Lu , W and San-Lang , W. 2006 . An antifungal protease produced by Pseudomonas aeruginosa M-1001 with shrimp and crab shell powder as a carbon source . Enzyme Microb Technol , 39 : 311 – 317 .
- Yi , YK and Park , YM. 1999 . Soft rot of onion stored under low temperature condition caused by Erwinia rhapontici and Burkholderia cepacia . J Agric Sci Technol. , 6 : 33 – 40 .