Abstract
In order to understand the contributions of groundwater and deep soil water to the growth of halophytes in salinity-affected area, water use strategies of four shrubes, i.e. 20-year-old Tamarix ramosissima Ledeb., three-year-old T. ramosissima., Lycium barbarum L., and Atriplex canescens (Pursh) Nutt. were studied under contrasted water regimes in Northwest China. The result showed that there was a vertical gradient in soil δ18O and δD profiles resulted from evaporation and irrigation. The 20-year-old T. ramosissima mainly used water from middle (40–140 cm) and deep (140–200 cm) under both water regimes indicating its phreatophytic nature. Soil water in upper profile (0–40 cm) was the dominant water source for the three-year-old T. ramosissima before irrigation. After irrigation, the three-year-old T. ramosissima and L. barbarum switched their water sources to middle soil profile. Our experiment revealed phreatophytic tendency for the three-year-old A. canescens, which was not responsive to irrigation enlighten by photosynthetic parameters and stem water potentials.
Introduction
For nearly a century, the rooting depths and patterns of plants living in water-limited environments have been the ‘hot point’ for plant physiologists and ecologists. Plant functional type of root system is fundamental in determining their water use strategy and physiological responses to a specific water source (Xu & Li Citation2006). Different rooting patterns among species have resulted from long-period evolution and served as adaptations minimizing competition for water in a particular habitat (Dawson et al. Citation1993; Dawson & Pate Citation1996). The patterns of water uptake by woody species are summarized as either using only deep soil water or tapping both shallow and deep layers (Dodd et al. Citation1998; Goldstein et al. Citation2008; Guevara et al. Citation2010; Williams & Ehleringer Citation2000; West et al. Citation2007). On the basis of this finding, a terminology ‘phreatophyte’(the species that extract water from aquifers or the capillary fringe above the water table) has come into being and has been widely agreed in the academic field (Busch et al. Citation1992; Eggemeyer et al. Citation2009; Gries et al. Citation2003; Lite & Stromberg Citation2005; Lin et al. Citation1996; Sperry & Hacke Citation2002). According to the extent of this dependence, phreatophytes are classified as either ‘obligate’ (plants that utilize only shallow alluvial groundwater) or ‘facultative’ (plants that have the ability to utilize sources in addition to alluvial groundwater) (Busch et al. Citation1992; Horton et al. Citation2003). T. ramosissima has been listed as facultative phreatophytes, it utilizes only groundwater when this water source is shallow and relatively constant in depth, but is also able to extract water from unsaturated soil by its greater root allocation and physiological adaptability to a higher degree of water stress when groundwater is deeper and temporally more variable in depth (Busch et al. Citation1992; Busch & Smith Citation1995; Horton et al. Citation2001; Smith et al. Citation1998). However, the controversial documents are not scarce. Horton et al. (2003) did not find water use from the unsaturated soil by T. ramosissima grown in a serious of sites with a groundwater gradient from 0 to 4.4 m. Xu and Li (Citation2006) reported T. ramosissima relied mostly on groundwater for survival and did not show a significant photosynthetic response to sustained drought or heavy rain pulse event.
Atriplex canescens, known as xerohalophyte, has been reported to have deepest root indices, vulnerable shallow roots, and uniformly high ψ throughout the summer, suggesting preferential use of more stable deep soil water and phreatophytic tendency (Sperry & Hacke Citation2002).
The source regions of soil water uptake by plants have traditionally been difficult to assess (Ehrlinger & Dawson Citation1992) and excavation of roots to determine their spatial distribution is destructive and time consuming (Meinzer et al. Citation2001). With the development of stable isotope techniques, natural abundance of stable isotopic ratios of hydrogen (δD) and oxygen (δ18O) has been used as a tracer to determine the source of water extracted from soil by plant (Dawson et al. Citation1993). The isotopic composition of the soil water may vary with depth. This gradient is related to the isotopic composition of rain water that recharges the soil profiles, the isotopic composition of the groundwater, and the isotopic fractionation occurring during evaporation near the soil surface (Ehrlinger & Dawson Citation1992; Picon-Cochard et al. Citation2001). There is no fractionation of water isotopes during root water uptake and transport to the stem. Therefore, the water source of plants can be identified by simultaneously analyzing the isotopic composition of xylem sap and soil water (White et al. Citation1985; Picon-Cochard et al. Citation2001).
A large number of reports concentrating on the water source, water use strategy, and plant–water relations of plants using stable isotopic compositions have touched on several ecological systems: riparian areas (Dawson & Ehleringer Citation1991; Shafroth et al. Citation2000; Horton et al. Citation2003; Chimner & Cooper Citation2004; Lite & Stromberg Citation2005), desert regions (Sperry and Hacke Citation2002; Gries et al. Citation2003; Xu & Li Citation2006), woodland (Lin et al. Citation1996; Picon-Cochard et al. Citation2001), and grassland (Eggemeyer et al. Citation2009). The information about water use strategies of halophytes, Tamarix ramosissima Ledeb., Atriplex canescens (Pursh) Nutt., and Lycium barbarum L. in salinity- and alkalinity-affected regions is scarce. Furthermore, the reported researches were conducted at the sites where groundwater table was not beyond the root range of T. ramosissima. Under such circumstance, the conclusion could not be necessarily drawn that T. ramosissima does not use water from upper unsaturated soil.
Yinchuan Plain is located in the southeastern area of Huanghe Alluvial Plain. Huanghe River flows through Yinchuan Plain from southwest to northeast and widespread irrigation web has been well established (Chen et al. Citation2003). Soil salinity has plagued irrigated lands along the Yellow River in the northern area of Yinchuan Plain shortly after the first canal was built in 214 BC. Such factors, that is, high groundwater table mainly caused by over-irrigation, leaky canals, inadequate drainage system, the flatness of the terrain, and the high level of the Yellow River during the flood season, have contributed to the increase in soil salinity. Low rainfall and strong evaporation also aggravate this ecological problem in this area (Xiong et al. Citation1996).
In this work, we chose a stand consisting of 3-year-old and 20-year-old T. ramosissima for study the water use strategy of this species. We anticipated that the former one would take up more water in unsaturated soil when its root had not accessed the groundwater, while the latter would mostly rely on groundwater and not responsive to the fluctuating water regimes. The three-year-old Lycium barbarum L. and three-year-old A.canescens inside the stand were also included as check. Irrigation was applied once during the mid-summer for observing the change in water use of the shrubs tested in the contrasted water regimes. The specific objectives of this study are (1) to quantify water uptakes of the shrubs mentioned above from different soil profiles prior to and after irrigation by using D and 18O isotopic composition; (2) to evaluate their water use strategies by supplementary measurement of leaf gas exchange parameters and stem water potentials before and after irrigation.
Materials and methods
Site description
The study was conducted in Xidatan, Ningxia, China (1089 m in altitude, 106°30′9″ E, 38°52′33″N). Xidatan is located in the northern area of Yinchuan Plain. Flat terrain makes it is difficult for lands to drain water in this area, thus, salinity has arisen. The groundwater table is shallow with salt concentration from 1 g/kg to 3 g/kg. Mean annual rainfall is 172.5 mm, mean annual evaporation is 1755 mm. The groundwater table was 2.5–2.8 m during plant growth period (Zhu et al. Citation2012).
A stand consisting of three artificially established shrubs, that is, Tamarix ramosissima Ledeb., Lycium barbarum L., and Atriplex canescens (Pursh) Nutt., was established in 2008. Mature Tamarix ramosissima Ledeb. whose age was around 20 years were natively distributed nearby was involved for comparing the age's effect on water uptake of this species.
One hundred and twenty-millimeter irrigation was applied on 26 June. Irrigation water was pumped from a nearby well.
Water source sampling and analysis
Samples of well water, precipitation water, soil water from different depths, and groundwater were collected for hydrogen and oxygen isotopic analyses; samples were sealed in glass vials with screw caps and wrapped with parafilm. For determination of plant water source, five individuals were chosen at random. Fully suberized branches on the top of canopy were collected from each plant. The barks and green leaves were eliminated from branches, then the samples were immediately sealed in glass vials in the way described above. Rain water samples were collected after precipitation from May to July. Groundwater was sampled in monitoring wells and well water was sampled in a machine well. Samples of soil and stem were kept frozen at −20°C, while rainwater, well water, and groundwater samples were stored at 4°C until analysis for hydrogen and oxygen. Samples of soil water, groundwater, and plant water were collected on 28 May and 5 July. Ground-water depth was measured monthly in monitoring wells. Irrigation (120 mm) was applied three days before the second sampling.
For analyzing D and 18O compositions, water in soil and stem was extracted using cryogenic vacuum distillation (Horton et al. Citation2003). H2O was split into CO and H2 on the effect of catalyst in FLASHEA 1112HT (Thermo Scientific, Germany) isotopic analyzer. DELTA V isotope ratio mass spectrometer (Thermo Scientific, Germany) was used to analyze O in CO and H in H2 for obtaining the ratios 18O/16O and 2H/1H. The δD and δ18O values were expressed in parts per thousand (%) relative to the V-SMOW standard (Gonfiantini Citation1978).
To determine the fractional contribution of water sources to studied species, the IsoSource program was used (http://www.epa.gov/wed/pages/models/stableIsotopes/isosource/isosource.htm) (Phillips et al. Citation2005). Totally, there were 11 source endpoints, i.e. 0–5, 5–20, 20–40, 40–60, 60–80, 80–100, 100–120, 120–140, 140–160, 160–180, 180–200 cm, measured from 0 to 200 cm soil profile, which were too many to allow for feasible solutions. Therefore, we combined the data into three layers (0–40, 40–140, and 140–200 cm).
Soil water content and salinity
Volumetric soil water content was determined by time domain reflectometry technology (TDR Trime-T3, Ettlingen/Baden-Württemberg, Germany). For measuring volumetric water content, a probe was inserted into a 2-m plastic tube, buried vertically in the field in advance, and readings were recorded at intervals of 20 cm. Measurement was made at 28 May and 5 July. The soil water content is showed in .
Soil soluble salt content was analyzed by dissolving the soil samples into distilled water to achieve a soil solution, and then drying the solution to obtain the salt residue (Xu & Li Citation2006). Soil pH was determined by pH-3D pH meter (ZhiGuang Co. Ltd., Shanghai, China).
Leaf photosynthesis and stem water potentials
Leaf gas exchange rates were measured with a Ciras-2 infrared gas analyzer (PP System; Hitchin, Hertfordshire, UK) on leaves of representative shrubs covering an area of 1.7 cm2. Leaves were maintained at right angles to intercept solar radiation. Net photosynthesis (Pn), transpiration rate (Tr), and stomatal conductance (gs) were provided by the apparatus. Three measurements were made per shrub. After measurement, the spinal leaves of Tamarix ramosissima Ledeb. were collected in plastic bags for measuring leaf area by using Photoshop 6.0. Pn, Tr, and gs were revised according to the ratio of leaf area to chamber area of the apparatus. Transpiration efficiency was calculated as Pn/Tr.
Predawn (Bar; 1 h before sunrise) and midday (Bar; 11:30–12:30 h) water potentials were measured for assessing plant water relations. Measurements were made on 28 May (before irrigation) and 4 July (after irrigation). Water potential was determined with a pressure chamber (PMS Instrument Co., Albany, OR, USA) on tree twigs collected from the same individuals that were used for stable isotope measurements.
Statistical analysis
The effects of irrigation treatments and differences between species were tested with factorial ANOVA analyses (GLM procedure of the SAS software package, SAS Institute, Cary, NC, USA), where the F-values were considered statistically significant at the 0.05 level. Pearson phenotypic correlations were calculated to determine the relationship between δ18O and δD values, water content, salt content, and pH in soil profile.
Result
Environmental parameters
Total precipitation for the study period was 112.1 mm. No effective rain occurred in April. Monthly rainfall was 45.9 mm in May and 49 mm in June (). Soil water content increased with depth. Highest soil water content was observed in the 160–180 cm profile. The highest soluble salt content was recorded in the 100–120 cm profile. The upper and deep profiles were characterized by lower soluble salt content. The salt content of groundwater was 1.48 g/L before irrigation and 1.97 g/L after irrigation. The pH values ranged from 7.1 to 8.8 before irrigation and 7–8.3 after irrigation. Higher pH was noted in the profile below 60 cm before irrigation and in the 20–80 cm profile after irrigation. Irrigation had significant effect on water and soluble salt contents in the 0–140 cm profile ().
Isotope compositions of different water sources and xylem water of different shrubs
The δ18O of well water was more negative than groundwater. The isotopic composition in the upper profile (<80 cm) fluctuated temporally and spatially. The δ18O values were lower in the deep soil profile (below 140 cm). In the soil profile from 180 to 200 cm, δ18O values of soil water were close to those of groundwater. Generally, isotopic compositions of groundwater and soil water significantly decreased after irrigation except for a sudden increase in the 80–100 cm profile (). The fractionation processes in different water sources and xylem water of shrubs were evaluated by a δ18O–δD graph (). Results showed that almost all the samples of soil water and groundwater plotted at the right side of the local meteoric water line in arid area of Northwest China (ANC LMWL) with the latter plotting neared to the LMWL than the former, while the well water plotted on the LMWL indicating the effect of evaporation on different water sources. Lower δ18O and δD values were found for 20-year-old T. ramosissima than the three-year-old shrubs. After irrigation, the δ18O and δD of four shrubs decreased to some extent ().
Relationship among δD, δ18O, soil water content, salt concentration, and pH
Significant and positive correlation between δD and δ18O were recorded regardless of irrigation or not. Soil water content was related positively with soil water δD and δ18O before irrigation. Soil salt concentration correlated positively with soil water δD and δ18O after irrigation. Negative relationship was found between soil pH and values of δD and δ18O before irrigation ().
Table 1. Relationship among soil water hydrogen and oxygen composition, soil water content, soil salt content and pH.
Water uptake of different water sources for four shrubs
A three-source mixing model was used to determine the fraction of water acquired by shrubs from three ranges of soil depths (0–40 cm, 40–140 cm, and 140–200 cm). As shown by the model, before irrigation, the three-year-old shrubs, i.e. T. ramosissima, L. barbarum, and A. canescens, mainly used soil water from 0 to 40 cm soil profile. The 20-year-old T. ramosissima mostly extracted moisture in the middle and deep soil profiles (below 40 cm). After irrigation, the three-year-old T. ramosissima and L. barbarum obtained most of their water from the 40–140 cm soil profile. Highest dependence on soil moisture in deep soil profile (140–200 cm) was observed for 20-year-old T. ramasissima. Among the three-year-old shrubs, the A. canescens exhibited higher water uptake percent from deep soil profile than the other two shrubs regardless of irrigation or not ().
Table 2. Water uptake rate of potential sources for four shrubs in Xidatan (mean (minimum–maximum)).
Photosynthetic gas exchange parameters xylem water potentials of different shrubs
The net photosynthetic rate (Pn) of the three-year-old L. barbarum L. was higher than other three shrubs regardless of irrigation or not (). Generally, irrigation exerted significant influence on Pn, gs, and Tr of the three-year-old shrubs T. ramosissima and L. barbarum L. and on Pn of the 20-year-old T. ramosissima (). No significant response of the three-year-old A. canescens in terms of photosynthetic parameters to irrigation was found (). Highest gs and transpiration rate (Tr) were found for the three-year-old L. barbarum L. after irrigation. The 20-year-old T. ramosissima displayed the highest Tr before irrigation. The three-year-old A. canescens exhibited the lowest gs and Tr and the highest intrinsic water use efficiency (WUE) no matter being irrigated or not (). A slight and significant decrease in Pn/Tr was noted for the three-year-old L. barbarum L. after applying irrigation ().
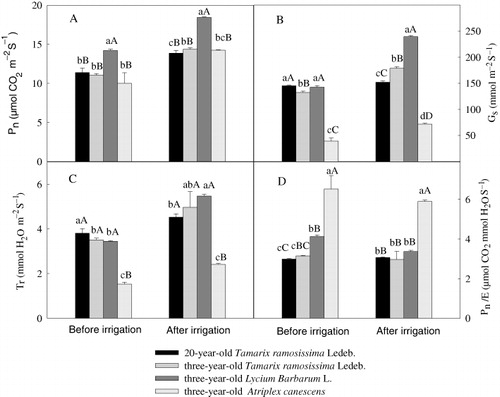
Before irrigation, twig predawn water potential (ψpre) differed among species. The 20-year-old T. ramosissima displayed the highest ψpre. The lowest ψpre was recorded for three-year-old A. canescens regardless of whether irrigation was applied or not (). Irrigation had significant effects on ψpre and twig midday water potential (ψmid) of three-year-old T. ramosissima and ψmid of three-year-old L. barbarum and A. canescens. No significant effect on twig water potentials of 20-year-old T. ramosissima was noted ().
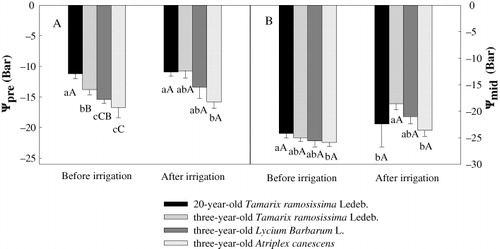
Discussion
Using stable isotopes to determining the water sources for plant transpiration depends on sources of water having different endogenous isotopic compositions (Eggemeyer et al. Citation2009). The seasonal input of moisture into the soil, evaporation in the uppermost surface layers, or differences between bulk soil moisture and groundwater contribute to the isotopic composition of water within soils (Ehrlinger & Dawson Citation1992). We observed a vertical gradient in soil water δ18O profiles (). The δ18O and δD values in 0–80 cm soil profile fluctuated indicating the multiple influences of the input of irrigational water, precipitation, and evaporation. A negative correlation between soil water content and values of δ18O and δD in 0–200 cm soil profile () indicated the significant effects of evaporation and irrigational event (well water being δ18O and δD-depleted) on the soil isotopic compositions. Slight more negative δ18O values were found in deep soil profiles than groundwater, reflecting the combinational effects of irrigations applied in current or previous seasons and the recharge of groundwater to the corresponding soil profiles.
Variations in depth of water uptake for different shrubs before irrigation
Prior to irrigation, the three-year-old shrubs mostly extracted water from upper profile (0–20 cm), while the 20-year-old T. ramosissima mainly used soil water in the middle and deep profiles, reflecting the effects of species and age on the water use strategy of shrubs tested. The three-year-old T. ramosissima was recorded by more negative water potential suggesting it was experiencing strong water stress due to low water content in the upper soil profiles. Presumably, the roots of three-year-old T. ramosissima have not been developed enough to access water in the deeper soil layers during seedling stage, its growth would highly depend on moisture in the upper soil profile through greater capacity in osmotic adjustment (Busch & Smith Citation1995; Stromberg et al. Citation1996; Horton et al. Citation2003). In contrast, the 20-year-old T. ramosissima mostly used deeper soil water by developing active absorbing roots at depths close to the groundwater table (Xu & Li Citation2006).
Sperry and Hacke (Citation2002) reported that A. Canescens exhibited phreatophytic tendency and had deepest root indices and vulnerable shallow root (Lin et al. Citation1996). Lin et al. (Citation1996) observed A. canescens experienced negative predawn and midday water potentials that were not relieved by summer rain indicating less uptake of rain. In our study, higher uptake rate from deep soil profile, and less responses of photosynthetic activity and plant water relations to irrigation for the A. canescens were consistent with the above reports.
Higher leaf intrinsic water use efficiency (Pn/Tr) of A. canescens agreed with the previous reports that A. canescens has C4 photosynthetic path allowing it to remain photosynthetic activity with a greater water use efficiency (Lin et al. Citation1996).
Variations in depth of water uptake for different shrubs after irrigation
After irrigation, a significant decrease in the values of δD and δ18O above 80 cm soil profile suggested the infiltration of well water (with more negative isotopic composition). Significant and positive correlations between soil salt concentration and values of δD and δ18O (r=0.58, 0.59) were observed (). This may be attributed to two aspects of the fact: firstly, the δD and δ18O-depleted irrigated water would leach soil salt to the deeper layer and lower the isotopic compositions in the corresponding soil profile; secondly, the evaporation would cause increase in salt concentration and isotopic enrichment in the upper soil profile.
To be unexpected, the highest values of δD and δ18O appeared in the 80–100 cm soil profile. This implied that a ‘piston flow’ might happened in soil profile after irrigation (Tang & Feng Citation2001; Eggemeyer et al. Citation2009) and the replacement of ‘old soil water’ with new infiltrating water would recompose the soil isotopic profile. Presumably, being pushed by irrigational water, the upper soil water (δD and δ18O-enriched) would move downwardly and stop at a certain soil layer, around 100 cm in depth, when the hydraulic gradient exhausted, enhancing the isotopic composition of this layer. A redistribution of the soil salt concentration in the whole soil profile after irrigation was noted, with and inflexion at depth of 100 cm, suggesting an obvious leaching effect on salt concentration profile.
Since the irrigation events occurred frequently in the neighbor fields (mostly paddy field) during our second sampling time (4 July), the isotopic compositions of groundwater, being recharged by the δD and δ18O-depleted well water, were more negative than before irrigation. The values of δD and δ18O in the deep profile that approached to groundwater also decreased on 4 July compared with 28 May, which may be accounted for by the effect of capillarity in the area above the aquifer.
In this study, the highest deep soil water uptake percentage was observed for the 20-year-old T. ramosissima. No irrigation effect was found on its Pn, gs, Tr, ψpre, ψmid of uncovering some physiological bases in relation to its phreatophytic nature (Cleverly et al. Citation1997; Xu & Li Citation2006). Compared with the 20-year-old T. ramosissima, the three-year-old T. ramosissima depended heavily on soil water in the middle profile (80–140 cm), implying the age effect on water use strategy of this species. Considering the root of T. ramosissima during seedling stage did not approach groundwater layer, it would mainly absorbed moisture from the middle profile where water content was high after irrigation (). This fact also reflected the flexible water use strategy of this species. Our experimental result partly corrected some findings of previous reports that T. ramosissima only used deep soil water on the ground that this species mostly uses groundwater during mature stage and use other water sources when groundwater is beyond of its root range.
In the present study, A. canescens used more water in the deep profile after applying irrigation, revealing its phreatophytic tendency and deep root distribution (Sperry & Hacke Citation2002). The non-significant increment in gs and Tr for A. canescens after irrigation suggests this plant relies mainly on stable water source – groundwater and remains unresponsive to irrigation.
Conclusion
A vertical gradient in soil water δ18O profiles was observed. Evaporation and irrigation have exerted significant effects on soil water oxygen and hydrogen compositions in soil profiles. The three-year-old Tamarix ramosissima Ledeb. exhibited flexible water use strategy: utilize water from upper unsaturated soil profile during dry period before irrigation and switched its water use to middle soil profile after irrigation. The 20-year-old T. ramosissima heavily depended on middle and deep soil water and was unresponsive to irrigation event. Considering the performances of T. ramosissima in different ages, this species is facultative phreatophyte using multiple water sources.
Acknowledgements
This study has been financially supported by the National Natural Science Foundation of China (31160478), National Key Technology Research and Development Program of China (2011BAC07B03), Key Project of Chinese Ministry of Education (211195), Open Fund of State Key Laboratory of Soil Erosion and Dryland Farming on the Loess Plateau (10501-287), Ningxia Natural Science Fund (NZ1142), The National Program on Key Basic Research Project of China (2012CB723206). The authors are thankful to the National Natural Science Foundation Committee, Ministry of Science and Technology of China, State Key Laboratory of Soil Erosion and Dryland Farming on the Loess Plateau, Institute of Water and Soil Conservation, Chinese Academy of Sciences and Ministry of Water Resources, for financial support.
References
- Busch DE, Ingraham NL, Smith SD. 1992. Water uptake in woody riparian phreatophytes of the southwestern United States: a stable isotope study. Ecol Appl. 2:450–9. doi: 10.2307/1941880
- Busch DE, Smith SD. 1995. Mechanisms associated with decline of woody species in riparian ecosystems of the southwestern US. Ecol Monogr. 65:347–70. doi: 10.2307/2937064
- Chen J, He D, Cui S. 2003. The response of river water quality and quantity to the development of irrigated agriculture in the last 4 decades in the Yellow River Basin, China. Water Resour Res. 39:1047–57. doi: 10.1029/2001WR001234
- Chimner RA, Cooper DJ. 2004. Using stable oxygen isotopes to quantify the water source used for transpiration by native shrubs in the San Luis Valley, Colorado USA. Plant Soil. 260:225–36. doi: 10.1023/B:PLSO.0000030190.70085.e9
- Cleverly JR, Smith SD, Sala A, Devitt DA. 1997. Invasive capacity of Tamarix ramosissima in a Mojave Desert floodplain: the role of drought. Oecologia. 111:12–8. doi: 10.1007/s004420050202
- Dawson TE, Ehleringer JR. 1991. Streamside trees that do not use stream water. Nature. 350:335–7. doi: 10.1038/350335a0
- Dawson TE, Ehleringer JR, Hall AE, Farquhar GD. 1993. Water sources of plants as determined from xylem-water isotopic composition: perspectives on plant competition, distribution, and water relations. San Diego, CA: Academic Press, Inc.
- Dawson TE, Pate JS. 1996. Seasonal water uptake and movement in root systems of Australian phreatophytic plants of dimorphic root morphology: a stable isotope investigation. Oecologia. 107:13–20. doi: 10.1007/BF00582230
- Dodd M, Lauenroth W, Welker J. 1998. Differential water resource use by herbaceous and woody plant life-forms in a shortgrass steppe community. Oecologia. 117:504–12. doi: 10.1007/s004420050686
- Eggemeyer KD, Awada T, Harvey FE, Wedin DA, Zhou X, Zanner CW. 2009. Seasonal changes in depth of water uptake for encroaching trees Juniperus virginiana and Pinus ponderosa and two dominant C4 grasses in a semiarid grassland. Tree Physiol. 29:157–9. doi: 10.1093/treephys/tpn019
- Ehrlinger JR, Dawson TE. 1992. Water uptake by plants: perspectives from stable isotope composition. Plant Cell Environ. 15:1073–82. doi: 10.1111/j.1365-3040.1992.tb01657.x
- Goldstein G, Meinzer FC, Bucci SJ, Scholz FG, Franco AC, Hoffmann WA. 2008. Water economy of Neotropical savanna trees: six paradigms revisited. Tree Physiol. 28:395–404. doi: 10.1093/treephys/28.3.395
- Gonfiantini R. 1978. Standards for stable isotope measurements in natural compounds. Nature. 271:534–6. doi: 10.1038/271534a0
- Gries D, Zeng F, Foetzki A, Arndt SK, Bruelheide H, Thomas FM, Zhang X, Runge M. 2003. Growth and water relations of Tamarix ramosissima and Populus euphratica on Taklamakan desert dunes in relation to depth to a permanent water table. Plant Cell Environ. 26:725–36. doi: 10.1046/j.1365-3040.2003.01009.x
- Guevara A, Giordano CV, Aranibar J, Quiroga M, Villagra PE. 2010. Phenotypic plasticity of the coarse root system of Prosopis flexuosa, a phreatophyte tree, in the Monte Desert (Argentina). Plant Soil. 330:447–64. doi: 10.1007/s11104-009-0218-4
- Horton JL, Hart SC, Kolb TE. 2003. Physiological condition and water source use of Sonoran Desert riparian trees at the Bill Williams River, Arizona, USA. Isot Environ Healt S. 39:69–82. doi: 10.1080/1025601031000096772
- Horton JL, Kolb TE, Hart SC. 2001. Leaf gas exchange characteristics differ among Sonoran Desert riparian tree species. Tree Physiol. 21:233–41. doi: 10.1093/treephys/21.4.233
- Lin GH, Phillips SL, Ehleringer JR. 1996. Monosoonal precipitation responses of shrubs in a cold desert community on the Colorado Plateau. Oecologia. 106:8–17.
- Lite SJ, Stromberg JC. 2005. Surface water and ground-water thresholds for maintaining Populus-Salix forests, San Pedro River, Arizona. Biol Conserv. 125:153–67. doi: 10.1016/j.biocon.2005.01.020
- Meinzer FC, Clearwater MJ, Goldstein G. 2001. Water transport in trees: current perspectives, new insights and some controversies. Environ Exp Bot. 45:239–62. doi: 10.1016/S0098-8472(01)00074-0
- Phillips DL, Newsome SD, Gregg JW. 2005. Combining sources in stable isotope mixing models: alternative methods. Oecologia. 144:520–7. doi: 10.1007/s00442-004-1816-8
- Picon-Cochard C, Nsourou-Obame A, Collet C, Guehl JM, Ferhi A. 2001. Competition for water between walnut seedlings (Juglans regia) and rye grass (Lolium perenne) assessed by carbon isotope discrimination and δ18O enrichment. Tree Physiol. 21:183–91. doi: 10.1093/treephys/21.2-3.183
- Shafroth PB, Stromberg JC, Patten DT. 2000. Woody riparian vegetation response to different alluvial water table regimes. Western North American Naturalist. 60:66–76.
- Smith SD, Devitt DA, Sala A, Cleverly JR, Busch DE. 1998. Water relations of riparian plants from warm desert regions. Wetlands. 18:687–96. doi: 10.1007/BF03161683
- Sperry J, Hacke U. 2002. Desert shrub water relations with respect to soil characteristics and plant functional type. Funct Ecol. 16:367–78. doi: 10.1046/j.1365-2435.2002.00628.x
- Stromberg J, Tiller R, Richter B. 1996. Effects of groundwater decline on riparian vegetation of semiarid regions: the San Pedro, Arizona. Ecol Appl. 6:113–31. doi: 10.2307/2269558
- Tang K, Feng X. 2001. The effect of soil hydrology on the oxygen and hydrogen isotopic compositions of plants' source water. Earth Plan Sci Lett. 185:355–67. doi: 10.1016/S0012-821X(00)00385-X
- West AG, Hultine KR, Burtch KG, Ehleringer JR. 2007. Seasonal variations in moisture use in a piñon–juniper woodland. Oecologia. 153:787–98. doi: 10.1007/s00442-007-0777-0
- White JWC, Cook ER, Lawrence JR, Wallace S. 1985. The D/H ratios of sap in trees: implications for water sources and tree ring D/H ratios. Geochim Cosmochim Acta. 49:237–46. doi: 10.1016/0016-7037(85)90207-8
- Williams DG, Ehleringer JR. 2000. Intra-and interspecific variation for summer precipitation use in pinyon-juniper woodlands. Ecol Monogr. 70:517–37.
- Xiong SY, Xiong ZX, Wang PW. 1996. Soil salinity in the irrigated area of the Yellow river in Ningxia, China. Arid Land Res Manag. 10:95–101.
- Xu H, Li Y. 2006. Water-use strategy of three central Asian desert shrubs and their responses to rain pulse events. Plant Soil. 285:5–17. doi: 10.1007/s11104-005-5108-9
- Zhu L, Xu X, Mao GL. 2012. Water sources of shrubs grown in the northern Ningxia Plain of China characterized by shallow groundwater table. Chin J Plant Ecol. 36(7):618–28.