Abstract
In this study, we isolated a homozygous T-DNA insertion mutant line, ckl2, of the casein kinase 1-Like 2 (CKL2) gene in Arabidopsis thaliana. Through analysis of the germination ratio, root length, water loss rate, and stomatal aperture, we found that the ckl2 mutants showed an abscisic acid (ABA)-hyposensitive phenotype in all tests. CKL2 was also found to mediate the expression of ABA-upregulated genes in seeds and seedlings. CKL2 was expressed in all tissues, especially in the leaf and leaf stalk, and could be induced by ABA. A proline accumulation experiment showed that the Pro content in ckl2 was lower than that of the wild type. The above results demonstrate that CKL2 was required for ABA-regulated seed germination, root growth, and gene expression, suggesting that CKL2 positively mediates ABA signaling in Arabidopsis.
Introduction
In vivo protein phosphorylation events control most of the cellular processes in a given organism and involve an unexpectedly high number of protein kinases (Kersten et al. Citation2006). Phosphorylation of serine, threonine, and tyrosine residues by cellular protein kinases plays an important role in the regulation of various cellular processes. Casein kinase (CK) is a serine/threonine specific protein kinase, including CK1 and CK2 families. CK1 and CK2 were among the earliest described protein kinases (Knippschild et al. Citation2005). CK2, which forms a tetrameric complex including two α-subunits and two β-subunits, is a highly conserved protein kinase and could be found in various organisms, such as yeast, plants, and mammals. CK2 can phosphorylate a range of different proteins, including transcription factors, and can modulate DNA-binding ability, intracellular localization, and protein stability by interacting with partner proteins (Ogiso et al. Citation2010).
All CK1 family members are monomer structure, which have a highly conserved kinase domain, but their N-terminal (9–76 amino acids [aa]) and C-terminal noncatalytic domains (24 aa up to more than 200 aa) are significantly different in the length and primary structure. CK1 isoforms exclusively use ATP as phosphate donor and generally do not require co-factors (Graves & Roach Citation1995; Knippschild et al. Citation2005). The substrate specificity of CK1 isoforms initially led to the identification of the canonical consensus sequence S/T(P)-X1–2-S/T (X represents any aa) (Agostinis et al. Citation1989). Interestingly, the CK1 family shows a strong preference both in vitro and in vivo for ‘primed,’ pre-phosphorylated substrates (Cheong & Virshup Citation2011). Considering the broad distribution of CK1 family members and their substrates, these kinases have been found to participate in many biological activities, such as Wnt signaling (Peters et al. Citation1999; Price Citation2006), biological rhythm (Etchegaray et al. Citation2009; Lee et al. Citation2009), DNA repair (Dhillon & Hoekstra Citation1994), and the cell cycle (Behrend et al. Citation2000; Petronczki et al. Citation2006). The Arabidopsis thaliana genome was found to contain 14 casein kinase 1-like (CKL) genes, and, within the conserved kinase domains, they share 89% sequence similarity at the aa level. It has been reported that CKL6 could participate in anisotropic cell growth and shape formation through the regulation of microtubule organization (Ben-Nissan et al. Citation2008). CKL3 was involved in abiotic stress, such as hormone, salt, and osmotic stress (Wang et al. Citation2011). So we assumed that CKL2 could participate in abscisic acid (ABA) signaling in higher plant.
CKL2 belongs to CKLs that is localized in the cytoplasm and nucleus (Lee et al. Citation2005). In this study, we isolated an Arabidopsis T-DNA insertion mutant line, ckl2. The mutant was hyposensitive to ABA in seed germination and seedling growth. The results obtained suggest that CKL2 may be involved in ABA signal transduction pathways and play a positive regulatory role.
Materials and methods
Plant material and growth conditions
The T-DNA insertion mutant of the CKL2 gene in Arabidopsis was identified in the Salk collection corresponding to donor stock number SALK_097886, and it was named ckl2 in this study. Arabidopsis ecotype Columbia-0 (Col-0) was used as the wild type along with the ckl2 mutant for germination and gene expression analyses. The plants were routinely grown in a growth chamber under 40% humidity with a temperature of 22°C and a 16 h light/8 h dark photoperiod at 100–150 µmol m−2 s−1 of light. For in vitro culture, seeds were surface sterilized three times in 70% ethanol for 1 min, soaked for 10 min in 20% NaClO, and, finally, washed five times in sterile distilled water. Stratification of the seeds was conducted during 3 days at 4°C. Afterwards, seeds were sowed on Murashige and Skoog (1962) plates. The Murashige and Skoog (MS) plates contained solid medium composed of MS basal salts and 1% (w/v) Suc solidified with 0.8% (w/v) agar, and the pH was adjusted to 6 with NaOH before autoclaving. The plates were sealed and incubated in a controlled environment growth chamber.
Mutant identification by PCR screening
The SALK_097886 line, which contains a single T-DNA insertion in CKL2, was obtained from the Salk Institute Genomic Analysis Laboratory. To identify individuals homozygous for the T-DNA insertion, genomic DNA was obtained from seedlings and submitted for PCR using the following primers: LP, 5′-ACCAGTTTCAAGTGATCCTGC-3′, and RP, 5′-GTGGTGATCCAACCACAAATC-3′. The T-DNA left border primer of the pROK2 vector was LBpROK2 (5′-TGGTTCACGTAGTGGGCCATC-3′).
Germination assays and root growth
To score seed germination, seeds were plated on solid medium composed of MS basal salts, 1% Suc, and different concentrations of ABA (0, 0.1, 0.3, or 0.6 µM). To determine the sensitivity to the inhibition of germination by high osmoticum, the medium was supplemented with different concentrations of NaCl (0, 50, 100, or 150 mM) and mannitol (0, 50, 150, or 350 mM). Each value represents the average seed germination percentage of approximately 50 seeds in at least three replicates.
The root growth assay for scoring ABA sensitivity was performed by measuring root growth 12 d after the transfer of five-day-old seedlings onto vertical MS plates containing 0, 10, or 40 µM ABA.
RNA extraction and quantitative RT-PCR
Total RNA was isolated from imbibed seeds, seedlings, root, stem, leaf, flower, and leaf stalk using Trizol (Invitrogen) according to the manufacturer's instructions. First-strand cDNA was reverse transcribed from DNase I-treated RNA with oligo (dT) as the primer. Quantitative RT-PCR analyses were performed using the SYBR Green PCR Master Mix (Applied Biosystems) in an Mx3000P thermal cycler (Stratagene), and data were analyzed with MxPro software (Stratagene). The primers used are listed in . Quantitative RT-PCR was performed in a total volume of 10 µL containing 1 µL of the reverse-transcribed product, 0.2 µM of each primer and 5 µL of 1× SYBR Green PCR Master Mix (TaKaRa Co. Ltd.). The PCR protocol began with a denaturing step for 10 min at 95°C followed by 40 cycles of 15 s at 95°C, 30 s at 55°C and a primer extension reaction at 72°C for 30 s. All PCR reactions and negative controls were run in duplicate with three biological replicates for each one.
Table 1. RT-PCR primer sequences.
Physiological assays
For the proline accumulation assay, seeds were sowed onto half-strength MS medium without sucrose or other sugars (Verslues et al. Citation2006). Then, six-day-old seedlings were transferred to half-strength MS plates without sugar but with 10 µM ABA added to the medium. The Pro content was assayed using the ninhydrin-based colorimetric assay (Bates et al. Citation1973).
For the water loss experiment, the rosette leaves were removed from four- to five-week-old plants at the same developmental stage and size. Five leaves per genotype were excised. The fresh weight in detached leaves of ckl2 and Col-0 was measured in three independent experiments.
For the stomatal aperture assay, leaves were removed from five-week-old plants and floated in stomatal opening solution containing 50 mM KCl, 50 mM CaCl2, 10 mM MES (pH 6.15), and different concentrations of ABA (0, 1, or 10 µM). After incubation for 2.5 h, leaves were blended, and the stomatal aperture was measured. Data were expressed as the mean of three independent tests, and 100 stomata were measured for each test.
Results
The ckl2 mutant is selected by PCR screening
The homozygous mutant was identified by PCR screening (). PCR products were obtained only from reactions containing the LBpROK2 primer, which indicated that the T-DNA was forwardly and reversely inserted. The homozygous A, B, C, and D mutants were selected for further studies and collectively named ckl2. Sequencing of the T-DNA flanking region in ckl2 showed that the insertion was located 4139 nucleotides downstream from the ATG start codon (). The expression of the CKL2 gene was seriously impaired in ckl2 based on semi-quantitative RT-PCR ().
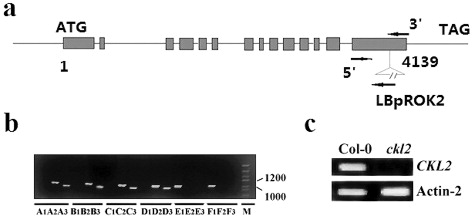
The ckl2 mutant is hyposensitive to ABA in seed germination
The progeny of homozygous ckl2 mutant lines were harvested, and many different assays were performed to test their sensitivity to ABA. The seed germination ratio was tested in MS solid medium containing different concentrations of ABA (0, 0.1, 0.3, 0.6 µM). In the absence of exogenous ABA, the germination ratio of ckl2 was similar to that of the wild-type plant (Col-0). However, in the presence of 0.6 µM ABA, the average seed germination percentage of ckl2 was higher than that of Col-0 on the third- to seventh-day (). The ckl2 mutant also showed hyposensitivity to NaCl (). In the presence of 150 mM NaCl, the average seed germination percentage of ckl2 was higher than that of Col-0 on the third- to seventh-day. In the presence of different concentrations of mannitol (0, 50, 150, 350 mM), there was no difference in germination ratio between the mutant and Col-0 ().
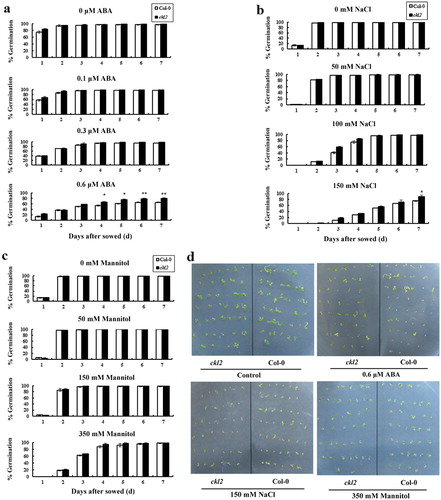
The ckl2 mutant is also hyposensitive to ABA in seedling root length and water loss analysis
When germination is complete, ABA can still regulate seedling growth. We then analyzed this aspect of ABA sensitivity in ckl2 by transferring five-day-old seedlings germinated on MS agar plates to the same medium with or without ABA. The length of the primary root was then measured 12 d later. The primary root length of ckl2 was found to be longer than that of Col-0 on medium containing 10 and 40 µM ABA ().
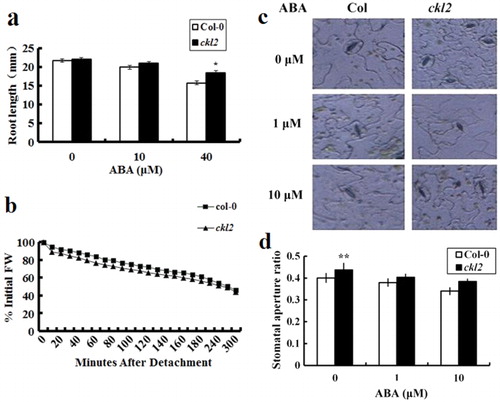
Next, the leaf water loss of ckl2 and Col-0 was examined. Detached leaves of both genotypes were measured every 10 min for 5 h (). The ckl2 mutant showed slight increases in leaf water loss, and these data suggest that CKL2 may have functions in stomatal control.
CKL2 is required for ABA-induced stomatal aperture
ABA can reduce water loss when plants are under water shortage conditions by regulating stomatal aperture. To further study the CKL2 functions in ABA signaling, specifically in regulating stomatal aperture, direct measurements of stomatal closing were performed (). Consistent with the previous results, stomata of ckl2 plants had larger stomatal apertures than those of Col-0 in the presence of 1 and 10 µM ABA.
CKL2 may be involved in ABA-induced changes in gene expression and proline accumulation
The expression of many genes that are induced by ABA is important for plants to adapt to stress. RESPONSIVE TO ABA18 (RAB18), RESPONSIVE TO DESICCATION29B (RD29B), RD22, and RD29A are examples of some well-documented ABA-upregulated genes. Quantitative RT-PCR was performed to examine the expression of these genes in ckl2 and Col-0. The ckl2 and Col-0 seedlings were treated with 100 µM ABA for 3 h, and we found that the expression of all of these genes were induced (). The induction of these genes was consistently less in ckl2. These ABA-upregulated genes are also expressed in seeds. We found that the expression of RD29A, RD29B, RAB18, and RD22 was greatly reduced in imbibed seeds of ckl2 ().
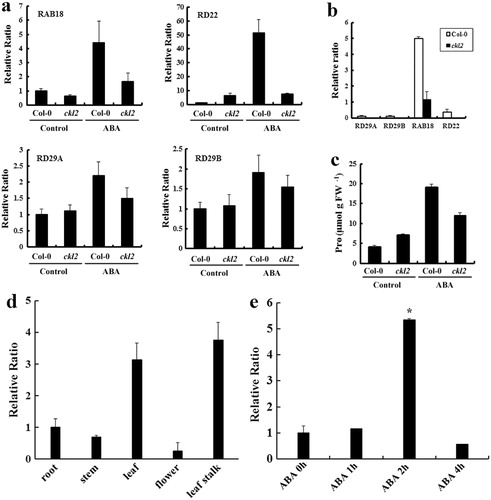
CKL2 also affected ABA-induced proline accumulation. Seedlings were exposed to 10 µM ABA for 96 h. Pro accumulation in ckl2 was found to be less than that in Col-0 ().
CKL2 is expressed in various tissues and can be induced by ABA
The gene expression profiles showed that CKL2 was expressed in all tissues of the wild-type plant, specifically in the leaf and leaf stalk (). The expression patterns for the CKL2 gene were observed under ABA stress. The result showed that a 10-µM ABA treatment induced an increase in the mRNA level of the CKL2 gene. The expression level of CKL2 was observed to be significantly high, two hours after treatment (). However, at four hours post-treatment, the expression level of CKL2 returned to normal.
Discussion
ABA, as an important plant hormone, can regulate many significant plant physiological processes, such as seed germination, dormancy, seedling growth, root growth, and stomatal aperture. Many genes have been reported to be involved in the ABA signaling pathway, but some key components are still unknown. CKL2, a CKL protein, may play a positive role in ABA signaling.
In this study, reverse genetics was used to study the function of CKL2 in ABA signaling. In a seed germination assay, a ckl2 mutant was found to be ABA-hyposensitive in seed germination, but there were no differences in the seed germination ratio between ckl2 and Col-0 treated with mannitol. These results indicate that the hyposensitivity of ckl2 plants to ABA in seed germination was not caused by osmotic stress. Rather, this hyposensitivity was caused by CKL2 as the protein kinase that positively regulates ABA signaling in seed germination.
Low concentrations of ABA (<1 µM) can stimulate root growth (Ephritikhine et al. Citation1999). In this study, high concentrations of ABA (10 and 40 µM) were used to measure root length. The ckl2 seedlings showed a reduced inhibition of root growth compared with that of wild-type plants. Therefore, identifying CKL2 as a new positive regulator of ABA signaling is a crucial step in understanding the physiological function of CKL2 in Arabidopsis. Furthermore, in the absence of exogenous ABA, the leaf water loss rate in ckl2 was faster than that of Col-0, and statistical data showed that CKL2 may have a function in guard cells. The stomatal aperture was measured in ckl2 and Col-0 in the presence or absence of exogenous ABA. We found that the stomatal aperture in ckl2 was larger than that of Col-0 in all of these tests. Thus, the mutant was found to be less sensitive to ABA, suggesting that CKL2 may act as a positive regulator of ABA signal transduction in stomatal closure. All of the phenotypic analysis of ckl2 and Col-0 indicated that CKL2 is a positive regulator in the ABA signaling pathway.
The differences in the expression level of the ABA-upregulated genes RAB18, RD29A, RD29B, and RD22 with or without ABA treatment in Col-0 were much more obvious than that of the ckl2 mutant line. This result indicated that CKL2 was responsible for ABA-regulated gene expression. Consistent with previous results, CKL2 could play a positive regulatory role in ABA signal transduction. The ABA-related genes contain ABA response elements (ABREs) in their promoters. RD29A and RD29B have ABREs in their promoters (Yamaguchi-Shinozaki & Shinozaki Citation1994; Yoshiba et al. Citation1999), and an ABRE was also found in the RAB18 promoter 300 bp upstream of the start codon (Fujii et al. Citation2007). Thus, CKL2 may affect the expression of these genes by phosphorylation of one or more ABRE binding factors. However, there is no distinct ABRE in the promoter region of RD22 (Abe et al. Citation1997). Interestingly, RD22 expression was also significantly reduced in ckl2. Thus, a question remains regarding whether this phenotype can be accounted for by a more direct, but as yet unknown, mechanism by which CKL2 can regulate gene expression independently of the ABRE or an indirect mechanism (i.e. that CKL2 regulates the expression of a gene that regulates RD22 expression).
ABA can induce proline accumulation in plants, but in ckl2, the accumulation of Pro was lower than that of Col-0 when under ABA stress. This result showed that CKL2 may be a positive regulator in the ABA signaling pathway.
CKL2 transcripts were expressed in various tissues of the plant, especially in the leaf and leaf stalk. The GENEVESTIGATOR tool (http://www.genevestigator.ethz.ch/) showed that the expression level of CKL2 was very high in each different growth period of Arabidopsis. This result indicated that CKL2 may not only have a function in the ABA signaling pathway but may also take part in many other signal transduction pathways. This study discovered a new member in ABA signaling and provided important clues to understand the additional CKL activities in ABA signal transduction pathways and plant development.
Acknowledgements
This research was supported by grants from the National Natural Science Foundation of China (31071076 and 30871325), the Program for New Century Excellent Talents in University (NCET-10-0363 to X. Guo), the Excellent Youth Foundation of Hunan Province (11JJ1005), the Project Sponsored by the Scientific Research Foundation for the Returned Overseas Chinese Scholars, the State Education Ministry ([2011]1139 to X. Guo), and the SIT Project of Hunan University, 2012 and 2013.
References
- Abe H, Yamaguchi-Shinozaki K, Urao T, Iwasaki T, Hosokawa D, Shinozaki K. 1997. Role of Arabidopsis MYC and MYB homologs in drought and abscisic acid-regulated gene expression. Plant Cell. 9:1859–68.
- Agostinis P, Pinna LA, Meggio F, Marin O, Goris J, Vandenheede JR, Merlevede W. 1989. A synthetic peptide substrate specific for casein kinase-1. FEBS Lett. 259:75–8. doi: 10.1016/0014-5793(89)81498-X
- Bates LS, Waldren RP, Teare ID. 1973. Rapid determination of free proline in water-stress studies. Plant Soil. 39:205–7. doi: 10.1007/BF00018060
- Behrend L, Stoter M, Kurth M, Rutter G, Heukeshoven J, Deppert W, Knippschild U. 2000. Interaction of casein kinase 1 delta (CK1 delta) with post-Golgi structures, microtubules and the spindle apparatus. Eur J Cell Biol. 79:240–51. doi: 10.1078/S0171-9335(04)70027-8
- Ben-Nissan G, Cui W, Kim DJ, Yang Y, Yoo BC, Lee JY. 2008. Arabidopsis casein kinase 1-like 6 contains a microtubule-binding domain and affects the organization of cortical microtubules. Plant Physiol. 148:1897–907. doi: 10.1104/pp.108.129346
- Cheong JK, Virshup DM. 2011. Casein kinase 1: complexity in the family. Int J Biochem Cell Biol. 43:465–9. doi: 10.1016/j.biocel.2010.12.004
- Dhillon N, Hoekstra MF. 1994. Characterization of two protein kinases from Schizosaccharomyces pombe involved in the regulation of DNA repair. EMBO J. 13:2777–88.
- Ephritikhine G, Fellner M, Vannini C, Lapous D, Barbier-Brygoo H. 1999. The sax1 dwarf mutant of Arabidopsis thaliana shows altered sensitivity of growth responses to abscisic acid, auxin, gibberellins and ethylene and is partially rescued by exogenous brassinosteroid. Plant J. 18:303–14. doi: 10.1046/j.1365-313X.1999.00454.x
- Etchegaray JP, Machida KK, Noton E, Constance CM, Dallmann R, DiNapoli MN, DeBruyne JP, Lambert CM, Yu EA, Reppert SM, Weaver DR. 2009. Casein kinase 1 delta regulates the pace of the mammalian circadian clock. Mol Cell Biol. 29(14):3853–66. doi: 10.1128/MCB.00338-09
- Fujii H, Verslues PE, Zhu JK. 2007. Identification of two protein kinases required for abscisic acid regulation of seed germination, root growth, and gene expression in Arabidopsis. Plant Cell. 19:485–94. doi: 10.1105/tpc.106.048538
- Graves PR, Roach PJ. 1995. Role of COOH2 terminal phosphorylation in the regulation of casein kinase I. J Biol Chem. 270:21689–94. doi: 10.1074/jbc.270.21.12717
- Kersten B, Agrawal GK., Iwahashi H, Rakwal R. 2006. Plant phospho-proteomics: a long road ahead. Proteomics. 6:5517–28. doi: 10.1002/pmic.200600232
- Knippschild U, Gocht A, Wolf S, Huber N, Lohle J, Stoter M. 2005. The casein kinase I family: participation in multiple cellular processes in eukaryotes. Cell Signal. 17:675–89. doi: 10.1016/j.cellsig.2004.12.011
- Lee H, Chen R, Lee Y, Yoo S, Lee C. 2009. Essential roles of CKI delta and CKI epsilon in the mammalian circadian clock. Proc Natl Acad Sci USA. 106:21359–64. doi: 10.1073/pnas.0906651106
- Lee JY, Taoka K, Yoo BC, Nissan GB, Kim DJ, Lucas WJ. 2005. Plasmodesmal-associated protein kinase in tobacco and Arabidopsis recognizes a subset of non-cell-autonomous proteins. Plant Cell. 17:2817–31. doi: 10.1105/tpc.105.034330
- Ogiso E, Takahashi Y, Sasaki T, Yano M, Izawa T. 2010. The role of casein kinase II in flowering time regulation has diversified during evolution. Plant Physiol. 152:808–20. doi: 10.1104/pp.109.148908
- Peters JM, Mckay RM, Mckay JP, Graff JM.. 1999. Casein kinase I transduces Wnt signals. Nature. 401:345–50. doi: 10.1038/43830
- Petronczki M, Matos J, Mori S, Gregan J, Bogdanova A, Schwickart M, Mechtler K, Shirahige K, Zachariae W, Nasmyth K. 2006. Monopolar attachment of sister kinetochores at meiosis I requires casein kinase 1. Cell. 126:1049–64. doi: 10.1016/j.cell.2006.07.029
- Price MA.. 2006. CKI, there's more than one: casein kinase I family members in Wnt and Hedgehog signaling. Gene Dev. 20:399–410. doi: 10.1101/gad.1394306
- Verslues PE, Agarwal M, Katiyar-Agarwal S, Zhu J, Zhu JK. 2006. Methods and concepts in quantifying resistance to drought, salt and freezing, abiotic stresses that affect plant water status. Plant J. 45:523–39. doi: 10.1111/j.1365-313X.2005.02593.x
- Wang M, Yu DS, Guo X, Cui Y, Li X, Zhang J, Zhao L, Chang H, Hu S, Zhang C, Shi J, Liu X. 2011. Casein kinase 1-Like 3 is required for abscisic acid regulation of seed germination, root growth, and gene expression in Arabidopsis. Afr J Biotechnol. 10(61):13219–29.
- Yamaguchi-Shinozaki K, Shinozaki K. 1994. A novel cisacting element in an Arabidopsis gene is involved in responsiveness to drought, low-temperature, or high-salt stress. Plant Cell. 6:251–64.
- Yoshiba Y, Nanjo T, Miura S, Yamaguchi-Shinozaki K, Shinozaki K. 1999. Stress-responsive and developmental regulation of Δ1-pyrroline-5-carboxylate synthetase 1 (P5CS1) gene expression in Arabidopsis thaliana. Biochem Biophys Res Commun. 261:766–72. doi: 10.1006/bbrc.1999.1112