Abstract
Beneficial rhizobacteria strains are of substantial interest as biological plant protection agents in agriculture. Bacteria of the genus Pseudomonas have been studied for many years for their role in plant growth and biocontrol. In this study, we analyzed the influence of the commercially available agent Proradix®, which contains the strain Pseudomonas sp. DSMZ 13134, on barley. In controlled infection experiments, we showed that Pseudomonas sp. DSMZ 13134 induces resistance to the barley leaf pathogen Rhynchosporium secalis and inhibits the growth of the barley root pathogen Gaeumannomyces graminis. In greenhouse experiments, Pseudomonas sp. DSMZ 13134-treated plants showed enhanced growth and yield under nutrient deprivation. In field trials, an increase of yield and straw weight was observed. While the quality of grains, as determined by starch and protein content, was not affected, the yield increased by up to 20%. Our results demonstrate the value of Pseudomonas sp. DSMZ 13134 in agronomical applications for barley.
Introduction
Pathogen infection and abiotic stress have negative influences on the productivity of crop plants in agriculture and result in a high level of dependence on fertilizers, fungicides, and other plant protective agents. However, the negative side-effects of agro-chemicals (for example, contamination of ground water, suppression of beneficial insect populations, selection of resistant strains, and health hazards for humans) have driven farmers to seek alternative means of pest control (Debrewer et al. Citation2008; Costello et al. Citation2009; Landgren et al. Citation2009). The use of rhizosphere bacteria [plant growth-promoting bacteria, PGPB (Bashan and Holguin Citation1998)] organically improves crop yield and plant health. PGPB have been used in agriculture for many years and are considered increasingly relevant alternatives to pesticides and fertilizers (Lucy et al. Citation2004) because they are nontoxic, target the roots of the crop plant, and multiply and decompose following the growth of the host plant, therefore causing less environmental damage (Berg Citation2009).
PGPB strains of the genera Pseudomonas, Azospirillum, Burkholderia, Bacillus, Serratia, and Erwinia are able to colonize plant roots and exert beneficial effects on plant proliferation and fitness by biofertilization, phytostimulation, biocontrol, and stress mitigation or a combination of those traits. Some bacterial inoculants are able to supply nutrients that are otherwise unavailable to the plant, e.g. by solubilization and mobilization of phosphate salts, by mineralizing organic phosphorus, by improving root growth and enhanced uptake of minerals and water or by fixing dinitrogen (Steenhoudt and Vanderleyden Citation2000; Rodriguez et al. Citation2006; Bashan and De-Bashan Citation2010). Phytostimulators are bacteria that modulate plant growth and development by producing plant hormones, e.g. auxins, cytokinins and gibberellins (Ortiz-Castro et al. Citation2009). Another important factor influenced by PGPB is the resistance against pathogens. Rhizosphere bacteria can directly control pathogens by competitive root colonization or by the production of antimicrobial compounds (Compant et al. Citation2005). They also have indirect effects by stimulating the plant's defense system. The latter can be achieved by the mechanism of induced systemic resistance (ISR) or the induction of systemic tolerance (Ryu et al. Citation2007; Van der Ent et al. Citation2009; Wang et al. Citation2009).
Proradix® is a PGPB-containing product sold in Europe (Sourcon-Padena GmbH & Co. KG, Tübingen, Germany) that contains the naturally occurring Pseudomonas sp. strain DSMZ 13134 as its biologically active component. This strain is thought to colonize the roots of treated plants and stimulate the plant's defense system and growth, resulting in healthier and stronger plants and often increased crop yield (www.sourcon-padena.de). Pseudomonas sp. DSMZ 13134 is applied to a wide range of plants, including potatoes, lettuce, tomato, cucumber, zucchini, and grass. In Arabidopsis thaliana, an ethanolic extract from Pseudomonas sp. DSMZ 13134 cultures induces the expression of genes of the systemic acquired resistance pathway (SAR) as well the jasmonic acid pathway (von Rad et al. Citation2005).
Efficient colonization of barley roots by Pseudomonas sp. DSMZ 13134 and its transient impact on the dominant root-associated bacterial community has been shown (Buddrus-Schiemann et al. Citation2010). However, there have been no investigations into the effect of root colonization of Pseudomonas sp. DSMZ 13134 on cereals. In many crops, root colonization by pseudomonads leads to changes in plant growth and/or pathogen resistance. Therefore the present study focuses on agronomically important effects of Pseudomonas sp. DSMZ 13134 on barley. The hypothesis was tested that the inoculation of Pseudomonas sp. DSMZ 13134 promotes the growth of barley and induces resistance against important fungal pathogens. Experiments were carried out in the laboratory, in the greenhouse, and in the field.
Materials and Methods
Bacterial strain and growth conditions
Pseudomonas sp. DSMZ 13134 was obtained from Sourcon-Padena GmbH & Co. KG (Tübingen, Germany). Bacteria were grown either on plates containing King's B agar or in liquid King's B medium (King et al. Citation1954). For inoculation of plants, Pseudomonas sp. DSMZ 13134 was grown overnight in 50 ml King's B medium at 30°C. Bacteria were harvested by centrifugation at 1000g and washed at least three times with sterile water. The optical density of the cell suspension was determined at 435 nm, with an OD of 0.8 equaling 108 cells/ml.
Analysis of physiological properties of Pseudomonas sp. DSMZ 13134
Phosphate solubilizing activity was assayed according to Mehta and Nautiyal (2001). Pseudomonas sp. DSMZ 13134 was grown in NBRIP-BPB medium. Medium pH and absorption at 600nm was measured throughout the experiment. For experiments on plates, Pseudomonas sp. DSMZ 13134 was grown on NBRIP medium supplemented with 1.5% Ca3(PO4)2. Halo formation around colonies was evaluated after 14 days of growth.
Production of siderophores was assayed according to Schwyn and Neilands (1987). Bacteria were grown on CAS plates which contain the blue iron-HDTMA complex. Siderophores remove iron from the complex leading to a color change from blue to red.
Plants, plant growth conditions, and inoculation
For experiments in axenic culture, barley seeds (cultivar Barke; Saatzucht Josef Breun GdbR, Herzogenaurach, Germany) were washed three times for 2 minutes in 70% ethanol and once for 20 minutes in 14% sodium hypochlorite solution. Seeds were incubated at 4°C in 1× antibiotic antimycotic solution (Sigma-Aldrich, Taufkirchen, Germany) for 3 days. Sterilized seeds were transferred to plates containing 50% Murashige and Skoog (MS) (Murashige and Skoog Citation1962) medium and sucrose (10 g/l) and grown for 5 days at 16°C in a phytochamber. Contaminated plates were discarded, and only the seedlings from sterile plates were transferred into sterile Vitro Vent containers (Duchefa, Haarlem, Netherlands) containing 150 g quartz sand and 50 ml 50% MS medium without sucrose. Each Vitro Vent container housed 15 plants. Plants were grown in the Vitro Vent containers for a maximum of 6 days. Liquid levels in the closed containers were observed daily and did not show significant changes during the experiment.
For non-sterile growth in phytochambers, barley (cultivar Barke and Alexis; Saatzucht Josef Breun GdbR, Herzogenaurach, Germany) was grown in pot soil (Einheitserde Typ T, Gebrüder Patzer GmbH & Co. KG, Sinntal-Jossa, Germany: pH 5.8, 310 mg/l N, 300 mg/l P2O5 and 420 mg/l K2O) containing 25% perlite (Knauf, Germany) with a photoperiod of 16 hours, 70% humidity, and a 16°C/12°C day/night cycle. For experiments with reduced nutrient content, pot soil without nutrient supplements was used (Einheitserde Typ 0, pH 5.8, 50 mg/l N, 80 mg/l P2O5 and 80 mg/l K2O).
In the greenhouse, barley was grown in 6-liter pots containing pot soil with 25% per liter. The growth conditions consisted of temperatures around 16°C during day and 12°C at night, a photoperiod of 12 hours and 50–70% humidity. Five plants were grown per pot, and eight replicate experiments were performed.
For inoculation of sterile barley seedlings with Pseudomonas sp. DSMZ 13134, seedling roots were dipped into a bacterial suspension (108 cells/ml) before being transferred into sterile phytotrays. For greenhouse and field experiments, barley seeds were inoculated with the commercial product Proradix®, which contains the strain Pseudomonas sp. DSMZ 13134, using vacuum infiltration (Puente and Bashan Citation1993). Treatment was conducted by the producer Sourcon-Padena GmbH & Co. KG. Briefly, a nutrient free suspension containing 8×1010 CFU/kg seeds was sprayed onto unsterile seeds, and the vacuum was applied. Thereafter, the seeds were dried at 27°C for 4 hours.
Field experiments
Field experiments were performed in 2006 and 2007 at the experimental station of the Helmholtz Zentrum Muenchen in Scheyern (http://www.helmholtz-muenchen.de/scheyern). Barley cv. Barke was grown on plots with a plot size of 10×1.5 m. In each year, two randomized experiments under conventional (C1, C2) and two randomized experiments under organic (E1, E2) agricultural conditions were performed. Each experiment contained four untreated and four Proradix® (Pseudomonas sp. DSMZ 13134)-treated plots. Conventional experiments were conducted under good agricultural practice (BMELV Citation2010) with application of fertilizer and pesticides as deemed necessary, whereas in organic experiments, no fertilizer or pesticide was used. Harvested barley grains were analyzed for thousand grain weight (tgw), protein and starch content at Agrolab (Bruckberg, Germany).
Infection experiments with Rhynchosporium secalis and Gaeumannomyces graminis
Eighteen-day-old barley cv. Alexis plants grown in pots were inoculated with Pseudomonas sp. DSMZ 13134 by soil drench at 107 cfu/g soil in water. Control plants were inoculated with water only. Four days after inoculation, plants were infected with R. secalis. Rhynchosporium spore suspensions were scraped from 14-day-old cultures grown on lima bean agar (Difco Laboratories, Detroit, Michigan, USA) into distilled water containing 500 µg/ml of Tween 20 and filtered through muslin. The spore suspensions were diluted to concentrations of 2×105 spores/ml. Plants were sprayed uniformly with inoculum and left for 20 minutes to dry. Inoculated plants were then lightly sprayed with water and kept for 48–72 hours in a dark moist chamber at 18°C and then transferred onto glasshouse benches at 16–18°C (Pickering et al. Citation2006). Samples were collected one week and two weeks after infection. For each time point, the leaves of at least three replicate samples consisting of four plants each were harvested.
For infections with G. graminis, axenic barley cultures were used. Five-day-old sterile barley cv. Barke seedlings were inoculated with Pseudomonas sp. DSMZ 13134 by dipping the roots into a bacterial suspension (108 cells/ml water) and maintained overnight in sterile phytotrays (Duchefa, Haarlem, Netherlands) containing 200 g sand and 50 ml 50% MS medium without sucrose. Control plants were inoculated with water only. After 24 hours, each seedling was transferred together with a piece of G. graminis mycelium into autoclaved glass cylinders (30×15 cm) containing 400 g sterile sand and 50 ml sterile filtered 50% MS medium without sucrose. Samples were collected 8 days and 16 days after infection. For each time point, the roots from three replicate cylinders containing five plants each were harvested. All infection experiments were repeated at least three times.
Quantitative real-time PCR
Quantification of infections was performed using quantitative real-time PCR (qPCR). Total genomic DNA was extracted from the harvested plant samples with the DNAeasy Plant DNA Extraction Kit (Qiagen, Hilden, Germany). The amount of pathogen DNA in the extracted DNA samples was analyzed by absolute quantification using AbsoluteTM QPCR SYBR® Green Low Rox Mix (ABgene, Hamburg, Germany) and an Applied Biosystems (Darmstadt, Germany) 7500 system. For analysis of R. secalis-infected samples, the primers RS8mod2 (CCAGAGGACCACAACTCTTGTT) and RS9mod2 (AAGGCGCAATGTGCGTTCAAA) were used to amplify a fragment from the 5.8S RNA gene of R. secalis (Lee et al. Citation2001). For analysis of G. graminis-infected samples, the primers AV3 (TGCTCATGGTGGTTCCTGCG) and Ggt_for1 (GGTCAGGACAAGGACTACCC) were used to amplify a fragment from the Avenacinase-like gene of G. graminis (Rachdawong et al. Citation2002). Each 96-well PCR plate contained the standard curve samples, non-template control and samples to be analyzed in threefold replicates. Standard curves for absolute quantification were generated by serial dilution of pathogen genomic DNA ranging from 20 ng to 2 pg per well. PCR conditions were chosen according to the manufacturer's instructions, with a combined 1 minute annealing and amplification step at 60°C.
Statistics
In infection experiments, data represent the means and standard deviation (SD) of at least three biological replicates containing at least four plants each. In greenhouse experiments, data represent the means and SD of eight biological replicates containing five plants each. In the field trials, data represent the means and SD of four plots of the size of 10×1.5 m each. Statistical significance was determined using a t-test (p<0.05).
Results
In this work, we elucidated the value of the strain Pseudomonas sp. DSMZ 13134 for agricultural application to the crop plant barley. Controlled conditions in laboratory and greenhouse experiments allowed analysis of the defined beneficial effects of this strain, namely, induction of resistance toward pathogens and growth promotion. In field trials, we tested the influence of Pseudomonas sp. DSMZ 13134 on barley yield under conventional and organic conditions.
Physiological properties of Pseudomonas sp. DSMZ 13134
Analysis of the phosphate solubilizing ability of Pseudomonas sp. DSMZ 13134 was done in liquid medium as well as on plate. Solubilization of insoluble phosphate compounds is often based on the acidification of the surrounding medium by the bacteria. In Ca3(PO4)2 –containing NBRIP-BPB medium, this leads to destaining of bromophenolblue. Growth of Pseudomonas sp. DSMZ 13134 in NBRIP-BPB medium showed a distinct decrease of the medium pH from 6.7 to 3.8 as well as destaining of the medium. Growth of Pseudomonas sp. DSMZ 13134 on plates containing insoluble Ca3(PO4)2 produced a clear halo with an average radius of 0.6 cm around the colony while E. coli controls did not show solubilization of Ca3(PO4)2 ().
Figure 1. Analysis of physiological properties of Pseudomonas sp. DSMZ 13134: Note: Assays for pH drop and destaining of bromophenol (A), solubilization of insoluble phosphates (B), and siderophore production (C) were conducted as described by Mehta and Nautiyal (Citation2001) and Schwyn and Neilands (Citation1987).
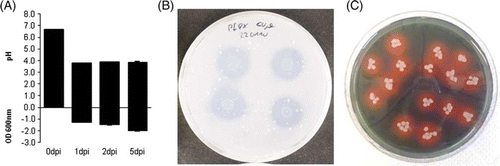
On CAS plates, Pseudomonas sp. DSMZ 13134 produced clear orange-red halos around their colonies (). Therefore, this strain is able to produce siderophores. E. coli was grown on separate CAS plates as a control and did not induce any color change on CAS medium.
Influence of Pseudomonas sp. DSMZ 13134 treatment on the resistance of barley against pathogens
One of the most commercially attractive effects of beneficial rhizosphere bacteria is the ability of some strains to increase the plant's resistance to pathogens. To elucidate the effect of Pseudomonas sp. DSMZ 13134 on pathogen resistance, treated barley plants were infected with R. secalis, a major barley leaf pathogen, and G. graminis, a pathogen infecting the root crown. Plants used for the infection with R. secalis were grown in soil. Differences in pathogen growth could be observed 14 days post infection (dpi) using qPCR. Pseudomonas sp. DSMZ 13134-treated plants showed a nearly 40% reduction in pathogen content compared to mock-treated plants (). Infection experiments with G. graminis were performed in axenic cultures. Inoculated plants showed significantly reduced pathogen growth at both sampling time points. Pathogen growth was reduced by almost 20% at 8 dpi and by almost 60% at 15 dpi compared to mock-treated plants. Visible symptoms caused by the pathogen on the root crown were also reduced ().
Figure 2. Infection of barley with Rhynchosporium secalis. Barley seedlings were inoculated with Pseudomonas sp. DSMZ 13134 (black columns) or mock treated (white columns) and additionally infected with Rhynchosporium secalis. Analysis was done by qRT-PCR at 7 and 14 dpi. Data represent the means and SD of three biological replicates containing four plants. Asterisk (*) denotes statistically significant; t-test; p<0.05; dpi = day post inoculation with the pathogen.
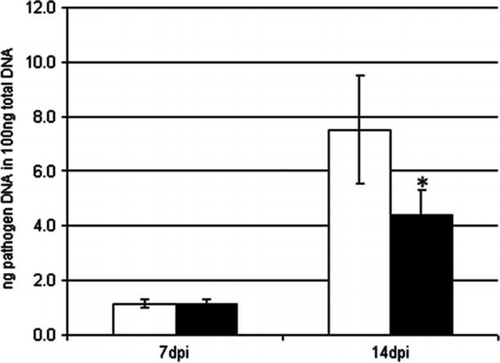
Figure 3. Infection of barley with Gaeumannomyces graminis. Infected roots of (A) Pseudomonas sp. DSMZ 13134-treated and (B) mock-treated barley plants. (C) Pseudomonas sp. DSMZ 13134-treated (black columns) or mock-treated (white columns) were infected with G. graminis. Analysis was done by qRT-PCR 8 dpi and 15 dpi. Data represent the means and SD of three biological replicates containing five plants each. Asterisk (*) denotes statistically significant; t-test; p<0.05; dpi = day post inoculation with the pathogen.
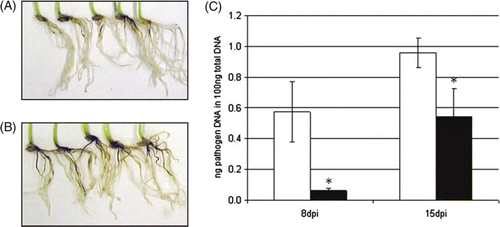
Effect of Pseudomonas sp. DSMZ 13134 on barley growth
Many rhizosphere bacteria influence the growth of their host plants and serve as PGPB. To evaluate the effect of Pseudomonas sp. DSMZ 13134 application on the growth of barley, inoculation experiments were conducted in three different experimental setups: (1) in sterile barley culture, (2) under controlled greenhouse conditions, and (3) in the field. Experiments in sterile culture allowed the analysis of inoculated barley seedlings up to 11 days. Within this time, inoculation of seedlings with Pseudomonas sp. DSMZ 13134 suspensions of densities of up to 108 cells/ml did not influence root morphology (data not shown).
In the greenhouse studies, potted barley plants were grown to full ripening. After harvest, the straw weight, thousand grain weight, the number of ears, and total yield were determined. Pseudomonas sp. DSMZ 13134-treated plants grown in pot soil containing an ample amount of nutrients did not show differences in the observed growth parameters compared to untreated control plants. However, plants grown on pot soil without nutrient supplements showed a pronounced difference between sp. DSMZ 13134-treated and control plants; Pseudomonas sp. DSMZ 13134-treated plants showed higher straw weight (29%), an increased number of ears (24%), and an increase in yield (16%) ().
Table 1. Determination of growth parameters under nutrient deprivation.
In 2006 and 2007, Pseudomonas sp. DSMZ 13134-treated barley plants were investigated in field trials at the experimental station in Scheyern, Germany (). In 2006, statistically significant increases in yield in one of the conventional (C2) and one of the organic (E1) experiments were observed (15% and 20%, respectively). Experiments C1 and E2 also showed an increase in yield, but the increase was not statistically significant ().
Table 2. Analysis of field trials.
In 2007, experiments were repeated at the same locations in the experimental station, although not exactly at the same position to prevent possible carryover of inoculant from the previous year's experiments. Only one of the conventional experiments (C1) showed an increase in yield (13%). The experiments were analyzed for additional agriculturally important traits in 2007. A significantly higher straw weight and thousand grain weight were also detected in the experiment C1 (2007), which produced the significant increase in yield. A change in starch and protein content per unit dry weight was not observed in harvested seeds after Pseudomonas sp. DSMZ 13134 application in any experiment ().
Discussion
Root-colonizing bacteria that exert beneficial effects on plant development via direct or indirect mechanisms have become increasingly utilized in agriculture. In this study, the influence of the commercially available Pseudomonas strain DSMZ 13134 (Proradix®) on the growth and resistance of the crop plant barley was analyzed under laboratory, greenhouse, and field conditions. Thus far, Pseudomonas sp. DSMZ 13134 has only been used in agriculture for dicotyledonous crops, such as potato, lettuce, and tomato (www.sourcon-padena.de). After sowing, Pseudomonas sp. DSMZ 13134 colonizes the plant root and leads to beneficial effects such as an increased yield and resistance toward pathogens. Colonization of the plant root by the inoculum is a crucial prerequisite for any growth promoting effect. The successful colonization of barley roots by Pseudomonas sp. DSMZ 13134 has previously been shown in detail. Using FISH techniques and a gfp-tagged strain, Pseudomonas sp. DSMZ 13134 has been shown to be localized on the root surface from the root base up to the tip, with higher cell densities in the root hair zone (Buddrus-Schiemann et al. Citation2010).
Induction of resistance against fungal pathogens
In laboratory experiments, we tested the resistance of Pseudomonas sp. DSMZ 13134-treated barley against the leaf pathogen R. secalis and the root pathogen G. graminis. Both pathogens can cause severe yield losses and are economically important barley diseases in Europe, North America, and Australia (Weller et al. Citation2007; Zaffarano et al. Citation2009). The inoculation of barley roots with Pseudomonas sp. DSMZ 13134 significantly reduced both R. secalis and G. graminis infections. Soil-borne pathogens can be suppressed by PGPB directly or indirectly. While effective colonization of the plant root alone is not sufficient for biocontrol of pathogens (Kamilova et al. Citation2005), pathogen growth is inhibited or slowed by the production of antibacterial and antifungal secondary metabolites (antibiosis), competition for nutrients and niches or signal interference (Lugtenberg and Kamilova Citation2009). Pseudomonas sp. DSMZ 13134 also produces siderophores in vitro. Although bacterial siderophore production and efficiency are highly dependent on various environmental conditions in vivo (Crowley and Kraemer Citation2007), their presence might play an important role in the suppression of pathogens in the rhizosphere of the plant because bacterial siderophores possess a higher affinity for iron than fungal siderophores. This leads to the deprivation of this essential element in the fungus and can cause fungal growth inhibition (Compant et al. Citation2005).
In contrast to the direct interaction between PGPB and pathogens, the inhibition of leaf pathogens by rhizosphere bacteria is thought to mainly occur indirectly through a priming effect called ISR. This mechanism puts plants into an alert state that allows them to respond faster and more strongly to pathogen attack. A hallmark of ISR is that inoculation of the roots with rhizobacteria alone does not directly induce defense genes in plant leaves. Therefore, no marker genes for the induction of ISR are known to date (Wang et al. Citation2005; Van Wees et al. Citation2008). Using a dedicated barley DNA microarray containing 1000 ESTs focusing on pathogen defense, we could not detect a statistically significant induction of defense genes in barley leaves after root-inoculation with Pseudomonas sp. DSMZ 13134 (data not shown), which is an indication that the induction of resistance against leaf pathogens by Pseudomonas sp. DSMZ 13134 is likely not a direct effect.
Influence of Pseudomonas sp. DSMZ 13134 on growth and yield of barley
In short-term axenic in vitro experiments, we observed colonization of barley roots by Pseudomonas sp. DSMZ 13134 (Buddrus-Schiemann et al. Citation2010), but no changes in root and shoot growth or morphology (data not shown). To investigate the effect of Pseudomonas sp. DSMZ 13134 on barley growth during a whole vegetation period, we conducted greenhouse experiments under controlled climatic conditions. Interestingly, we observed a clear beneficial effect of Pseudomonas sp. DSMZ 13134 on barley growth under nutrient limitation. Compared to control plants, the inoculated plants showed an increase in straw weight, a higher number of ears and, therefore, a higher yield. When a surplus of nutrients was available, no beneficial effect of Pseudomonas sp. DSMZ 13134 application on yield was observed, a phenomenon which is also very common for inoculation with other bacteria like Azospirillum (Bashan and de-Bashan 2010). Rhizosphere bacteria influence the growth of plants in many different ways, which are often strain specific. While some strains act on certain plant species only, other bacteria, like Azospirillum, act completely non-specific and affect many different plant species. The primary mechanisms by which the bacteria affect growth are the mineralization of organic phosphates, the solubilization of insoluble inorganic phosphates by the release of organic acids (Rodriguez et al. Citation2006; Vassilev et al. Citation2006), and the iron uptake that many rhizosphere bacteria exert through the production of siderophores. Pseudomonas sp. DSMZ 13134 also causes a drop of environmental pH, solubilizes insoluble phosphates, and produces siderophores in vitro. These properties could improve the plant's nutrient supply under nutrient-poor growth conditions.
Field experiments using rhizosphere bacteria have been performed throughout the world using different combinations of crops and bacteria (Dobbelaere et al. Citation2001; Bashan et al. Citation2004; Karthiba et al. Citation2010). Multiple effects on the growth of crops have been observed, including increased growth, yield, and quality (Somers et al. Citation2004). Using Pseudomonas fluorescens biovar I (Q72a-80), an increase of grain yield of winter wheat up to 26% after seed treatment was found in field studies (Weller and Cook Citation1986). In another study with different rice cultivars, yield increases due to inoculation with Pseudomonas fluorescens strains varied from 3% to 160% (Sakthivel and Gnanamanickam Citation1987). To determine the reproducibility and consistency of the Pseudomonas sp. DSMZ 13134 effects on barley, our field experiments were conducted at the experimental station in Scheyern (Germany) over 2 years (2006 and 2007) using conventional or organic cultivation methods. In general, Pseudomonas sp. DSMZ 13134 increased barley yield but had no effect on grain quality. In 2006, all experiments showed an increase in yield, while in 2007, only one of four experiments resulted in higher yield. Many complex, interacting factors may influence the beneficial effects of treatments with PGPB (Kloepper Citation1996; Haas and Defago Citation2005). In our field experiments, the growth of barley plants and the survival of Pseudomonas sp. DSMZ 13134 on the root surface were probably severely affected by climatic conditions. The year 2007 was marked by higher-than-average temperatures and very dry conditions in the first half of the vegetation period (Appendix ), resulting in reduced yield in all experiments (up to 50%) compared to 2006. It has been shown that the humidity within the soil influences the growth of rhizosphere bacteria both positively and negatively. Sorghum bicolor inoculated with Azospirillum is able to utilize water from the soil more efficiently than control plants, reducing the adverse effects resulting from drought (Sarig and Okon Citation1992). However, drought conditions and excessive water can also inhibit PGPB root colonization (de Freitas and Germida Citation1992; Lucy et al. Citation2004), which would counteract the beneficial effects induced by PGPB. Other authors have reported the negative impact of temperature on the survival of PGPB inocula in soil (Seong et al. Citation1991; Vandenhove et al. Citation1991). Thus, the extraordinarily dry conditions in 2007 compared to 2006 could be one of the reasons why there was a reduced overall yield and a less pronounced difference between Pseudomonas sp. DSMZ 13134-treated plots and untreated control plots in 2007.
Most of the known positive effects of PGPB (e.g. increase of nutrient uptake, ISR, antibiosis) should mainly benefit plants grown under organic conditions, where the use of fertilizers and pesticides is severely restricted. Therefore, when conducting our field trials, we expected a stronger effect of Pseudomonas sp. DSMZ 13134 under organic growth conditions than conventional cultivation. However, we did not observe a correlation between the type of cultivation and growth promotion. Growth promotion even under technically supported growth conditions is a strong asset for this PGPB product.
Conclusions
Taken together, our results demonstrate the usefulness of Pseudomonas sp. DSMZ 13134 for agricultural applications in barley production. As in many cases of PGPB treatments, the net yield increases in field experiments varied by year and field. Suboptimal environmental conditions can also hamper the colonization, survival and beneficial effects of Pseudomonas sp. DSMZ 13134. Its ability to inhibit infection by both leaf and root pathogens makes it useful in agriculture, especially in organic cultivation, where the use of pesticides is restricted. Depending on the situation, single or combined effects may lead to beneficial results. The results of this study support further work in elucidating the mechanisms of Pseudomonas sp. DSMZ 13134 plant growth promotion. Analyses using commercial barley DNA-microarrays and barley RNAi lines will give detailed insights into the molecular mechanisms of induced resistance and plant growth promotion for this plant–PGPB interaction.
Acknowledgements
We thank Georg Gerl for his extraordinary help and insightful advice in performing the field experiments and Dr. Günther Schweizer (LfL Bayern) for the Rhynchosporium secalis infections. Furthermore, we are grateful to Sourcon-Padena GmbH & Co. KG (Dr. Wolfgang Vogt, Karin Mai) for providing Proradix® and for performing the inoculation of the seeds by vacuum infiltration.
Additional information
Notes on contributors
A. Fröhlich†
†These authors contributed equally to this studyK. Buddrus-Schiemann†
†These authors contributed equally to this studyReferences
- Bashan , Y and De-Bashan , LE . 2010 . How the plant growth-promoting bacterium Azospirillum promotes plant growth-a critical assessment . Adv Agro. , 108 : 77 – 136 .
- Bashan , Y and Holguin , G . 1998 . Proposal for the division of plant growth-promoting rhizobacteria into two classifications: biocontrol-PGPB (plant growth-promoting bacteria) and PGPB . Soil Biol Biochem. , 30 : 1225 – 1228 .
- Bashan , Y , Holguin , G and de-Bashan , LE . 2004 . Azospirillum-plant relationships: physiological, molecular, agricultural, and environmental advances (1997–2003) . Can J Microbiol. , 50 : 521 – 577 .
- Berg , G . 2009 . Plant-microbe interactions promoting plant growth and health: perspectives for controlled use of microorganisms in agriculture . Appl Microbiol Biotechnol. , 84 : 11 – 18 .
- BMELV 2010 Gute fachliche Praxis im Pflanzenschutz: Grundsätze für die Durchführung [Basic guidelines for good agricultural practice in crop protection] . Ed. L u V B Bundesministerium für Ernährung. German Ministry for Nutrition and Agriculture. .
- Buddrus-Schiemann , K , Schmid , M , Schreiner , K , Welzl , G and Hartmann , A . 2010 . Root colonization by Pseudomonas sp. DSMZ 13134 and impact on the indigenous rhizosphere bacterial community of barley . Microb Ecol. , 60 : 381 – 393 .
- Compant , S , Duffy , B , Nowak , J , Clement , C and Barka , EA . 2005 . Use of plant growth-promoting bacteria for biocontrol of plant diseases: principles, mechanisms of action, and future prospects . Appl Environ Microbiol. , 71 : 4951 – 4959 .
- Costello , S , Cockburn , M , Bronstein , J , Zhang , X and Ritz , B . 2009 . Parkinson's disease and residential exposure to maneb and paraquat from agricultural applications in the central valley of California . Am J Epidemiol. , 169 : 919 – 926 .
- Crowley , DEKraemer SM and Kraemer , SM . 2007 . “ Function of siderophores in the plant rhizosphere ” . In The rhizosphere: biochemistry and organic substances at the soil-plant interface , Edited by: Varanini , Z , Pinton , R and Nannipieri , P . 616 USA : CRC Press .
- Debrewer , LM , Ator , SW and Denver , JM . 2008 . Temporal trends in nitrate and selected pesticides in mid-atlantic ground water . J Environ Qual. , 37 : S296 – S308 .
- de Freitas , J and Germida , JJ . 1992 . Growth promotion of winter wheat by fluorescent pseudomomads under field conditions . Soil biol biochem. , 24 : 1137 – 1146 .
- Dobbelaere , S , Croonenborghs , A , Thys , A , Ptacek , D , Vanderleyden , J , Dutto , P , Labandera-Gonzalez , C , Caballero-Mellado , J , Aguirre , JF Kapulnik , Y . 2001 . Responses of agronomically important crops to inoculation with Azospirillum . Aust J Plant Physiol. , 28 : 871 – 879 .
- Haas , D and Defago , G . 2005 . Biological control of soil-borne pathogens by fluorescent pseudomonads . Nat Rev Microbiol. , 3 : 307 – 319 .
- Kamilova , F , Validov , S , Azarova , T , Mulders , I and Lugtenberg , B . 2005 . Enrichment for enhanced competitive plant root tip colonizers selects for a new class of biocontrol bacteria . Environ Microbiol. , 7 : 1809 – 1817 .
- Karthiba , L , Saveetha , K , Suresh , S , Raguchander , T , Saravanakumar , D and Samiyappan , R . 2010 . PGPR and entomopathogenic fungus bioformulation for the synchronous management of leaffolder pest and sheath blight disease of rice . Pest Manag Sci. , 66 : 555 – 564 .
- King , EO , Ward , MK and Raney , DE . 1954 . Two simple media for the demonstration of phycocyanin and fluorescin . J Lab Clin Med. , 44 : 301 – 307 .
- Kloepper , JW . 1996 . Host specificity in microbe-microbe interactions-biological control agents vary in specificity for hosts, pathogen control, ecological habitat and environmental conditions . Bioscience. , 46 : 406 – 409 .
- Landgren , O , Kyle , RA , Hoppin , JA , Beane Freeman , LE , Cerhan , JR , Katzmann , JA , Rajkumar , SV and Alavanja , MC . 2009 . Pesticide exposure and risk of monoclonal gammopathy of undetermined significance in the agricultural health study . Blood , 113 : 6386 – 6391 .
- Lee , H , Tewari , J and Turkington , T . 2001 . A PCR-based assay to detect Rhynchosporium secalis in barley seed . Plant Disease. , 85 : 220 – 225 .
- Lucy , M , Reed , E and Glick , BR . 2004 . Applications of free living plant growth-promoting rhizobacteria . Antonie Van Leeuwenhoek. , 86 : 1 – 25 .
- Lugtenberg , B and Kamilova , F . 2009 . Plant-growth-promoting rhizobacteria . Annu Rev Microbiol. , 63 : 541 – 556 .
- Mehta , S and Nautiyal , CS . 2001 . An efficient method for qualitative screening of phosphate-solubilizing bacteria . Curr Microbiol. , 43 : 51 – 56 .
- Murashige , T and Skoog , F . 1962 . A revised medium for rapid growth and bioassays with tobacco tissue cultures . Physiol Plant. , 15 : 473 – 497 .
- Ortiz-Castro , R , Contreras-Cornejo , HA , Macias-Rodriguez , L and Lopez-Bucio , J . 2009 . The role of microbial signals in plant growth and development . Plant Signal Behav. , 4 : 701 – 712 .
- Pickering , R , Ruge-Wehling , B , Johnston , P , Schweizer , G , Ackermann , P and Wehling , P . 2006 . The transfer of a gene conferring resistance to scald (Rhynchosporium secalis) from Hordeum bulbosum into H. vulgare chromosome 4HS . Plant Breed. , 125 : 576 – 579 .
- Puente , M-E and Bashan , Y . 1993 . Effect of inoculation with Azospirillum brasilense strains on the germination and seedlings growth of the giant columnar cardon cactus (Pachycereus pringlei) . Symbiosis. , 15 : 49 – 60 .
- Rachdawong , S , Cramer , C , Grabau , E , Stromberg , V , Lacy , G and Stromberg , E . 2002 . Gaeumannomyces graminis vars. avenae, graminis, and tritici identified using PCR amplification of avenacinase-like genes . Plant Disease. , 86 : 652 – 660 .
- Rodriguez , H , Fraga , R , Gonzalez , T and Bashan , Y . 2006 . Genetics of phosphate solubilization and its potential applications for improving plant growth-promoting bacteria . Plant Soil. , 287 : 15 – 21 .
- Ryu , CM , Murphy , JF , Reddy , MS and Kloepper , JW . 2007 . A two-strain mixture of rhizobacteria elicits induction of systemic resistance against Pseudomonas syringae and cucumber mosaic virus coupled to promotion of plant growth on Arabidopsis thaliana . J Microbiol Biotechnol. , 17 : 280 – 286 .
- Sakthivel , N and Gnanamanickam , SS . 1987 . Evaluation of Pseudomonas fluorescens for suppression of sheath rot disease and for enhancement of grain yields in rice (Oryza sativa L.) . Appl Environ Microbiol. , 53 : 2056 – 2059 .
- Sarig , S and Okon , Y . 1992 . Effect of Azospirillum brasilense inoculation on growth dynamics and hydraulic conductivity of Sorghum bicolor roots . J Plant Nut. , 15 ( 6&7 ) : 805 – 819 .
- Schwyn , B and Neilands , JB . 1987 . Universal chemical assay for the detection and determination of siderophores . Anal Biochem. , 160 : 47 – 56 .
- Seong , K , Höfte , M , Boelens , J and Verstraete , W . 1991 . Growth, survival and root colonization of plant growth beneficial Pseudomonas fluorescens ANP15 and Pseudomonas aeruginosa 7NSK2 at different temperatures . Soil Bio Biochem. , 23 : 423 – 428 .
- Somers , E , Vanderleyden , J and Srinivasan , M . 2004 . Rhizosphere bacterial signalling: a love parade beneath our feet . Crit Rev Microbiol. , 30 : 205 – 240 .
- Steenhoudt , O and Vanderleyden , J . 2000 . Azospirillum, a free-living nitrogen-fixing bacterium closely associated with grasses: genetic, biochemical and ecological aspects . FEMS Microbiol Rev. , 24 : 487 – 506 .
- Vandenhove , H , Merckx , R , Wilmots , H and Vlassak , K . 1991 . Survival of Pseudomonas fluorescens inocula of different physiological stages in soil . Soil Biol Biochem. , 23 : 1133 – 1142 .
- Van der Ent , S , Van Wees , SC and Pieterse , CM . 2009 . Jasmonate signaling in plant interactions with resistance-inducing beneficial microbes . Phytochemistry. , 70 : 1581 – 1588 .
- Van Wees , SC , Van der Ent , S and Pieterse , CM . 2008 . Plant immune responses triggered by beneficial microbes . Curr Opin Plant Biol. , 11 : 443 – 448 .
- Vassilev , N , Vassileva , M and Nikolaeva , I . 2006 . Simultaneous P-solubilizing and biocontrol activity of microorganisms: potentials and future trends . Appl Microbiol Biotechnol. , 71 : 137 – 144 .
- von Rad , U , Mueller , MJ and Durner , J . 2005 . Evaluation of natural and synthetic stimulants of plant immunity by microarray technology . New Phytol. , 165 : 191 – 202 .
- Wang , Y , Ohara , Y , Nakayashiki , H , Tosa , Y and Mayama , S . 2005 . Microarray analysis of the gene expression profile induced by the endophytic plant growth-promoting rhizobacteria, Pseudomonas fluorescens FPT9601-T5 in Arabidopsis . Mol Plant Microbe Interact. , 18 : 385 – 396 .
- Wang , S , Wu , H , Qiao , J , Ma , L , Liu , J , Xia , Y and Gao , X . 2009 . Molecular mechanism of plant growth promotion and induced systemic resistance to tobacco mosaic virus by Bacillus spp . J Microbiol Biotechnol. , 19 : 1250 – 1258 .
- Weller , DM and Cook , RJ . 1986 . Increased growth of wheat by seed treatments with fluorescent pseudomonads, and implications of Pythium control . Can J Plant Pathol. , 8 : 328 – 334 .
- Weller , DM , Landa , BB , Mavrodi , OV , Schroeder , KL , De La Fuente , L , Blouin Bankhead , S , Allende Molar , R , Bonsall , RF , Mavrodi , DV and Thomashow , LS . 2007 . Role of 2,4-diacetylphloroglucinol-producing fluorescent Pseudomonas spp. in the defense of plant roots . Plant Biol (Stuttg) , 9 : 4 – 20 .
- Zaffarano , PL , McDonald , BA and Linde , CC . 2009 . Phylogeographical analyses reveal global migration patterns of the barley scald pathogen Rhynchosporium secalis . Mol Ecol. , 18 : 279 – 293 .