Abstract
Ten genotypes of wheat (Triticum aestivum L.) were grown for 20 days in complete nutrient solution with 1 mM (nitrogen-insufficient), 4 mM (nitrogen-moderate) and 10 mM (nitrogen-sufficient) nitrogen (N) levels, and nitrogen efficiency (NE) was analyzed. Of these 10 genotypes, VL829 was identified as the most N-efficient, while HUW234 the most N-inefficient. To find out the physiological basis of this difference, we investigated the possible role of influx across the root cell plasma membrane in conferring NE by measuring the short-term
uptake in selected 10 genotypes. Uptake experiments revealed the presence of two separate nitrate transporter systems mediating high- and low-affinity nitrate uptake. Interestingly, the nitrate uptake by the roots of VL829 is mediated by both high- and low-affinity nitrate transporter systems, while that of HUW234 by only low affinity nitrate transporter system. The study suggests that root
uptake rate may play an important role in conferring N- efficiency of wheat genotypes under N-limiting condition. Also the activities and expression levels of nitrate assimilatory enzymes in N-efficient and N-inefficient wheat genotypes showed that nitrate reductase (NR) and glutamine synthetase (GS) play important roles in N assimilation under low-nitrogen conditions.
Introduction
Globally 50% of human population relies on nitrogen (N) fertilizer for food production. The N fertilizer consumption has grown dramatically in Asia, about 17-fold in the last 40 years (Pathak et al. Citation2008). Only 50% or less of the applied nitrogen is used for the producing of the aboveground biomass of cereals. The other 50% or more gets dissipated in the wider environment by volatilization, leaching, surface runoff, and denitrification (Jeffrey et al. Citation2002) resulting the major detrimental impacts on environment such as eutrophication of fresh water and marine ecosystems (Beman et al. Citation2005) gaseous emission of oxides reacting with the stratospheric ozone and the emission of toxic ammonia (Stulen et al. Citation1998) into the atmosphere. Despite these hazardous impacts on the biosphere the use of N fertilizers 100-fold increase over the last 100 years as it is known that cereal yield and fertilizer N consumption have increased in a linear fashion during the past 40 years and both are highly correlated (Ladha Citation2005).
Nitrogen is an important and desirable agronomic character in wheat (Triticum astivum L.). Under most soil conditions, ammonium fertilizer is rapidly nitrified to nitrate by soil organisms (Edhaie and Waines Citation2001). In the early growth season, nitrate tends to be high in the soil and so it would be desirable to utilize as much of this form as possible. There are clear indications that activation of its absorption by plants is under genetic control (Ahmad et al. Citation2005) and that considerable differences exist among cultivars. The variation is due to differences in the size and morphology of the roots, the demand for mineral elements caused by differences in relative growth rate, uptake and transport and use efficiency (Pathak et al. Citation2008).
So, when an excess of N cannot be totally avoided, it should also be important to search for species or genotypes that are able to absorb and accumulate high concentrations of N or those which can grow and yield well under low N conditions that is N-efficient genotypes (Chandna et al. Citation2010; Hakeem et al. Citation2011) to workout their physiological N uptake kinetics and finally determine the biochemical pathway of the nitrogen absorbed from the soil. This will help us in improvement of N-uptake efficiency which ultimately leads to the increase in the nitrogen use efficiency (NUE) among the crop plants as it has a practical merit because millions of tones of N-fertilizers are applied to the soil to increase crop productivity per unit area. Keeping this as the primary motive some work has been done with reference to wheat genotypes.
Plants have evolved an active, regulated and multiphasic transport system making their nitrate uptake scheme efficient enough to transport sufficient nitrate to satisfy total nitrogen demand of the plant in face of varying external nitrate concentrations. Nitrate is the most abundant form of nitrogen available to the plant roots in aerated soils. Root nitrate uptake is carried out by both high- and low-affinity nitrate transporters that are encoded by a multi-gene family (Forde Citation2002). The uptake involves high- and low-affinity transport systems, also known as HATS and LATS, respectively (Touraine et al. Citation2001). One of the high-affinity systems is strongly induced in presence of and is known as inducible high-affinity transport system (or iHATS), while the second high-affinity system (the cHATS) and LATS are constitutively expressed (Forde Citation2002). The dynamics of nitrogen uptake can be quantitatively described using Michaelis–Menten equation. The equation considers two parameters; V
max and K
m. They are the measures of maximum uptake rate and the affinity of the uptake sites for the nutrient, respectively. Previously Herbert et al. (Citation1995) have also recognized kinetic parameters (K
m and V
max) as important factors that greatly affect the efficiency of nutrient uptake from soil, and suggested that these parameters can be useful to select the genotypes having high nutrient-utilization efficiency.
In the present study, attempts have been made to investigate the Nitrogen efficiency (NE) among 10 different geographically grown wheat genotypes and their genetic variability of N-uptake. Further the biochemical basis of this variability was also analyzed.
Material and methods
Plant culture
Ten wheat (Triticum aestivum L.) genotypes were selected for this study. Two genotypes of wheat VL-421 and HS-277 procured from Vivekananda Parvatiya Krishi Anusandhan Sansthan (VPKAS), Almora, Uttaranchal and seven genotypes of wheat HUW206, HD2270, HD2307, HUW468, UP-262, PBW-65, PBW-154, and 3077Raj from Indian Agricultural Research Institute, New Delhi (plain area) of India.
The seeds were germinated in moist petri plates, after surface sterilization with 0.2% mercuric chloride. The seedlings were grown with 0.5 strength nutrient solution (Hirel et al. Citation2001) containing three different levels of KNO3 1 mM (T1), 4 mM (T2), and 10 mM (T3). Potassium was balanced as per the concentration of the nutrient solution so that only the effect of Nitrate would be measured. The plants were grown for 20 days in the growth chamber, maintained at day/night temperature (28°C/22°C), relative humidity (75%), and photoperiod of 16/8 hours (280–300 mmol m−2 s−1). The nutrient solution was continuously bubbled with sterile air and changed every fourth day.
Growth parameter and NE
For determination of fresh weight, the plants were taken out of nutrient solution. Shoot were separated from roots and weighed. For determination of dry weight, plant samples were dried at 65°C for 72 hours and weighed.
Nitrogen efficiency of 10 wheat varieties was measured by applying the following formula. The experiment was conducted in three replicates. NE was calculated as follows according to Moll et al. (Citation1982).
Measurement of Km
Uptake of NO3 into roots of intact seedlings was measured in 10-day-old seedlings grown in nutrient solution without N. Ten-day-old seedlings were removed from the nutrient solution and their roots were rinsed with deionized water and then held for two minutes in the air. Each part of the root system was then placed in 100 ml of 10 mM 2-morpholinoethan sulphonic acid-KOH (MES-KOH) buffer (pH 5.8) and nitrate uptake was measured at 3 mM external concentrations. Plants were incubated for 12 hour. The uptake rate was measured at every 2 hour intervals. The solution was aerated by continuous shaking to provide ample O2 and maintain the solution concentration at the root surface. Temperature and relative humidity of the chamber were maintained at 28°C and 75%, respectively. Uptake rate was determined by the disappearance of nitrate from the external solution, which represents net flux into the root. The uptake rate was determined in three replicates for every time interval.
Nitrate was measured by high-pressure liquid chromatography (HPLC) using a 10 mm×250 mm analytical anion exchange column (Whatman Partisil-10 SAX) according to Hunt and Seymour (Citation1985). UV detector was used to detect the ions at 210 nm. Eluent was sodium phosphate buffer (50 mM/pH 3) and flow rate was kept at 1 ml/min with 25 µl of sample injection volume. Fifteen minutes run time was maintained. Uptake rate was expressed as µmol absorbed g−1 root fresh weight min−1.
Enzyme assays
Activities of nitrogen assimilatory enzymes namely, NR and glutamine syntheses (GS) were analyzed in both leaves and roots to workout the basis of variability in Nitrogen Assimilation measured in 20-day-old wheat genotypes.
In-vitro assay of NR activity
Activity of NR, the enzyme catalyzing the first step of nitrogen assimilation was analyzed by the method of Ahmad and Abdin (Citation1999). Root tissue was frozen in liquid nitrogen and homogenized in extraction mixture consisting 25 mM Potassium phosphate (pH 7.5). The extract was filtered through muslin cloth and then centrifuged. This crude extract was assayed for enzymatic activity. Enzyme extract and reaction mixture containing, 100 µM Potassium phosphate buffer pH 6.8, was incubated at 33°C for 30 min. Reaction was stopped by the addition of zinc acetate. Nitrite produced was estimated at 540 nm using UV-Vis spectrophotometer (Model λ-bio-20, PerkinElmer, Germany). At each treatment the NR activity was determined in two sets of experiment each with three replicates. The enzyme activity was expressed as µmol g−1 DW h−1.
In-vitro assay of glutamine synthase activity
Enzyme was assayed using the method of McNally et al. (Citation1983). Root tissue was homogenized in liquid nitrogen and extraction medium containing Tris-HCl buffer (pH 7.6) was added to it. The extract was filtered through muslin cloth and then centrifuged. This crude extract was assayed for enzymatic activity. Reaction mixture contained Tris-HCl buffer (pH 8), ATP, sodium glutamate, MgSO4, L-cysteine, hydroxylamine was added to enzyme extract. Reaction was started by addition of hydroxylamine. The mixture was incubated at 30°C for 30 minutes-glutamyl hydroxamate (GH) was measured at absorbance 540 nm by using UV-Vis spectrophotometer (-BIO 20, Perkin Elmer, Germany). The activity was calculated by using the standard curve of GH. At each treatment the glutamate synthase enzyme activity was measured in two sets of experiment each with three replicates. Enzyme activity was expressed as µmol-GH formed g−1 DW min−1.
Nitrate content
Nitrate content of root was estimated following Grover et al. (Citation1978). The plant material was boiled with charcoal to release the leaf extract. This extract was then filtered. Catalyst solution (copper sulphate + zinc sulphate), hydrazine sulphate and sodium hydroxide were added to the aliquot. The mixture was incubated at 33°C for 10 min and then transferred to ice bath. To this, acetone, 1% sulphanilamide in 1N-HCl and 0.02% NEDD were added. Pink color was allowed to develop. Absorbance was read at 540 nm using UV-Vis Spectrophotometer (Model λ-bio-20, PerkinElmer, Germany). The experiment repeated twice with three replicates for each treatment. The calibration curve was prepared using potassium Nitrate solution. The nitrate content was expressed as µmol NO3 produced g−1 DW h−1.
Total nitrogen content
Nitrogen concentration (in percentage) was determined using Elementar (CHNS Analyser, Vario EL III, Germany). Roots of wheat varieties were dried in oven at 65°C for 72 hours. Dried material of root of varieties were grounded into fine powder using mortar and pestle and processed in Elementar to measure nitrogen. The experiment was conducted twice with three replicates for each treatment.
Data analysis
Data were analyzed according to factorial randomized block design, performing analysis of variance (ANOVA) for each treatment separately. Significance of differences among treatments, varieties, and their interaction was determined by the least significant difference (LSD) calculated at p<0.05.
Real-time RT-PCR
Total RNA was extracted from 20-day-old plants placed in nutrient solution with 1 mM and 10 mM nitrate levels with method of Chomsczynsky and Sacchi (Citation1987), and 1 mg of total RNA was reverse transcribed with oligo (dT) primer using ReverAid™ H Minus First strand cDNA synthesis kit (Fermentas Life Science, USA). The following gene-specific primers were used for NADH NR NADH-NAR1 (forward, 5′- GAATTCCAAGCAGTTCACCATGTCCGA-3′; reverse 5′- GAATTCGATCTCGT CGACCATGCTCGT-3′), primers used for fd-cytosolic GS were: GLN1;1 (high-affinity form) (forward, 5′-TTGAGTCGCATATCGCCCACTTGC-3′; reverse 5′-GGACAGGACACCATCAACTCTCA-3′) (accession no. AY621539.1). RNA for GS genes was extracted from 20-day-old plant grown in 1 mM N and 10 mM N treatment. Actin (AB047313), which was defined as 100 relative expression units (REU), was used as an internal standard in these experiments. The expression level of genes corresponds to the ratio of the copy number of cDNA of the studied gene to the copy number of the “housekeeping” gene Actin multiplied by 100 REU. Quantitative real time RT-PCR was performed with the QuantiTect SYBR Green PCR Kit (Qiagen) and LightCycler (Roche).
Result and discussion
Growth and NE
Growth was measured in terms of fresh weight and dry weight of the plants. Wheat genotypes showed three growth patterns in response to applied nitrogen. Growth of the genotypes of first category increased with the increase in the level of nitrogen in nutrient solution. Interestingly, the growth of genotypes of second category was maximum at 1 mM N treatment, and the growth was not significantly affected with increase in N levels. The third category showed increase in their growth upto 4 mm N application and no further increase in growth was observed at 10 mM N application. HUW-468, HUW-206, and UP-262 genotype belongs to first category, VL-421 and HS-277 to second category and PBW-154, 3077RAJ, HD-2270, HD-2307, and PBW-65 genotypes, belongs to third category ().
Table 1. Effect of nitrogen treatments on shoot fresh weight (g), root fresh weight (g), shoot dry weight (mg), root dry weight (mg) and nitrogen efficiency (%) of wheat genotypes.
Nitrogen efficiency of wheat genotypes showed large variability, from 40.5% to 94.6%. The genotypes having NE below than 50–60% are grouped as Low Nitrogen Efficient (LNE). The genotypes having NE of more than 60–90% are grouped as Moderate Nitrogen Efficient (MNE). The genotypes having NE of more than 90% are grouped as High Nitrogen Efficient (HNE). HUW-468 (53.9%, 40.5%), HUW-206 (57.5%, 46.5%), UP-262 (58.35, 48.2%) was categorized as LNE, PBW-154 (73.0%, 62.9%), 3077Raj (71.7%, 67.3%), HD-2270 (69.7%, 61.18%), HD-2307 (78.3%, 74.6%), and PBW-65 (79.4%, 67.6%) were categorized as MNE and VL-421 (94.2%, 91.4%) and HS-277 (96.6, 94.6%) were categorized as HNE ().
Nitrogen in plants plays an important role in their growth. Growth of the wheat genotypes in our study showed large variation in response to nitrogen treatments. Earlier reports also showed that the number of leaves, their rate of appearance and number of nodes, number of tilters (Bradley and Kindred Citation2009), and shoot length are affected by N limiting conditions. Gastal and Nelson (Citation1994) showed in grasses that the reduction of leaf area extension and the relationship between leaf area index and N uptake was found to be directly proportional. It has also been shown that the morphology of the root system may be influenced by a locally restricted nitrate supply. It was observed that N application rates affected various essential components of root morphology such as length, number of apices and frequency of branching (Shangguan et al. Citation2004). Studies have shown that there is a weak but significant correlation between root traits and biomass production. A relationship between root architecture and N assimilation under low N fertilization inputs has also been reported in wheat (Chandna et al. Citation2011) and maize (Laperche et al. Citation2007).
Biomass accumulation capacity of wheat genotypes was measured at low (1 mM), moderate (4 mM), and high (10 mM) nitrogen levels (). Supply of nitrogen howed enhanced biomass accumulation in wheat genotypes, compared with plants grown under a low N (T1) condition, with an exception of the genotype VL-421, whose growth was not affected by N supply, thus indicating its high tolerance to the low levels of N treatment (T1). Tolerance to N deficiency was then established on the basis of calculated NE with considerations for the growth of the whole shoot. The whole shoot dry weight based NE has long been an extensively used parameter for assessing genotypic variation in tolerance to other nutrient deficiency (Ahmad et al. Citation2005). The NE showed a very large variation among genotypes (). VL-421 showing maximum NE (94.6%) clearly indicated the ability of these genotypes to accumulate higher biomass than MNE and LNE genotypes under low N supply condition. This may be because of efficient utilization of N into protein synthesis. Since, its well-known fact that Rubisco accounts for 50–60% of the total soluble protein in leaves, efficient utilization of N directly correlated with the increase in Rubisco content, and thereby photosynthesis, leading to the higher biomass production (Chandna et al. Citation2010). HNE plants are highly desirable because these can be grown with limited N supply for environment friendly farming systems.
Km of nitrate uptake
Nitrate uptake kinetic of wheat varieties was expressed in terms of K m. Interestingly, large variability in the K m values of wheat varieties was observed. The LNE genotypes were observed with K m of HUW-468 (51.2), HUW-206 (48.01), UP-262 (49.32). MNE genotypes of wheat were observed with K m of PBW-154 (22.68), 3077Raj (21.01), HD-2207 (18.06), HD-2307 (22.42), and PBW-65 (29.32), respectively. HNE (VL-421 and HS-277) had K m of 0.4 and 0.81 µM, respectively ().
Nitrate uptake was determined as the amount of nitrate depleted from the uptake solutions. In the present study, K
m values of the selected 10 genotypes varied from 0.4 to 52 mM (). These values were in general agreement with other plant species ranging 0.007–0.187 mM. K
m values have been reported as being 0.033 mM for perennial ryegrass, 0.040 mM for Arabidopsis (Herbert et al. Citation1995), 0.027 mM for wheat (Goyal and Huffaker Citation1986), 0.281 mM in Citrus (Carezo et al. Citation1997), and 7.5 mM excised corn root (Sehtiya and Goyal, Citation2000). Rroco et al. (2000) examined eight wheat varieties and Chandna et al. (2010) examined 16 wheat varieties with respect to their uptake kinetics, and observed four-fold differences for apparent K
m. The variations in endogenous levels of plants, different Mo status of roots and intensive breeding of some species for high nitrogen response have been proposed as possible explanation for variations in K
m values. In addition, since
efflux by roots is affected by
content of roots, factors affecting
reduction during the plant growth are also thought to influence net
uptake rate and its K
m. One theoretical explanation for possible differences in K
m and V
max (i.e. capacity of the saturable component of the uptake system) is that genotypes differ in the quantity (number) of transport molecules per unit of absorbing cell membrane (Chopin et al. Citation2007).
Most studies on the kinetic and energetic aspects of uptake have been confined to low concentration system, wherein the net nitrate uptake rate normally obeys Michaelis–Menten kinetics (Herbert et al. Citation1995). As to the kinetic parameters (V
max and K
m), it is difficult to relate the magnitude of genotypic difference to performance under nutrient-limiting conditions. The kinetic analysis of nitrate uptake into intact roots of induced and uninduced seedlings of VL-421 and HS-277 (HNE) demonstrated that nitrate uptake in the concentration range of 0.01–3.0 mM is mediated by two distinct transport systems in types of seedlings. At low nitrate concentrations (0.01–1.0 mM), uptake of nitrate occurs by means of high-affinity saturable system (HATS) in both induced and uninduced plants. At high nitrate concentrations (1.0–4.0 mM), the nitrate uptake system is of low affinity (LATS) and shows a linear non-saturable dependence on nitrate concentrations in both types of seedlings. Nitrate uptake in the concentration range of 0.01–4.0 mM is mediated by only one transport system in both induced and uninduced seedlings of HUW-468, HUW-206, and UP-262 (LNE). At low nitrate concentrations, uptake of nitrate occurs by means of high-affinity saturable system (HATS) in both the plant types. High concentrations of nitrate inhibited the uptake. In the induced and uninduced seedlings of PBW-154, 3077Raj, HD-2207, HD-2307, and PBW-65 (MNE), nitrate uptake in the concentration range of 0.01–4.0 mM is mediated by one transport system only. At low nitrate concentrations, uptake of nitrate was very low, but at high concentrations, seedlings were able to take up nitrate from the uptake solution actively and the nitrate uptake system was of low affinity (LATS), showing a linear non-saturable dependence on nitrate concentrations (Chandna et al. Citation2011).
Nitrate assimilatory enzymes
Once taken up by soil nitrate () is assimilated via a series of enzymatic steps. The first step, in which
is reduced to
, involves the transfer of two electrons.
Nitrate reductase is the enzyme which catalyzes the first step of nitrate assimilation in plants, leading to reduction of nitrate to nitrite with pyrimidine nucleotide in higher plants. Variability in the activity of this enzyme was observed at various levels of nitrate treatments. The studies conducted at whole plant level in cereals and other species of crop plants indicated that shoots have high NR activity as compared to roots (Oaks Citation1994). Analysis of shoot in cereals like wheat (Chandna et al. Citation2010), corn (Gupta and Beevers Citation1983) and barley (Chatterjee et al. Citation1981) revealed that major amount of nitrate is assimilated by leaf blades. Studies done by Ahmad et al. (Citation2005) suggested that genotypic differences were present in NR activity. One of the likely factors in NR regulation seems to be the differences in the uptake of nitrate and accumulation of nitrate ions by the genotypes. Likewise in the present study, different genotypes showed significant differences in the enhancement of NR activity with the supply of various N levels.
The NR activity of HUW468, HUW-206, and UP-262 (LNE genotypes) was increased significantly by 22%, 47%, and 48% with the supply of 4 mM N (T2), and by 95%, 81%, and 77% with the supply of 10 mM N (T3), when compared with T1. In MNE genotypes (PBW-154, 3077Raj, HD-2207, HD-2307, and PBW-65), there was a significant increase in the NR activity with increase in 4 mM N. No further increase in the activity of this enzyme was observed. However, the increase in NR activity was lesser than LNE genotype. The increase in the NR activity was 64%, 20%, 38%, 30%, and 42%, respectively with the supply of 4 mM N (T2), and it was 68%, 12%, 33%, 46%, and 36%, respectively with the supply of 10 mM N (T3) over 1 mM N (T1). Increase in the level of N treatment (4 and 10 mM) did not affect the NR activity of HNE genotypes (VL-421 and HS-277) significantly, when compared with 1 mM (T1) ().
Table 2. Effect of nitrogen treatment on the nitrate reductase activity (µmol g−1 DW h−1), glutamate synthase activity (µmol γ-GHA g−1 DW min−1), nitrate estimation (µmol g−1 DW) and total nitrogen content (%) in roots of wheat genotypes.
This difference in activity indicates the threshold difference of the enzyme in HNE, MNE, and LNE genotypes. In high efficient genotypes, the NR enzyme showed high affinity for N thus the threshold of the NR enzyme seems to be low. Thus, the activity of enzyme becomes constant at all concentration of N. At high N levels, the feedback mechanism occurs at early stage, therefore showing no further significant change in its activity. In moderate efficient genotypes, the threshold level of NR enzyme is slightly higher than HNE genotypes therefore it is able to utilize N at 4 mM N but becomes constant when N is increased to 10 mM level, exhibiting feedback mechanism. NR enzyme has high threshold level in low efficient genotypes, showing low affinity for substrate, thus they respond well at high N doses (Anjana et al. Citation2010).
Ammonium originating in the plant from reduction is incorporated into an organic form primarily by the enzyme GS (EC 6.3.1.2). This enzyme catalyses the conversion of the amino acid, glutamate into the amide, glutamine (Gln), using NHx
4, ATP and a divalent cation such as Mg2 + , Mn2 + , or Co2 + as a cofactor. Gln then serves as one of nitrogen donors for the biosynthesis of organic nitrogenous compounds, such as amino acids, nucleotides, and chlorophyll. Thus, the GS enzyme is likely to be a key factor controlling plant nitrogen assimilation (Cai et al. Citation2009).
The Glutamate synthetase activity in LNE genotypes (HUW-468, HUW-206, and UP-262) also showed marked increase by 115%, 67%, and 90% with the supply high N levels (10 mM N), when compared with 1 mM (T1). In MNE genotypes (PBW-154, 3077Raj, HD-2207, HD-2307, and PBW-65) there was a significant increase in the GS activity with increase in 4 mM N. No further increase in the activity of this enzyme was observed. The increase in the GS activity was 42%, 17%, 60%, 75%, and 66%, respectively with the supply of 4 mM N (T2), and it was 89%, 28%, 87%, 5%, and 50%, respectively with the supply of 10 mM N (T3) over 1 mM N (T1). Higher doses of N treatments (10 and 25 mM) did not affect the GS activity of HNE genotypes (VL-421 and HS-277), when compared with 1 mM (T1) (Table 2).
Studies done by Masclaux et al. (Citation2001) revealed that in the LNE genotypes a substantial portion of N is not re-translocated to the harvested structures and in this the GS activity in leaves is lower as compared to the HNE genotypes. Hence, the ability of the HNE genotypes to harvest more N and redistribute to grains is due to the well-coordinated system of N uptake and assimilation. In LNE genotypes, GS activity was sufficiently high to maintain a low level of ammonium in leaf tissues irrespective of external N supply that explains the increase in activity of GS with increase in N supply. In addition to this, it was observed that GS activity was two-folds higher in plants growing at low N levels than plants growing at high N levels (Cao et al. Citation2008). It appears reasonable to conclude that high GS activity in the chloroplasts would be a key point to detoxify ammonium by incorporating it into amino acids as the sink for ammonium and carbohydrate as well as an excellent biochemistry parameter for a genetic screening program to obtain nitrogen assimilating efficient genotypes (Magalhães et al. Citation1993).
Expression of NR and GS gene families
Using real-time RT-PCR, the expression levels of NR were analyzed in the leaves of HUW-468 (LNE) and VL-421 (HNE) under low and high nitrogen conditions (). Expression of NR was upregulated by 3.5-fold in the leaves of HUW-468 at 10 mM N compared with 1 mM N, whereas in VL-421 no change in expression levels was observed at low and high nitrogen conditions. Subsequently, using real-time RT-PCR, we also analyzed expression levels of the GS gene (Gln1;1) in leaves of VL-421 and HUW-468 at 1 and 10 mM nitrogen (). Gln1;1 (high-affinity form) of GS isoform is responsible for the primary assimilation of ammonium ions in plants. The expression of Gln1;1 was up-regulated in VL-421 by 1.0-fold under low-nitrogen levels (P50.05) in comparison with HUW-468, and no significant change in the expression level of Gln1;1 was observed with increase in N supply. However, HUW-468 showed an increase in the expression of Gln1;1 at 10 mM N by one-fold ().
Figure 1. Expression of NADH-nitrate reductase in leaves of HUW-468 and VL-421 in dependence of N concentration in nutrient solution.
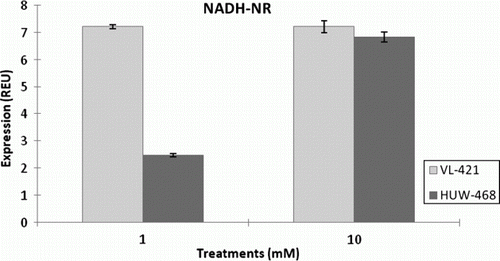
Figure 2. Expression of Glutamine synthase gene family member (Gln1;1) in leaves of HUW-468 and VL-421 in dependence of N concentration in nutrient solution.
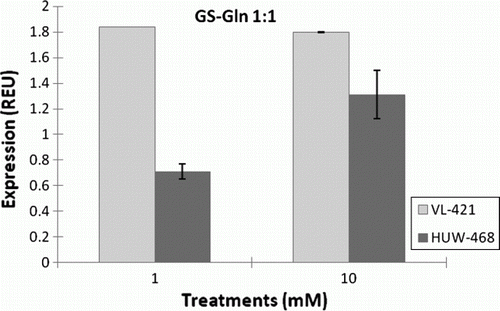
Levels of expression of the GS gene family (Gln1;1) in leaves of VL-421 under low N levels show up-regulation in comparison with transcript expression of HUW-468 under high nitrogen conditions. Hence, the ability of the HNE genotypes to harvest more N and redistribute to grains is due to the well-coordinated system of N uptake and assimilation (Shi et al. Citation2010). In LNE genotypes, GS activity was sufficiently high to maintain a low level of ammonium in leaf tissues irrespective of external N supply (Cao et al. Citation2008), which explains the increase in activity of GS with increase in N supply. Sakakibara et al. (Citation1992) reported that GS enzyme is induced within 30 minutes of nitrate addition, indicating that this enzyme is induced directly by nitrate application. In addition to this, it was observed that GS activity was 0.5–2-fold higher in plants able to grow at low N levels than plants growing at high N levels (Cao et al. Citation2008). It appears reasonable to conclude that high GS activity in the chloroplasts would be the key to detoxifying ammonium by incorporating it into amino acids as the sink for ammonium and carbohydrate, as well as an excellent biochemistry parameter for a genetic screening program to obtain efficient nitrogen assimilating genotypes (Brauer and Shelp Citation2010).
These results show that a HNE wheat genotype is more efficient in N uptake and assimilation even at low nitrogen conditions.
Nitrate content and nitrogen content
There was a 79%, 76%, and 68% increase in the nitrate content of HUW-468, HUW-206, and UP-262 (LNE genotypes), respectively with of 4 mM N treatment, and a 140%, 129%, and 106%, respectively with the supply of 10 mM N treatment, when compared with T1 (1 mM N). In MNE genotypes, there was no significant effect of supply of 10 mM N treatment on the nitrate content of the genotypes studied, when compared with T1. However, supply of higher dose of N (25 mM) increased the nitrate content by 83%, 68%, 97%, 88%, and 35%, respectively in PBW-154, 3077Raj, HD-2207, HD-2307, and PBW-65 over T1. No significant effect of supply of higher levels of N was observed on the nitrate content of HNE genotypes (VL-421 and HS-277). Similar trend of nitrate content in response to supplied N was observed in roots ().
Low NR activity in LNE genotypes might be a reason for accumulation of more nitrate revealing that there is a negative correlation between NR activity and nitrate concentration (Anjana et al. Citation2007). Olday et al. (Citation1976) found that other non-legume plants accumulated more nitrate with increase in N treatments and showed that the highest accumulation was in the shoot. Such effects are normally considered positive, since excess availability of nitrate resulted in enhanced dry weights. Study done by Chen et al. (Citation2004) showed that the nitrate concentration in both the metabolic pool (MP) and the storage pool (SP) of the leaf blades increased with nitrate supply. The nitrate concentration increased with nitrate supply in the whole plant level.
With the supply of higher levels of N, the genotypes responded differently in terms of the nitrogen contents. There was no significant effect of supply of higher levels of N (4 and 10 mM) on the nitrogen content of LNE genotypes when compared with T1 (1 mM N). In MNE genotypes (PBW-154, 3077Raj, HD-2207, HD-2307, and PBW-65), the N content increased by 14%, 15%, 11%, 13%, and 12%, respectively, with the supply of 4 mM N (T2), over T1 (1 mM). However, no significant increase in N content of MNE genotypes was reported with the supply of 25 mM N. The N content of HNE genotypes remained unaffected by the higher levels of N ().
Nitrogen content among root and shoot of wheat genotypes showed significant variation with increase in N supply. Genetic differences for N utilization have been shown for wheat (Le Gouis et al. Citation2000). The difference in nitrogen content may be due to the fact that nitrogen accumulation efficiency is based on biomass production and tissue nitrogen content. This shows that in low efficient genotypes the reduced N metabolites are being distributed in plant parts and mostly N gets stored in vegetative parts (Martre et al. Citation2003).
Conclusions
The present study shows that genotypes with high NE are able to grow and perform well in limited nitrogen conditions, indicating that their high NUE can be used for environmentally friendly farming systems. These genotypes with high uptake efficiency had higher nitrogen contents than genotypes with low uptake efficiency from nitrogen application. Therefore, these genotypes could reduce nitrate contamination in the environment. This study also brings an idea that integration of physiological studies coupled with various agronomic management practices may help to improve the utilization of nitrogenous fertilizers, which are one of the major inputs in Indian agriculture.
Notes
Ruby Chandna and Khalid Rehman Hakeem contributed equally to this work.
References
- Ahmad , A and Abdin , MZ . 1999 . NADH: nitrate reductase and NAD (P) H: Nitrate reductase activity in mustard seedlings . Plant Sci. , 143 : 1 – 8 .
- Ahmad , A , Khan , I , Anjum , NA , Diva , I , Abrol , YP and Iqbal , M . 2005 . Effect of timing of sulphur fertilizer application on growth and yield of rapeseed (Brassica campestris L.) . J Plant Nut. , 28 : 1049 – 1059 .
- Anjana US, Abrol YP, Iqbal M. 2010 . Modulation of nitrogen-utilization efficiency in wheat (Triticum aestivum L.) genotypes differing in nitrate reductase activity . J. Plant Nutrition , in press .
- Anjana , US , Iqbal , M and Abrol , YP . 2007 . Are nitrate concentrations in leafy vegetables within safe limits? . Current Sci. , 92 : 355 – 360 .
- Beman , JM , Arrige , K and Matson , PM . 2005 . Agricultural runoff fuels large phytoplankton blooms in vulnerable areas of the ocean . Nature , 434 : 211 – 216 .
- Bradley , RS and Kindred , DR . 2009 . Analyzing nitrogen responses of cereals to prioritize routes to the improvement of nitrogen use efficiency . J Exp Botany. , 60 : 1939 – 1951 .
- Brauer , EK and Shelp , BJ . 2010 . Nitrogen use efficiency: re-consideration of the bioengineering approach . Botany. , 88 : 103 – 109 .
- Cai , H , Zhou , Y , Xiao , J , Li , X , Zhang , Q and Lian , X . 2009 . Overexpressed glutamine synthetase gene modifies nitrogen metabolism and abiotic stress responses in rice . Plant Cell Rep. , 28 : 527 – 537 .
- Cao , Y , Fan , XR , Sun , S , Xu , G , Hu , J and Shen , Q . 2008 . Effect of nitrate on activities and transcript levels of nitrate reductase and glutamine synthetase in rice . Pedosphere , 18 : 664 – 673 .
- Carezo , M , Garcia-Agustin , P , Serna , MD and Primo-Millo , E . 1997 . Kinetics of nitrate uptake by citrus seedlings and inhibitory effects of salinity . Plant Sci. , 126 : 105 – 112 .
- Chandna , R , Gupta , S , Ahmad , A , Iqbal , M and Prasad , M . 2010 . Variability in Indian bread wheat (Triticum aestivum L.) varieties differing in nitrogen efficiency as assessed by microsatellite markers . Protoplasma. , 242 : 55 – 67 .
- Chandna R, Kaur G, Iqbal M, Khan I, Ahmad A. 2011 . Differential response of wheat genotypes to applied nitrogen: biochemical and molecular analysis . Arch Agro Soil Sci . doi: 10.1080/03650340.2011.555761
- Chatterjee , SR , Pokhriyal , TC and Abrol , YP . 1981 . Nitrogen economy of the main shoot of field-grown Barley (Hordeum vulgare L.) II. In vivo nitrate reductase activity during growth . J Exp Botany. , 32 : 701 – 712 .
- Chen , H , Hsu , D and Li , CW . 2004 . A new method to produce nanoscale iron for nitrate removal . J Nano Res. , 6 : 639 – 647 .
- Chomsczynsky , R and Sacchi , N . 1987 . Single-step method of RNA isolation by acid guanidinium thiocyanate–phenolchloroform extraction . Anal Biochem. , 162 : 156 – 159 .
- Chopin , F , Orsel , M , Dorbe , MF , Chardon , F , Truong , HN , Miller , AJ , Krapp , A and Daniel-Vedele , F . 2007 . The Arabidopsis ATNRT2.7 nitrate transporter controls nitrate content in seeds . Plant Cell. , 19 : 1590 – 1602 .
- Edhaie , B and Waines , JG . 2001 . Sowing date and N rate effects on dry matter and N partitioning in bread and durum wheat . Field Crops Res. , 73 : 47 – 61 .
- Forde , BG . 2002 . Local and long-range signaling pathways regulating plant responses to nitrate . Ann Rev Plant Biol. , 53 : 203 – 224 .
- Gastal , F and Nelson , CJ . 1994 . Nitrogen use within the growing leaf blade of tall fescue . Plant Physiol. , 105 : 191 – 197 .
- Goyal , SS and Huffaker , RC . 1986 . The uptake of NO3− and NH4+ by intact wheat (Triticum aestivum) seedlings . Plant Physiol. , 82 : 1051 – 1056 .
- Grover , HL , Nair , TVR and Abrol , YP . 1978 . Nitrogen metabolism of the upper three leaf blades of wheat at different soil levels. I. Nitrate Reductase activity and content of various nitrogenous constituents . Physiol Plant. , 43 : 287 – 292 .
- Gupta , SC and Beevers , L . 1983 . Environmental influences on nitrite reductase Activity in Pisum sativum L. Seedlings . J Exp Bot. , 34 : 1455 – 1462 .
- Hakeem , KR , Altaf , A , Iqbal , M , Gucel , S and Ozturk , M . 2011 . Nitrogen-efficient rice cultivars can reduce nitrate pollution . Env Sci Poll Res. , 18 : 1184 – 1193 .
- Herbert , J , Kronzucker , M , Siddiqi , Y and Anthony , DM . 1995 . Kinetics of NO,− influx in spruce . Plant Physiol. , 109 : 319 – 326 .
- Hirel , B , Bertin , P , Isabella , Q , Bourdoncle , W , Attagnant , C , Dellay , C , Gouy , A , Cadiou , S , Retailliau , C Falque , M . 2001 . Towards better understanding of thegenetic and physiological basis for nitrogen use efficiency in Maize . Plant Physiol. , 125 : 1258 – 1270 .
- Hunt , J and Seymour , DJ . 1985 . Method for measuring Nitrate-nitrogen in vegetables using anion-exchange High performance liquid chromatography . Analyst , 110 : 131 – 133 .
- Jeffrey , A , Kaplan , I , Zhang , DS , Shan-Tan , T and Nielsen , J . 2002 . Environmental tracers: identifying the sources of nitrate contamination in groundwater . Soil Sed Waters , 6 : 15 – 20 .
- Ladha , JK . 2005 . Improving the recovery efficiency of fertilizer nitrogen in cereals . J India Soc Soil Sci. , 53 : 472 – 477 .
- Laperche , A , Brancourt-Hulmel , M , Heumez , E , Gardet , O , Hanocq , E , Devienne-Barret , F and Le Gouis , J . 2007 . Using genotype x nitrogen interaction variables to evaluate the QTL involved in wheat tolerance to nitrogen constraints . Theo App Gene. , 115 : 399 – 415 .
- Le Gouis , JD , Heumez , BE and Pluchard , P . 2000 . Genetic differences for nitrogen uptake and nitrogen utilization efficiencies in winter wheat . Euro J Agro. , 12 : 163 – 173 .
- Magalhães , JR , Machado , AT , Fernandes , MS and Silveira , JAG . 1993 . N assimilation efficiency in maize genotypes under ammonia stress . Rev Bras de Fisiol Veg Bras. , 5 : 163 – 166 .
- Martre , P , Porter , JR and Jamieson , PD . 2003 . Modeling grain nitrogen accumulation and protein composition to understand the sink/source regulations of nitrogen remobilization for wheat . Plant Physiol. , 133 : 1959 – 1967 .
- Masclaux , C , Quillere , I , Gallis , A and Hirel , B . 2001 . The challenge of remobilization in plant nitrogen economy. A survey of physio-agronomic and molecular approaches . Ann Appl Biol. , 138 : 69 – 81 .
- McNally , SF , Hirel , B , Gadal , P , Mann , AF and Stewart , GR . 1983 . Glutamine synthethase of higher plants: evidences for a specific isoform content related to their possible physiological and their compartmentation within the leaf . Plant Physiol. , 72 : 22 – 25 .
- Moll , RH , Kamprath , EJ and Jackson , WA . 1982 . Analysis and interpretation of factors which contribute to efficiency of nitrogen utilization . Agro J. , 74 : 562 – 564 .
- Oaks , A . 1994 . Primary nitrogen assimilation in higher plants and its regulation . Canad J Bot. , 72 : 739 – 750 .
- Olday , FC , Barker , AV and Maynard , DN . 1976 . A physiological basis for different patterns of nitrate accumulation in cucumber and pea . J Am Soc Hort Sci. , 101 : 219 – 221 .
- Pathak , RR , Ahmad , A , Lochab , S and Raghuram , N . 2008 . Molecular physiology of plant nitrogen use efficiency and biotechnological options for its enhancement . Current Sci. , 94 : 1394 – 1403 .
- Rroco , E , Kosegarten , H and Mengel , K . 2002 . Mechanisms of nitrogen release from roots of intact wheat plants (Triticum aectivum L.) . Plant Nutrit. , 92 : 206 – 207 .
- Sakakibara , H , Kawabata , S , Takahashi , H , Hase , T and Sugiyama , T . 1992 . Molecular cloning of the family of glutamine synthetase genes from maize: expression of genes for glutamine synthetase and ferredoxin-dependent glutamate synthetase in photosynthetic and nonphotosynthetic tissues . Plant Cell Physiol. , 33 : 49 – 58 .
- Sehtiya , HL and Goyal , SS . 2000 . Comparative uptake of nitrate by intact seedlings of C-3 (barley) and C-4 (corn) plants: effect of light and exogenously applied sucrose . Plant Soil. , 227 : 185 – 190 .
- Shangguan , ZP , Shao Ren , SJ , Zhang , LM and Xue , Q . 2004 . Effect of nitrogen on root and shoot relations and gas exchange in winter wheat . Bot Bull Acad Sinica. , 45 : 49 – 54 .
- Shi , WM , Xu , WF , Li , SM , Zhao , XQ and Dong , GQ . 2010 . Responses of two rice cultivars differing in seedling-stage nitrogen use efficiency to growth under low-nitrogen conditions . Plant Soil. , 326 : 291 – 302 .
- Stulen , I , Pérez-Soba , M , De Kok , LJ and Van der Eerden , L . 1998 . Impact of gaseous nitrogen deposition on plant functioning . New Phytolo. , 139 : 61 – 65 .
- Touraine , B, Daniel-Vedele F, Forde B. 2001 . Nitrate uptake and its regulation . In : Lea PJ, Morot-Gaudry JF Plant nitrogen. Berlin-Heidelberg: INRA Editions and Springer-Verlag .