Abstract
The aim of this study was to determine the effect of salicylic acid (SA) on nitrogen fixation and assimilation under conditions of cadmium stress in chickpea plants. Chickpea seeds were sown in pots containing 0, 25, 50, or 100 mg of cadmium per kilogram of soil. The foliage of the 30-day-old plants was sprayed with 10−5 M SA, and the activities of nitrogenase, nitrate reductase, glutamine synthetase, glutamate synthase, and glutamate dehydrogenase were investigated. SA exposure increased the number of nodules, fresh and dry nodule masses, leghemoglobin content, and activity of the nitrogen-fixing enzyme nitrogenase compared with the control conditions. Furthermore, SA application enhanced the activities of the enzymes involved in nitrogen assimilation, in both the control and cadmium-stressed plants. The overall results indicate that SA increases the fixation and assimilation of nitrogen regardless of whether the plants are grown in the presence or absence of cadmium.
Abbreviations | ||
ATP | = | adenosine triphosphate |
DM | = | dry mass |
DAS | = | days after sowing |
EDTA | = | ethylenediaminetetraacetic acid |
FM | = | fresh mass |
GS | = | glutamine synthetase |
GOGAT | = | glutamate synthase |
GDH | = | glutamate dehydrogenase |
NR | = | nitrate reductase |
SA | = | salicylic acid |
γGH | = | γ-glutamyl hydroxamate. |
1. Introduction
The soils in many parts of the world are slightly to moderately contaminated by heavy metals, such as Cd, Cu, Zn, Ni, Co, Cr, Pb, and As, possibly as a result of the long-term use of phosphate fertilizers, the application of sewage sludge, and the presence of industrial waste and dust from smelters (Passariello et al. Citation2002). Because of its solubility, Cd is one of the most toxic and mobile heavy metals present in contaminated crop environments and has been ranked No. 7 among the top 20 toxins (Yang et al. Citation2004). Cd is easily taken up by plants and accumulates to high levels in the aerial organs (Kabata-Pendias & Pendias Citation2001), thereby potentially causing cellular injury. Cadmium preferentially accumulates in the chloroplasts and disrupts chloroplast function by damaging the membrane and inhibiting the biosynthesis of chlorophyll and the CO2 fixation activity (Siedlecka et al. Citation1997). In addition, various physiological processes can be altered, including growth (Ekmekci et al. Citation2008; Hasan et al. Citation2008; Krantev et al. Citation2008) and plant–water relationships (Barcelo & Poschenrieder Citation1990). The Cd-induced generation of superoxide () anions, hydroxyl (OH−) radicals, and H2O2 causes a considerable amount of membrane damage by inducing the peroxidation of polyunsaturated fatty acids (De Vos & Schat Citation1981) and altering the activity of antioxidative enzymes (Hasan et al. Citation2008). Cadmium directly or indirectly interferes with the uptake of nutrients by plants because Cd generally competes for uptake by plant roots at the same absorption sites as heavy metals and mineral nutrients such as Ca2+ and Mg2+, which have chemical properties similar to those of Cd (Jarvis et al. Citation1976). Cadmium toxicity is known to decrease nodulation and the activity of nitrogen-metabolizing enzymes, thereby decreasing the ability of the plants to fix nitrogen (Hasan et al. Citation2008).
Salicylic acid (SA) is an endogenous plant growth regulator that acts as a signal in the induction of specific plant responses to biotic and abiotic stresses. SA is involved in the protection of plants against multiple stresses, including freezing, salinity, ozone, ultra-violet radiation, water stress, drought stress (Hayat et al. Citation2010; Hussain et al. Citation2011; Habibi Citation2012; Patel & Hemantaranjan, Citation2012), and herbicides (Ananieva et al. Citation2004). Indeed, there are many reports that show that SA can ameliorate the injurious effects of heavy metals on plants (Hayat et al. Citation2010; Zhang & Chen Citation2011). Owing to the considerable evidence of the adverse effects of Cd on normal plant development and the protective role of SA during stress, it was hypothesized that SA can mitigate the injurious effects of Cd on nitrogen metabolism in chickpea. Therefore, the objective of this work was to determine whether SA could improve the efficiency of nitrogen fixation and assimilation in chickpea plants exposed to varying doses of soil-applied Cd.
2. Materials and methods
2.1. Plant material and treatments
The seeds of Cicer arietinum L. cv. Avarodhi were surface-sterilized with 0.01% mercuric chloride solution, inoculated with Rhizobium, and sown in five sets of earthen pots (10-inch diameter) filled with sandy loam soil and farmyard manure (6:1). These pots were then arranged in a simple randomized block design. Of these five sets of pots, four sets were supplemented with different doses (0, 25, 50, or 100 mg per kg of soil) of Cd, and one set of pots was left untreated, serving as the control. The foliage of 30-day-old plants was sprayed with 10−5 M SA, except for the control plants, which received double-distilled water (DDW). The concentration of SA was selected on the basis of our previous experiments (Hayat Citation2010). The plant samples were collected at 60 and 90 days after sowing (DAS) for the assessment of various parameters.
2.2. Nodule number and fresh and dry masses
The nodules from each plant were picked and counted to determine the number of nodules per plant. The nodules were subsequently weighed to obtain their fresh mass. These nodules were then transferred to Petri dishes for overnight drying in an 80°C oven to obtain their dry mass.
2.3. Nodule leghemoglobin content
The leghemoglobin content of the fresh nodules was estimated following the method described by Sadasivam and Manickam (Citation1992). Fresh nodules (200 mg) were mixed with 3 ml of 0.1 M phosphate buffer and macerated in a mortar and pestle, followed by filtration through two layers of cheesecloth. The nodule debris was discarded, and the turbid reddish-brown filtrate was centrifuged at 10,000×g for 10–30 minutes. A 3 ml aliquot of alkaline pyridine reagent was added to 3 ml of nodule extract and mixed well; the solution became greenish yellow due to the formation of hemochrome. The hemochrome was divided equally into two test tubes. To one test tube, a few crystals of potassium hexacyanoferrate were added to oxidize the hemochrome, and the absorbance was measured at 539 nm using a spectrophotometer (Spectronic 20D, Milton Roy, USA). To the other test tube, a few crystals of sodium dithionite were added to reduce the hemochrome, and the absorbance of this mixture was measured at 556 nm after an interval of 2–5 minutes. The leghemoglobin content (mM) was calculated using the following formula:
2.4. Nodule nitrogenase activity
The nitrogenase activity was assayed using the procedure of Hardy et al. (Citation1968). The assay was performed immediately after harvesting the plants. The nodulated roots were cut from the base and shaken slowly in water to remove the attached soil particles. The samples were assayed in 30-ml glass tubes sealed with a subseal to allow piercing with a hypodermic needle. Five milliliters (v/v) of air was withdrawn from the sample container and replaced with an equal volume of acetylene gas. After an incubation of 1 h at room temperature, 0.5 ml of gas withdrawn from the sample tube was injected into a gas chromatograph (Nucon Series 5500) equipped with a flame ionization detector to detect ethylene gas. The results are expressed in terms of nanomoles of ethylene formed/gram nodule fresh mass/hour.
2.5. Root nitrate content
The nitrate content in the roots was estimated following the method of Singh (Citation1988). The root powder was macerated with acetic acid and was reacted with a powdered mixture containing citric acid, manganese sulfate monohydrate, sulfanilamide, N-1-naphthyl-ethylenediamine hydrochloride, and zinc. The absorbance of the reaction mixture was measured at 540 nm, and the absorbance of each sample was compared with that of the calibration curve constructed using potassium nitrate. The nitrate content was computed on a dry mass basis.
2.6. Nitrate reductase (NR) activity
The activity of NR was measured following the method of Jaworski (Citation1971). A mixture of fresh leaf samples, phosphate buffer (pH 7.5), KNO3, and isopropanol was incubated at 30°C for two hours. Sulfanilamide and N-1-naphthyl ethylenediamine hydrochloride were added to the incubated mixture, and the absorbance was measured at 540 nm using a spectrophotometer.
2.7. Nitrogen content
The nodule nitrogen content was estimated by employing the method of Lindner (Citation1944). The plant material was digested with concentrated H2SO4, followed by neutralization with NaOH and sodium silicate solutions. Nessler's reagent was added to this solution, and the samples were measured using a spectrophotometer.
2.8. Nodule carbohydrate content
The carbohydrates were extracted from the samples following the method of Yih and Clark (Citation1965), and the concentrations were estimated by adopting the procedure of Dubois et al. (Citation1956).
2.9. Glutamine synthetase (GS), glutamate synthase (GOGAT), and glutamate dehydrogenase (GDH) activity assays
The extraction and evaluation of GS, GOGAT, and GDH were performed following the methods described by Thimmaiah (Citation1999). The extract used for the determination of the GS activity was prepared from nodules homogenized in an extraction buffer containing potassium phosphate buffer, sucrose, dithiothreitol, KCl, MgCl2, and EDTA. A 50 ml aliquot of this enzyme extract was added to a reaction mixture containing Tris-maleate buffer, hydroxylamine, L-glutamine, ATP, and EDTA. The absorbance was measured at 540 nm using a spectrophotometer and was compared with a calibration curve constructed using pure γ-glutamyl hydroxamate. The extract used for the determination of the GOGAT and GDH activities was prepared from nodules homogenized in extraction buffer containing Tris–HCl, sucrose, and β-mercaptoethanol. Aliquots of 1 ml of this extract were added to reaction mixtures consisting of Tris–HCl buffer, 2-oxoglutarate, NADH, and either NH4Cl or L-glutamine to assess the GOGAT and GDH activities, respectively. The absorbance was measured at 340 nm using a UV spectrophotometer (Elico, India).
2.10. Data analysis
Each observation was replicated three times, and the treatment means were compared using an analysis of variance with SPSS software version 10 (SPSS, Chicago, IL, USA). The least significant difference (LSD) was calculated at a 5% level of probability. The standard error (SE) of the replicates was also calculated.
3. Results
All of the parameters studied followed a similar trend at both of the sampling times. The magnitude of the data for these parameters was higher at 90 DAS, so the data at this stage are presented herein.
3.1. Nodulation
The exogenous application of 10−5 M SA increased the number of nodules by 58.0% in the unstressed plants and by 24.0% in the plants exposed to 25 mg Cd per kg of soil compared with the control (). Moreover, after the application of SA to the plants grown in the presence of 50 mg Cd per kg of soil, the number of nodules was 14.0% higher than that of the control. The exogenous application of SA also resulted in an increase of 59.5% (unstressed plants), 38.1% (25 mg Cd), and 12.0% (50 mg Cd) in the nodule dry mass compared with the control (). However, the plants treated with 100 mg Cd and SA had lower values for both of the above parameters than did the control.
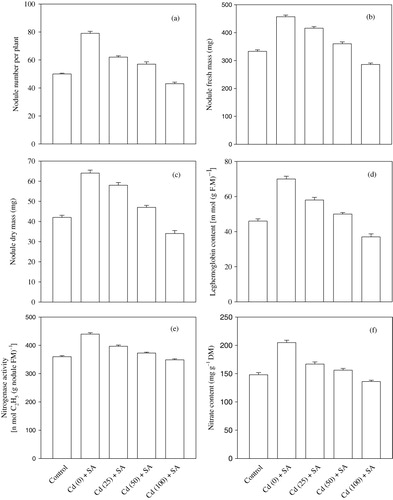
3.2. Leghemoglobin content in nodules
The application of SA to the nonstressed and stressed (25 mg per kg of soil) plants improved the leghemoglobin content in the nodules by 52.2% and 26.1%, respectively, over the control (). The plants grown with 50 mg Cd that were treated with SA exhibited a nodule leghemoglobin value that was statistically equal to that of the control. In contrast, SA was unable to overcome the impact of 100 mg Cd per kg of soil.
3.3. Nitrogenase activity in nodules
The foliar application of 10−5 M SA enhanced the nitrogenase activity by 22.2% (unstressed plants), 10.3% (25 mg Cd), and 3.6% (50 mg Cd) over that of the control (). Like other parameters, the nitrogenase activity in plants exposed to 100 mg Cd per kg of soil was not improved by the foliar application of SA ().
3.4. Nitrate content in roots
The foliar application of 10−5 M SA increased the nitrate content in the roots by 38.51% (unstressed), 12.8% (25 mg Cd), and 5.4% (50 mg Cd) over that of the control (). Conversely, the nitrate content of the roots of the plants supplemented with 100 mg Cd and sprayed with SA was found to be 8.1% lower than that of the control.
3.5. NR activity in leaves
The highest NR activity was observed in the unstressed plants sprayed with SA (). The activity of NR increased under the influence of SA but decreased with an increasing concentration of the metal in the Cd-stressed plants. The increase in the activity of this enzyme was found to be 27.5% (unstressed), 11.5% (25 mg Cd), and 5.6% (50 mg Cd) relative to the control. The 100 mg Cd+SA treatment resulted in a value below that of the control ().
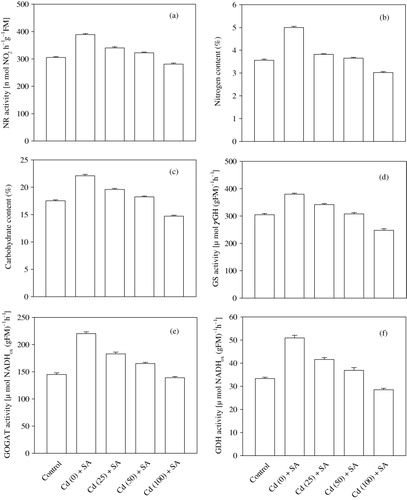
3.6. Leaf nitrogen content
The leaf nitrogen content increased by 40.4%, 7.3%, and 2.5% in response to SA applied to the unstressed and Cd-stressed (25 and 50 mg per kg of soil) plants, respectively, over the control (). However, the nitrogen content in the plants exposed to 100 mg Cd and SA was 15.8% lower than that of the control ().
3.7. Carbohydrate content in nodules
The carbohydrate content in the nodules increased significantly by 26.2% (unstressed plants) and 11.9% (25 mg Cd-applied plants sprayed with SA). However, the nodule carbohydrate content of the plants exposed to 50 mg Cd per kg of soil together with SA was statistically equal to that of the control. Like other parameters, the carbohydrate content of plants exposed to 100 mg Cd per kg of soil was not improved by the application of SA; in these plants, a reduction of 16.0% compared with the control was observed ().
3.8. GS activity in nodules
The application of 10−5 M SA to the foliage of chickpea plants resulted in significant increase of 24.6% (unstressed plants) and 12.1% (25 mg Cd) in the GS activity compared with the control (). The GS activity in the nodules of the plants treated with Cd at 50 mg per kg of soil and sprayed with SA was statistically equal to that of the control. In contrast, the plants grown in 100 mg Cd per kg of soil and sprayed with SA had 18.7% lower GS activity than the control.
3.9. GOGAT and GDH activities in nodules
The foliar application of SA significantly enhanced the activity of GOGAT by 51.7% (unstressed plants), 26.2% (25 mg Cd), and 13.8% (50 mg Cd) relative to the control, whereas the GOGAT activity in the nodules of the plants grown in soil supplemented with 100 mg Cd per kg of soil was found to be statistically equal to that of the control (). The GDH activity followed a pattern similar to that of the GOGAT activity ().
4. Discussion
The toxic effects of heavy metals, particularly cadmium, on crop plants have received considerable attention in recent years. It has been observed that exposure to heavy metals can cause perturbations in various plant processes, such as nitrogen fixation and nitrogen assimilation. Cadmium is known to decrease nodulation (Rana & Ahmad Citation2002) by affecting the establishment of the symbiosis between the host and Rhizobium species, thereby decreasing the number and the fresh and dry masses of nodules and reducing the activity of nitrogenase. These effects consequently reduce the total nitrogen content. Cd stress is also known to induce nodule senescence (Balestrasse et al. Citation2004), resulting in a decrease in the leghemoglobin content; leghemoglobin protects the O2-labile enzyme nitrogenase, so a lower leghemoglobin content decreases the nitrogenase activity as well. The breakdown of leghemoglobin might be a consequence of the increased generation of ROS, which is a characteristic feature of Cd toxicity (Balestrasse et al. Citation2004).
The sequence of events that leads to the establishment of mature nitrogen-fixing nodules begins with the infection thread formed by the joint action of the bacteria and host, which is somehow activated by phytohormones (Dart Citation1977; Hopkins Citation1995). This conclusion is also supported by the observed increase in nodulation and nodule mass in Cajanus cajan and Vigna sinensis (Singh & Kumar Citation1989) supplemented with auxins. Similarly, pea (Nandwal & Bharti Citation1982) and groundnut (Bishnoi & Krishna Citation1970) plants exhibited a higher nitrogen-fixing ability due to cytokinin. Brassinosteroids, another class of phytohormones, also affected the nodulation and nitrogen fixation of groundnut (Vardhini & Rao Citation1999) and chickpea plants (Ali et al. Citation2007). These results suggest that chemicals belonging to various groups of phytohormones have an inherent ability to activate the symbiotic association between the host and bacteria by favoring increase in the number of nodules and in the nodule mass. Similarly, the exogenous application of SA favors legume-Rhizobium symbiosis (Hayat et al. Citation2010), thereby leading to the enhanced establishment and development of nodules, which is reflected by the increase in the nodule number and the fresh and dry masses (). Phenolic compounds are known to possess chelating properties and prevent the degradation of auxin (Schneider & Wightman Citation1974), and auxins are known to increase the content of leghemoglobin (Hayat et al. Citation2009), in agreement with the observed increase in the nodule leghemoglobin content observed in the present study (). The nitrogen-fixing potential of each nodule is determined by three main factors (Cooper & Scherer Citation2012): (1) the availability of photosynthates; (2) a low oxygen supply to the bacteroids (an excessive level inhibits nitrogenase), which is mediated by the increase in leghemoglobin (); and (3) the export of fixed nitrogen in the form of ammonia. The nitrogen fixed in the form of ammonia diffuses across the peribacteroid membrane to the host cytosol by simple diffusion (Udvardi & Day Citation1990) and is further metabolized by GDH, GS, and GOGAT. GDH catalyzes the direct reductive amination of α-ketoglutarate to produce glutamate, whereas GS catalyzes the addition of to glutamate, forming the corresponding amide, glutamine. Glutamine is then converted into glutamate by the transfer of the amide group to a molecule of α-ketoglutarate by the action of GOGAT (Hopkins Citation1995). In our study, the exogenous application of 10−5 M SA to the foliage of the Cd-stressed plants resulted in enhanced GS, GOGAT, and GDH activities (), which may be due to the ROS-scavenging potential of SA via the enhancement of the activity of antioxidative enzymes, i.e. Catalase (CAT), peroxidase (POX), and superoxide dismutase (SOD) (Hayat et al. Citation2010Hayat et al. Citation2012). These results are supported by previous findings in which exogenous SA (Hayat et al. Citation2008) was shown to increase the activity of antioxidative enzymes (CAT, POX, and SOD) by acting at the level of transcription and/or translation, thereby preventing oxidative damage to plants in general and nodules in particular. In our study, the number of nodules, the fresh and dry nodule masses, and the leghemoglobin content () were increased in the Cd-stressed plants sprayed with SA. The development of healthy nodules, as expressed in terms of an increase in the number of nodules and their fresh and dry masses (), coupled with an increased leghemoglobin content (), is expected to increase the nodule nitrogenase activity, which is evident from the data shown in .
The foliage of the plants exposed to Cd stress exhibited a decline in the activity of NR, which may be due to the inhibition and/or metabolic dysfunction of this enzyme (Hopkins Citation1995). Moreover, the metal also has a negative impact on the activity of plasma membrane-bound ATP proton pumps (Obata et al. Citation1996) and the fluidity of membranes (Meharg Citation1993), thereby restricting the uptake of nitrate, an inducer of NR (Hernandez et al. Citation1996; Campbell Citation1999), and decreasing the nitrate content in the roots and, consequently, the activity of NR in the whole plant. In the present investigation, the exogenous application of 10−5 M SA to the Cd-stressed plants resulted in a significant increase in the activity of NR in the leaves () and in the nitrate content in the roots (). The increased activity of NR could have either been a result of an interaction between SA- and NR-specific inhibitors, as claimed by Srivastava (Citation1980), and/or through the induction of other phytohormones, such as auxins. Auxins (IAA and Cl-IAA) are protected by phenols (Schneider & Wightman Citation1974) and elevate the activity of NR, as has been observed in pea (Ahmad & Hayat Citation1999), mustard (Ahmad et al. Citation2001), and chickpea plants (Hayat et al. Citation2009). Our results suggest that the most appropriate explanation for the SA-mediated elevation of the activity of NR is that SA stabilizes the membrane structure and fluidity, which could have facilitated the increased uptake of nutrients, including nitrate, thus increasing the root concentration of nitrate (), which also acts as an inducer of NR (Campbell Citation1999). The increase in the content of nitrate and in the activity of NR due to SA treatment is well established (Hayat et al. Citation2005), strongly supporting our results. The increased activity of NR might also be attributable to the fact that, at lower concentrations (10−5 M), SA acts as an enzyme protectant under stress conditions (Rane et al. Citation1995; Hayat et al. Citation2010). The exogenous application of SA also increases the net photosynthetic rate by increasing the stomatal conductance and the activity of carbonic anhydrase, favoring the production of photosynthates (Hayat et al. Citation2010, Citation2012). The nodules, therefore, obtain a significant proportion of the carbohydrates () for the rapidly metabolizing bacteria, as previously reported by Hayat et al. (Citation2009). The enhanced efficiency of nitrogen fixation and nitrogen assimilation culminated in increased nitrogen content ().
5. Conclusion
It can be concluded that SA treatment applied as a foliar spray enhanced the efficiency of nitrogen fixation and assimilation by increasing the nodulation and the activities of various enzymes such as nitrogenase, NR, GS, GOGAT, and GDH even under the cadmium stress.
Acknowledgements
The authors extend their appreciation to the Deanship of Scientific Research at King Saud University for funding the work through the research group project No. RGP-VPP-199.
References
- Ahmad, A, & Hayat, S (1999). Response of nitrate reductase to substituted indoleacetic acids in pea seedlings. GC Srivastava (Ed.), Plant physiology for sustainable agriculture, (pp. 252–9). Meerut: Kalyani Publisher.
- Ahmad, A, Hayat, S, Fariduddin, Q, & Ahmad, I (2001). Photosynthetic efficiency of plants of Brassica juncea, treated with chlorosubstituted auxins. Photosynthetica., 39, 365–8. doi: 10.1023/A:1015608229741
- Ali, B, Rani, I, Hayat, S, & Ahmad, A (2007). Effect of 4-Cl-indole-3-acetic acid on the seed germination of Cicer arietinum exposed to cadmium. Acta Bot. Croat., 66, 57–65.
- Ananieva, EA, Christov, KN, & Popova, LP (2004). Exogenous treatment with salicylic acid leads to increased antioxidant capacity in leaves of barley plants exposed to paraquat. J. Plant Physiol., 161, 319–28. doi: 10.1078/0176-1617-01022
- Balestrasse, KB, Gallego, SM, & Tomaro, ML (2004). Cadmium-induced senescence in nodules of soybean (Glycine max L.) plants. Plant Soil., 262, 373–81. doi: 10.1023/B:PLSO.0000037056.11877.7b
- Barcelo, J, & Poschenrieder, C (1990). Plant water relations as affected by heavy metal stress: a review. J. Plant Nut., 13, 1–37. doi: 10.1080/01904169009364057
- Bishnoi, NR, & Krishna, MHN (1970). Effect of waterlogging and gibberellic acid on nodulation and nitrogen fixation in peanut. Plant Physiol. Biochem., 28, 663–6.
- Campbell, HW (1999). Nitrate reductase structure, function and regulation bridging the gap between biochemistry and physiology. Annu. Rev. Plant Physiol. Plant Mol. Biol., 50, 277–303. doi: 10.1146/annurev.arplant.50.1.277
- Cooper, J, & Scherer, H (2012). Nitrogen fixation. P Marchner (Ed.), (3rd ed). Mineral nutrition of higher plants, (pp. 389–408). London: Academic Press.
- Dart, PJ (1977). Infection and development of leguminous nodules. RWI Hardy, & WS Silver (Eds.), A treatise of dinitrogen fixation section-III. Biology, (pp. 367–472). New York, NY: Wiley.
- De Vos, CHR, & Schat, H (1981). Free radicals and heavy metal tolerance. J Rozema, & J Verkleij (Eds.), Ecological response to environmental stress, (pp. 22–30). , The Netherlands: Kluwer Academic Publishers.
- Dubois, M, Gills, K, Hamilton, JK, Robers, PA, & Smith, F (1956). Colorimetric method for determination of sugars and related substances. Annal. Chem., 28, 350–6. doi: 10.1021/ac60111a017
- Ekmekci, Y, Tanyolac, D, & Ayhan, B (2008). Effect of cadmium on antioxidant enzyme and photosynthetic activities in leaves of two maize cultivars. J. Plant Physiol., 165, 600–11. doi: 10.1016/j.jplph.2007.01.017
- Habibi, G (2012). Exogenous salicylic acid alleviates oxidative damage of barley plants under drought stress. Acta Biol. Szeged., 56(1), 57–63.
- Hardy, RWE, Holstein, RW, Jackson, EK, & Burns, RC (1968). The acetylene ethylene assay for nitrogen fixation. Lab. Field Evaluation. Plant Physiol., 43, 1185–207. doi: 10.1104/pp.43.8.1185
- Hasan, SA, Hayat, S, Ali, B, & Ahmad, A (2008). 28-Homobrassinolide protects chickpea (Cicer arietinum) from cadmium toxicity by stimulating antioxidants. Environ. Poll., 151, 60–6. doi: 10.1016/j.envpol.2007.03.006
- Hayat Q. 2010. Effect of proline and salicylic acid on the cadmium induced changes in chickpea (Cicer arietinum L.) [Ph.D thesis]. Aligarh: Aligarh Muslim University.
- Hayat, S, Fariduddin, Q, Ali, B, & Ahmad, A (2005). Effect of salicylic acid on growth and enzyme activities of wheat seedlings. Acta Agron. Hung., 53, 433–7. doi: 10.1556/AAgr.53.2005.4.9
- Hayat, S, Hasan, SA, Fariduddin, Q, & Ahmad, A (2008). Growth of tomato (Lycopersicon esculentum) in response to salicylic acid under water stress. J. Plant Inter., 3, 297–304. doi: 10.1080/17429140802320797
- Hayat, Q, Hayat, S, Ali, B, & Ahmad, A (2009). Auxin analogues and nitrogen metabolism, photosynthesis, and yield of chickpea. J. Plant Nutr., 32, 1469–85. doi: 10.1080/01904160903092671
- Hayat, Q, Hayat, S, Alyemeni, MN, & Ahmad, A (2012). Salicylic acid mediated changes in growth, photosynthesis, nitrogen metabolism and antioxidant defense system in Cicer arietinum L. Plant Soil Environ., 58(9), 417–23.
- Hayat, Q, Hayat, S, Irfan, M, & Ahmad, A (2010). Effect of exogenous salicylic acid under changing environment: a review. Environ. Exp. Bot., 68, 14–25. doi: 10.1016/j.envexpbot.2009.08.005
- Hernandez, LL, Carpena-Ruiz, R, & Garate, A (1996). Alterations in the mineral nutrition of pea seedlings exposed to cadmium. J. Plant Nutr., 19, 1581–6. doi: 10.1080/01904169609365223
- Hopkins WJ. 1995. Introduction to plant physiology . 2nd ed New York, NY: Wiley; 99–121, 313–25.
- Hussain, K, Nawaz, K, Majeed, A, Ilyas, U, Lin, F, Ali, K, Nisar, MF (2011). Role of salicylic acid applications for salt tolerance in violet. Sarhad J. Agric., 27(2), 171–5.
- Jarvis, C, Jones, LHP, & Hopper, MJ (1976). Cadmium uptake from solution by plants and its transport from roots to shoots. Plant Soil, 44, 179–91. doi: 10.1007/BF00016965
- Jaworski, EG (1971). Nitrate reductase assay in intact plant tissues. Biochem. Biophys. Res. Commun., 43, 1274–9. doi: 10.1016/S0006-291X(71)80010-4
- Kabata-Pendias, A, & Pendias, H (2001). (3rd ed). Trace elements in soils and plants, Boca Raton, FL: CRC Press.
- Krantev, A, Yordanova, R, Janda, T, Szalas, G, & Papova, L (2008). Treatment with salicylic acid decreases the effect of cadmium on photosynthesis in maize plants. J. Plant Physiol., 165, 920–31. doi: 10.1016/j.jplph.2006.11.014
- Lindner, RC (1944). Rapid analytical method for some of the more common inorganic constituents of plant tissues. Plant Physiol., 19, 76–89. doi: 10.1104/pp.19.1.76
- Meharg, AA (1993). Integrated tolerance mechanisms constitutive and adaptive plant response to elevated metal concentrations in the environment. Plant Cell Environ., 17, 989–93. doi: 10.1111/j.1365-3040.1994.tb02032.x
- Nandwal, AS, & Bharti, S (1982). The effect of kinetin and IAA on growth, yield and nitrogen fixing efficiency of nodules in pea. Ind. J. Plant Physiol., 25, 358–63.
- Obata, H, Inone, N, & Umebayshi, M (1996). Effect of cadmium on plasma membrane ATPase from plant root differing in tolerance to cadmium. Soil Sci. Plant Nutr., 42, 361–6. doi: 10.1080/00380768.1996.10414698
- Passariello, B, Giuliano, V, Quaresima, S, Barbaro, M, Caroli, S, Forte, G, Garelli, G, Iavicoli, I (2002). Evaluation of the environmental contamination at an abandoned mining site. Microchem. J., 73, 245–50. doi: 10.1016/S0026-265X(02)00069-3
- Patel, PK, & Hemantaranjan, A (2012). Salicylic acid induced alteration in dry matter partitioning, antioxidant defense system and yield in chickpea (Cicer arietinum) under drought stress. Asian J. Crop Sci., 4(3), 86–102. doi: 10.3923/ajcs.2012.86.102
- Rana, A, & Ahmad, M (2002). Heavy metal toxicity in legume microsymbiont system. J. Plant Nutr., 25, 369–86. doi: 10.1081/PLN-100108842
- Rane, J, Lakkineni, KC, Kumar, PA, & Abrol, YP (1995). Salicylic acid protects nitrate reductase activity of wheat leaves. Plant Physiol. Biochem., 22, 119–21.
- Sadasivam S, Manickam A. 1992. Biochemical methods. New Delhi: New Age International Publishers; 187–8.
- Schneider, EA, & Wightman, F (1974). Metabolism of auxin in higher plants. Annu. Rev. Plant Physiol., 25, 487–513. doi: 10.1146/annurev.pp.25.060174.002415
- Siedlecka, A, Krupa, Z, Samuelsson, G, Oquist, G, & Gardestrom, P (1997). Primary carbon metabolism in Phaseolus vulgaris plants under Cd (II)/Fe interaction. Plant Physiol. Biochem., 35, 951–7.
- Singh, JP (1988). A rapid method for determination of nitrate in soil and plant extracts. Plant Soil., 110, 137–9. doi: 10.1007/BF02143549
- Singh, T, & Kumar, V (1989). Nodulation and plant growth as influenced by growth regulators in some legumes. Acta Bot. Ind., 17, 177–81.
- Srivastava, HS (1980). Regulation of nitrate reductase activity in higher plants. Phytochemistry., 19, 725–33. doi: 10.1016/0031-9422(80)85100-4
- Thimmaiah, SR (1999). Standard methods of biochemical analysis, New Delhi: Kalyani Publishers.
- Udvardi, MK, & Day, DA (1990). Ammonia (14C-methylamine) transport across the bacteroid and peribacteroid membranes of soybean root nodules. Plant Physiol., 94, 71–6. doi: 10.1104/pp.94.1.71
- Vardhini, BV, & Rao, SSR (1999). Effect of brassinosteroids on nodulation and nitrogenase activity in groundnut (arachis hypogaea L.). Plant Growth Regul., 28, 165–7. doi: 10.1023/A:1006227417688
- Yang, Y, Qi, M, & Mei, C (2004). Endogenous salicylic acid protects rice plants from oxidative damage caused by ageing as well as biotic and abiotic stresses. Plant J., 40, 909–19. doi: 10.1111/j.1365-313X.2004.02267.x
- Yih, RY, & Clark, HE (1965). Carbohydrate and protein content of boron deficient tomato root tips in relation to anatomy and growth. Plant Physiol., 40, 312–5. doi: 10.1104/pp.40.2.312
- Zhang, W, & Chen, W (2011). Role of salicylic acid in alleviating photochemical damage and autophagic cell death induction of cadmium stress in Arabidopsis thaliana. Photochem. Photobiol. Sci., 10, 947–55. doi: 10.1039/c0pp00305k