Abstract
To understand the molecular responses of Thai jasmine rice (Oryza sativa L. ssp. indica cv. KDML105) under drought, proteomes of KDML105 cultivars and two check cultivars, namely NSG19 (tolerant) and IR20 (sensitive) were investigated. The effect of PEG6000 and mannitol for creating osmotic stress (−0.1 MPa to − 2.1 MPa) was tested in KDML105 before applied to the three cultivars. The leaf proteomes were analyzed by GeLC-MS/MS shotgun proteomics. Among 623 proteins identified, 53 proteins showed significant difference in protein expressions, which could be hierarchically classified into three clusters of proteins up-regulated in IR20, NSG19, or in KDML105. Coronatine-insensitive 1 protein that thought to be involved in stomatal closing was prominently observed in NSG19, regarding to its physiology of rapid stomatal closing and highest stability of photosystem II. WD-40 repeat protein which involves in cell death was induced in IR20. On the other hand, H-protein promoter binding factor-2a remarkably increased in KDML105.
1. Introduction
Drought is a major constraint to the yield of rice (Oryza sativa L.). More than half of all rice production is often lost due to drought stress, leading to increased poverty and reducing world food security (Pandey et al. Citation2007). Drought stress response mechanisms in plants are a result of complex molecular and physiological mechanisms (Chaves et al. Citation2003). These molecular responses are initiated by signal transduction resulting in transcriptional and translational control of stress-responsive genes. Several stress-responsive mechanisms are activated to reestablish cellular homeostasis as well as to protect cells during water deficit stress and include regulation of macromolecules involved in cell wall metabolism, accumulation of compatible solutes, and altered transportation of water and micromolecules (Wang et al. Citation2003). Drought stress enhances accumulation of reactive oxygen species, activates programmed cell death, and induces male sterility in rice anthers (Nguyen et al. Citation2010). Photosynthesis capacity is directly related to growth and rice yields and is one of the major systems affected by drought. Under drought stress, levels of abscisic acid are increased, resulting in closing of stomata, which is accompanied by reduced concentrations of CO2 in photosynthetic cells. In addition, photosynthetic apparatuses, especially in photosystem II (PSII), are damaged by drought stress (Giardi et al. Citation1996) altering the electron transport chain and ultimately leading to a reduction in photosynthetic ability, growth and yield of rice (Giardi et al. Citation1996; Yamane et al. Citation2003; Pieters & Souki Citation2005).
Thai jasmine rice (Oryza sativa L. ssp. indica cv. KDML105) originated in Chonburi province of Thailand and it was named as KDML105 by Sunthorn Seehanern, an official of the ministry of agriculture in Chachoengsao province of Thailand in 1954. It is also known as Thai fragrance rice caused by 2-acetyl-1-pyrroline (http://en.wikipedia.org/wiki/Jasmine_rice). NSG19 or Nam Sagui 19, a breeding line originated in Thailand, has seedling vigor and drought-adapted traits while IR20, indica rice from Philippines, has semidwarf and drought-sensitive characters (Cabuslay et al. Citation2002). Among the exported indica rice, Thai Jasmine rice has been classified as high-end trait and it was reported as the moderate drought tolerance (Sarkarung Citation2003). Whereas NSG19 and IR20 have been used as check cultivars in the rainfed lowland rice breeding program for drought tolerance and have been previously used as drought stress tolerant and sensitive cultivars, respectively (Sarkarung Citation2003). Although many studies have investigated the effects of drought stress in rice (Cabuslay et al. Citation2002; Khan & Komatsu Citation2004), the molecular responses of these indica cultivars to water deficit have not been clearly reported. In order to improve tolerance of indica rice to drought conditions, a better understanding of the molecular mechanisms that enable adaptation to conditions of drought stress is needed.
Proteomics is a powerful and direct approach for functional gene analysis and is widely used to understand the response of plants under abiotic stresses (Hashiguchi et al. Citation2010). Several protocols for proteome analysis are available that are based on protein separation, detection, and identification methods. Two major techniques used for protein separation in proteomic analysis are two-dimensional polyacrylamide gel electrophoresis (2D-PAGE)-based proteomics and liquid chromatography (LC)-based shotgun proteomics. Currently, the 2D-PAGE-based technique has been utilized almost exclusively for rice proteomics analysis (Khan & Komatsu Citation2004; Hashiguchi et al. Citation2010). However, this technique is known to be less suitable for the analysis of very high and low molecular weight proteins, as well as hydrophobic, highly acidic, and alkaline proteins (Santoni et al. Citation2000). In order to overcome these limitations, shotgun proteomics has been developed where the total mixture of digested proteins is separated on LC columns, analyzed with tandem mass spectrometry (MS/MS) followed by mapping of the peptide sequences onto the corresponding proteins using computer algorithms (Yates Citation1998; Matros et al. Citation2011). Shotgun proteomics can identify more than 1000 peptides within one analysis and also enables the detection of proteins rarely found using 2D-PAGE-based proteomics (Washburn et al. Citation2001). Moreover, shotgun proteomics has proven to be more reproducible in terms of peptide identification and protein quantitation than other techniques (Delmotte et al. Citation2009; Piersma et al. Citation2010; Tabb et al. Citation2010). While the complexity of the peptide mixtures limits the use of shotgun proteomics (Malmstrom et al. Citation2007), this problem can be reduced through the use of gel-based liquid chromatography-tandem mass spectrometry (GeLC-MS/MS) in which proteins are first separated by sodium dodecyl sulfate-PAGE (SDS-PAGE) then the entire gel is sliced into pieces according to protein marker sizes before digestion and analysis by MS/MS (Rezaul et al. Citation2005). Thus, the GeLC-MS/MS approach can substantially reduce the number of proteins present in each sample making it suitable for quantitative proteomic studies in complex organisms.
The identification of proteins with altered abundance in response to drought stress has been reported for many crop plants (Hashiguchi et al. Citation2010). However, studies of rice proteomes under drought stress have been limited to only a few cultivars with most studies being conducted with japonica rice (Oryza sativa L. ssp. japonica) using conventional 2D-PAGE-based proteomic analysis (Salekdeh et al. Citation2002; Ali & Komatsu Citation2006; Zang and Komatsu Citation2007). Moreover, there is no convincing evidence on the suitability of osmotic agents for generating osmotic stress in rice seedlings to simulate drought conditions. Hence, the effects of polyethylene glycol (PEG) 6000- and mannitol-inducing water stress on rice seedling were investigated in KDML105 and leaf proteomes were analyzed using the GeLC-MS/MS technique in the three different indica cultivars, KDML105, NSG19, and IR20. Comparative proteomic analyses of these cultivars in response to drought stress may lead to a better understanding of the drought stress response mechanisms and could identify proteins that may suggest strategies for generating rice capable of thriving in drought-prone environments.
2. Materials and methods
2.1. Plant materials, growth conditions, and stress treatments
Seeds of rice (Oryza sativa L. ssp. indica cv. KDML105, NSG19, and IR20) were obtained from the Pathumthani Rice Research Center, Rice Research Institute, Department of Agriculture, Ministry of Agriculture and Cooperative, Pathumthani, Thailand. The accession numbers of KDML105, NSG19, and IR20 are 27748, 11462, and 11355, respectively. Seedlings were hydroponically grown in Yoshida nutrient solution (Yoshida Citation1981) under controlled conditions (25±2 °C, 60±5% relative humidity, and 80±5 µmol m−2 s−1 photosynthetic photon flux with a 16 h d−1 photoperiod). The susceptibility of KDML105 rice seedling to drought was tested by transferring the two-week-old seedlings into media solutions containing 0, 20, 30, and 40% (w/v) PEG6000 or 0-, 200-, 400-, and 800-mM mannitol, which created osmotic stress of approximately − 0.1 to − 2.1 MPa. The period of exposure to drought stress was varied between 0, 24, 48, 96, and 192 h. The three-week-old seedlings of all cultivars were grown under PEG-created water stress at approximately − 0.5 MPa for 48 and 96 h. The osmolality of the media was measured using the VAPRO®® Vapor Pressure Deficit Osmometer (WESCOR Inc., USA) and osmotic pressure of the media was calculated following the method of Money (Citation1989). Leaf samples were harvested for GeLC-MS/MS shotgun analysis.
2.2. Morphological and physiological measurements
Seedling fresh weight (FW), dry weight (DW), shoot length, and the maximum quantum efficiency of PSII photochemistry (Fv/Fm) were measured from 20 seedlings in each treatment group. The Fv/Fm was measured using a portable fluorometer Handy PEA (Hansatech Instruments Ltd., King's Lynn, UK) following the manufacturer's instructions. Eight seedlings were randomly sampled to calculate the shoot:root ratio (division of shoot DW to root DW) and percentage of relative water content (RWC) calculated using the equation: RWC=([FW − DW]/FW)×100. Concentrations of chlorophyll a (chl a) and chlorophyll b (chl b) were analyzed as described by Cha-um et al. (Citation2006) with the modifications from Lichtenthaler (Citation1987) and Shabala et al. (Citation1998). To evaluate the rate of stomata closing, the three-leaf stage seedlings from tissue culture were used to evaluate guard cells under water stress of approximately − 0.5 MPa for 0, 1, 3, 5, and 10 min. Guard cells were sampled from the fully expanded leaf of two seedlings (two regions per seedling) and evaluated within an area of 66.57 mm2 by observation with 40× microscopic magnification. The percentage of stomatal closing calculated using the equation: stomatal closing (%)=(numbers of close stomata/numbers of total stomata)×100. Statistical analysis of all data was conducted using the SPSS software (SPSS for Windows; SPSS Inc., Chicago, IL) and mean values were compared by employing Duncan's multiple range test with a P value ≤ 0.01 defined as statistically significant. The bars in all figures represent the standard deviation of the mean.
2.3. GeLC-MS/MS shotgun proteomics
Leaf samples were taken from the pooled samples of leaves from 20 independent rice seedlings grown under PEG-created water stress at − 0.5 MPa for 0, 48, and 96 h. Total proteins were extracted from 200 mg of leaf samples after being ground in liquid nitrogen and precipitated with trichloroacetic acid/acetone according to Granier (Citation1988) with slight modifications. The protein concentrations were determined as described by Lowry et al. (Citation1951). Fifteen nanogram of the protein from each sample was pre-fractionated by 12.5% SDS-PAGE followed by silver staining (Blum et al. Citation1987). The size of polypeptides was estimated by comparison with a low molecular weight protein standard marker (Amersham Biosciences, Buckinghamshire, UK). Gels were cut into five pieces according to their sizes (less than 20, 20–30, 30–45, 45–66, and over 66 kDa). The 45 gel pieces from nine treatments were obtained and each gel piece was cut into small pieces (about 1×1 mm2) before aliquot into a 96-well plate for in-gel digestion. In brief, the gel plugs were dehydrated with 100% acetonitrile (ACN), reduced with 10-mM dithiothreitol in 10-mM ammonium bicarbonate, and alkylated (in the dark) with 100-mM iodoacetamide in 10-mM ammonium bicarbonate, respectively. Each step was performed at room temperature for 1 h and gel pieces were dehydrated twice with 100% ACN for 5 min before digestion with 10 µL of trypsin solution (10-ng/µL trypsin in 50% ACN/10-mM ammonium bicarbonate) at room temperature for 20 min. Gels were immersed in 20 µL of 30% ACN and incubated at 37 ° C overnight, and digested peptide products were extracted from gel pieces by adding 30 µL of 50% ACN in 0.1% formic acid and shaking at room temperature for 10 min. Extracted peptides that came from the same treatment and the same size interval on the 96-well plate were pooled together in a new tube. Finally, the 45 peptide samples (nine treatments×five intervals) were prepared for further mass spectrometric analysis and the analysis was performed in triplicate. Peptide samples were injected into an Ultimate 3000 LC System (Dionex, Sunnyvale, CA) coupled to an ESI-Ion Trap MS, HCT Ultra PTM Discovery System (Bruker, Rheinstetten, Germany) with electrospray at a flow rate of 300 nL/min to a nanocolumn (Acclaim PepMap 100 C18, 3 µm, 100Å, 75 µm id×150 mm). The solvent gradient utilized in this analysis was solvent A (0.1% formic acid in water) and 80% of solvent B (0.1% formic acid in 80% ACN) with the grading running in 40 min.
2.4. Protein quantitation and identification
Proteins were quantified by using the DeCyder MS 2.0 Differential Analysis software (GE Healthcare Europe GmbH, Freiburg, Germany) (Johansson et al. Citation2006; Thorsell et al. Citation2007). The PepDetect module was used for peptide detection, charge state assignments, and quantitation. Protein identifications were assigned using the Mascot search engine (Matrix Science, London, UK) (Perkins et al. Citation1999). Only significant hits, as defined by the MASCOT algorithm at P ≤ 0.05, were accepted. The subcellular and functional annotation of the identified proteins was screened and assigned according to the biological function using the Gene Ontology Categorizer (http://eagl.unige.ch/GOCat). The protein expression patterns from each cultivar was statistically compared among the treatments by one-way ANOVA algorithm using the Multi Experiment Viewer (MEV) software version 4.6.2 (The Institute for Genomic Research) with P ≤0.05 and 0.10. Proteins which displayed significant differences in their expression patterns were further analyzed using hierarchical and K-mean clustering and their biological functions were defined using the InterPro database (http://www.ebi.ac.uk/interpro).
3. Results
3.1. Effect of PEG6000- and mannitol-inducing drought stress on rice seedlings
To compare the effect of PEG6000 and mannitol on rice seedlings, 2-week-old seedlings of KDML105 were transferred into media containing PEG6000 or mannitol, both of which mimic the effect of drought stress. The reduction of shoot or root FW, shoot length, and Fv/Fm were more readily observed upon treatment with PEG6000 compared with mannitol (). These changes could be observed at an osmotic stress of approximately − 0.4 to − 0.6 MPa with more severe effects observed with an increase in stress. At an osmotic stress of approximately − 0.9 to − 1.1 MPa, shoot FW, shoot length, and Fv/Fm were decreased by 57.6, 14.9, and 93.0%, respectively, with PEG6000 treatment, whereas these parameters were decreased by 42.7, 1.1, and 55.1% with mannitol treatment.
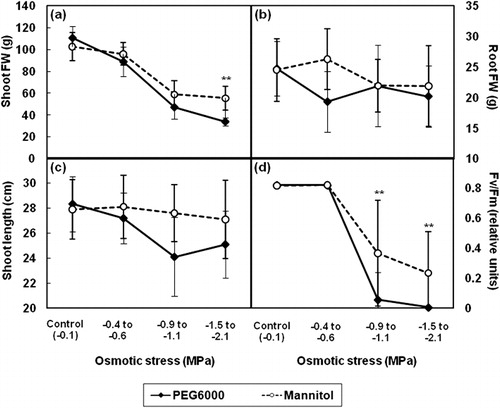
3.2. Effect of PEG6000-induced drought stress on growth, RWC, photosynthesis, and stomatal closing
PEG6000-induced drought stress (−0.5 MPa) significantly affected the growth, RWC, and photosynthetic ability of rice seedlings (). The RWC and shoot:root ratio were dramatically reduced after 48 and 96 h of stress. After 96 h of drought stress, the RWC of seedlings was reduced by 7.3% and the shoot:root ratio was decreased by 25.0%. Moreover, the levels of chl a and chl b were decreased by 10.6 and 11.5%, respectively. To determine the best osmotic stress agent, the effects of mannitol and PEG6000 were evaluated with KDML105 rice. Once it was established that PEG6000 was more effective for inducing drought stress in KDML105 seedlings, its effect on Fv/Fm and stomatal closing was evaluated in KDML105, NSG19, and IR20. The Fv/Fm was observed at 48 and 96 h after treatment with PEG6000 () and after 96 h of stress, the reduction in Fv/Fm was larger in IR20 (9.4%) than in KDML105 (4.2%) with no significant change was observed in NSG19. In addition, NSG19 closed their stomata faster than KDML105 and IR20 (). After 1 min of water deficit, 80% of stomata in NSG19 was closed, whereas only about 20% in KDML105 and IR20 was closed, even though eventually 100% of the stomata was closed after 3, 5, and 10 min, respectively.
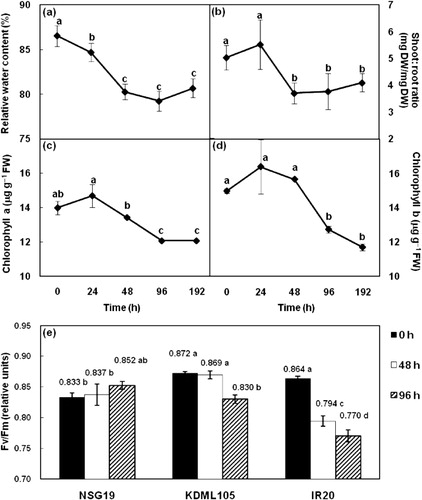
3.3. Comparative proteomic analyses of three indica rice cultivars under drought stress
In the present study, 623 proteins, with significantly differing expression patterns under drought stress were detected using GeLC–MS/MS shotgun proteomics and identified using the Mascot software (Perkins et al. Citation1999). Among these proteins, 369 could be assigned to known proteins, while the others were hypothetical, unknown, or unnamed proteins. The expression patterns of all 623 proteins were compared among the three indica cultivars, IR20, KDML105, and NSG19, by using the MEV software. Fifty-three proteins showed significantly different protein expression patterns among the three cultivars (P ≤ 0.10), whereas 44 proteins showed similar expression patterns (P ≤ 0.05). Other proteins do not meet the statistical criteria analyzed by MEV software. The 53 differentially expressed proteins were further analyzed using hierarchical clustering () and K-mean clustering (). Hierarchical clustering showed expression patterns of each protein and K-mean sorted these proteins into three groups: Group I, up-regulated in IR20; Group II, up-regulated in KDML105; and Group III, up-regulated in NSG19. The biological functions of the proteins present in each group were defined using the InterPro database (http://www.ebi.ac.uk/interpro) (). Proteins that exhibited a significant difference in expression patterns under water stress (>1.5-fold change) among the rice cultivars are given in .
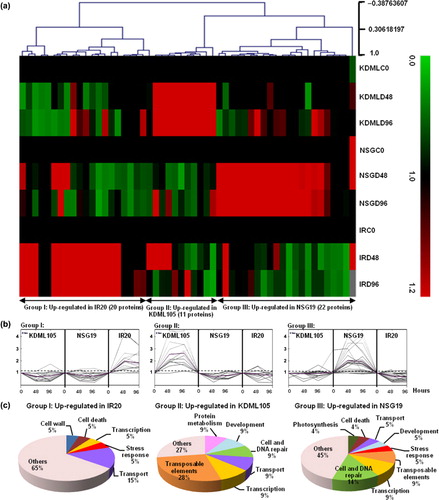
Table 1. Proteins that exhibited different expression patterns (P ≤ 0.10) among rice cultivars and was inducted more than 1.5 folds under water stress.
4. Discussion
4.1. PEG6000 is a better osmoticum for water stress studies in rice seedlings compared to mannitol
Many studies on drought stress responses in rice have been conducted by transferring rice seedlings into a nutrient solution containing osmotic agents such as mannitol (Zang & Komatsu Citation2007) or PEG6000 (Xiong et al. Citation2010) to create osmotic stress. However, examination as to which agent is more suitable for induction of water stress in rice seedlings has rarely been reported. In the present study, PEG6000 affected the growth and photosynthetic ability of rice seedlings and resulted in a remarkable reduction in shoot FW, shoot length, and Fv/Fm. The effect of PEG6000 on these parameters was greater than upon treatment with mannitol, and these effects became more severe as the stress increased (). There are some comparative studies on the effect of PEG and mannitol as osmotic agents on plants (Hohl & Schopfer Citation1991; Pandey et al. Citation2004; Slama et al. Citation2007). PEG6000 affects on changes of stress-related enzymes and compounds in 5-day-old rice seedlings more than mannitol (Pandey et al. Citation2004). In contrast to PEG6000, mannitol can be transported into root cells lowering its ability to produce water stress in plants (Hohl & Schopfer Citation1991). Thus, in order to avoid the adaptation of rice seedlings under conditions of mild stress, PEG6000 is preferred for use as an osmoticum.
4.2. Drought affects chlorophyll and photooxidative damage of PSII in rice seedlings
Drought stresses have been reported to damage the photosynthetic system by causing severe disruption of the chloroplast envelope (Yamane et al. Citation2003; Vassileva et al. Citation2012) and the PSII complex. A consequence of this damage is a reduction in chlorophyll content, chl a fluorescence parameters, and D1 protein levels (Giardi et al. Citation1996; Pieters & Souki Citation2005). In this study, we observed that the contents of chl a and chl b were progressively reduced after 24 h of water stress ( and ). This suggests that the degradation of chlorophylls is occurring under drought stress. Remarkably, the contents of chl b were slightly higher than the contents of chl a. This might due to the low light intensity during the growing and experimental condition. Chlorophyll fluorescence is widely used as a tool to assess the response of plants to environmental stress (Sayed Citation2003) and the decrease in Fv/Fm, one of the chlorophyll fluorescence parameters, refers to increases in photo-inactivation and oxidative damage of PSII reaction centers (Baker Citation2008). Our results indicated that drought stress may indirectly affect PSII activity of rice seedlings as shown by a reduction of Fv/Fm ( and ). The reduction in Fv/Fm under drought stress has been observed in many plant species such as rice (Garg et al. Citation2002), barley (Li et al. Citation2006), and maize (Efeoglu et al. Citation2009). The smaller changes in the chlorophyll fluorescence yield can indicate the ability to tolerate water stress. The data in indicate that high photooxidative damage to the PSII reaction center occurred in the IR20 and that this cultivar was more susceptible to such damage than the KDML105 and NSG19 cultivars. Consistent with our results, Cha-um et al. (Citation2010) observed that Fv/Fm ratios were significantly reduced after water deficit (7% soil water content) in IR20, KDML105, and NSG19 by 25.9, 6.5, and 2.1%, respectively. In addition, a reduction in chl a, chl b, RWC, and yield traits were more readily observed in IR20 compared to NSG19 and KDML105 (Cha-um et al. Citation2010). Taken together, these observations suggest that under drought stress reduced levels of water in rice leaves may increase oxidative stress leading to damage of chlorophyll pigments and PSII ultimately resulting in reduced photosynthetic efficiency. Finally, these changes are likely to affect overall photosynthesis and yield, especially in the IR20 cultivar.
4.3. GeLC-MS/MS shotgun proteomics as a powerful tool for analysis of leaf proteins in rice
Proteomics has been proposed to be an effective tool for studying plant stress responses (Hashiguchi et al. Citation2010; Kim et al. Citation2011). With the increased interest in analyzing plant proteomes, shotgun proteomics has been developed for rapid, highly accurate, and effective detection of protein expression under specific conditions (Yates Citation1998; Delmotte et al. Citation2009; Piersma et al. Citation2010; Tabb et al. Citation2010; Matros et al. Citation2011). However, shotgun proteomics is limited by the complexity of the peptide mixtures (Malmstrom et al. Citation2007). From our preliminary data, the subcellular and functional categorization of the 369 known proteins was performed based on their biological function using the Gene Ontology Categorizer (http://eagl.unige.ch/GOCat). These results indicate that the response to water stress mainly altered proteins localized in the nucleus (58%), cytoplasm (13%), and membranes (12%). However, proteins localized to organelles that play important roles in energy and metabolism, i.e. mitochondria (7%), chloroplast (3%), endoplasmic reticulum (ER), and Golgi apparatus (3%), were also affected. Proteins whose functions correspond to stress response (17%) and metabolism (17%) were detected as well as those involved in transcription (11%), transport (9%), signaling (7%), and cell death (7%). The wide variety of proteins identified indicates the complexity of leaf proteins and the complicated network of drought stress response in rice leaves. GeLC-MS/MS is a recent technique for shotgun proteomics and is designed to overcome problems caused by sample complexity (Rezaul et al. Citation2005; Gilmore and Washburn Citation2010). Since shotgun proteomics produces very large data-sets due to the extreme redundancy in shotgun MS/MS data-sets, it may be difficult to interpret the results (Malmstrom et al. Citation2007). In this study, the complex protein mixtures were pre-fractionated by SDS-PAGE then physically separated by cutting each lane in the gel into five pieces prior to digestion and analysis by MS, resulting in reduced protein complexity in each analysis. Several thousand peptide sequences were detected from each gel piece using the DeCyder MS 2.0 Differential Analysis Software and the redundant data were filtered out and only the MS/MS data showing significant differences among treatments (P ≤ 0.05) were exported to the Mascot search engine. The 623 nonredundant proteins were then obtained from the Mascot search at P ≤ 0.05 and the patterns of protein expression were statistically compared and clustered by using the MEV software, as shown in and . Analysis with the MEV software package has the potential to increase the reliability of the results and reveal new insights from large data-sets. Thus, even with the high level of complexity, proteome analysis of rice samples can be studied effectively by the GeLC-MS/MS technique, together with the appropriate computer algorithms and databases.
4.4. Protein profiling reveals different drought stress responses of the rice cultivars
Different rice cultivars may have different response mechanisms to drought and in this study we observed that each indica rice cultivar altered expression of a different set of proteins in response to drought stress (). According to the results from hierarchical and K-mean clustering, protein expression can be classified into three groups according to their specific high accumulation in each cultivar (). Possible functions of the proteins in each group were reviewed and discussed in order to gain an insight for adaptation to water stress in these three indica rice.
4.4.1. Proteins highly up-regulated in IR20
Cell death is one of the commonly defensive mechanisms of plant under abiotic stresses. The WD-40 repeat proteins (WD40) were increased about 2.5 times under water stress in IR20 (). These data are in accordance with those found in leaves and roots of maize seedlings after treatment with 20% PEG for 6 h (3.77- and 1.44-fold increase, respectively; Zheng et al. Citation2004). In addition, BnSWD1, a WD40 repeat-containing protein, is involved in salt stress in Brassica napus (Lee et al., Citation2010). The WD-40 repeats are short ~40 amino acid motifs and are usually found in E3 ubiquitin ligase enzymes which functions in cell cycle control and apoptosis (Yee & Goring Citation2009). Thus, IR20 may have a higher rate of cell death due to sensitivity to drought stress compared to KDML105 and NSG19. According to Liu et al. Citation2007, root cells of IR20 display and increased rate of cell death in response to drought stress (15% PEG treatment for 2, 4, and 8 days) than those found in the drought-adapted line, IRAT109 (O. sativa ssp. japonica). Moreover, it is in fact that PSII cannot function in the death cells thus the increase expression of WD40 might indirectly involve with a reduction of Fv/Fm in IR20. It was found that Os02g0511600 protein showed 2.21–2.62 times induction in IR20 under water stress (). This protein was annotated by the Rice Annotation Project (http://rapdb.lab.nig.ac.jp/) as a glycosyltransferase (GT) family 31 protein (GT31) and its function is protein glycosylation (GO:0006486). GTs were found to be induced by drought stress in Macrotyloma uniflorum (Lam.) Verdc. (Reddy et al. Citation2008). Glycosylation by GTs has a key function in the modification of plant secondary metabolites and maintenance of cell and hormone homeostasis (Wang & Hou Citation2009). The GT31 enzyme was found to be widely distributed in several plants but was most abundant in rice (Cao et al. Citation2008) suggesting a vital role of GT31 in rice. The function of GT31 in rice is unclear but it has been hypothesized to be involved in cell wall biosynthesis (Cao et al. Citation2008; Bosch et al. Citation2011). However, this protein consists of short amino acid sequence (132 amino acids) so it would not be involved in modifications related to the cell wall. In addition, 15% of the up-regulated proteins were thought to be involved in transportation (). For example, a putative potassium transporter was up-regulated 2.75 times in IR20 (). This may implicate the activity of K+ uptake to balance the cellular electro-neutrality under water stress (Banuelos et al. Citation2002). Therefore, drought stress appears to mainly induces changes in expressions of proteins involve in cell death, protein glycosylation, and transportation.
4.4.2. Proteins highly up-regulated in KDML105
H-protein promoter binding factor-2a (Hp2a) was found to be increased about 3.5-fold in KDML105 (). This protein is homologous to the Dof zinc finger protein, a plant-specific transcription factor, which functions as a transcriptional activator or repressor and is involved in diverse plant-specific biological processes (Yanagisawa Citation2004). Rdd1 is a homolog of Hp2a in rice. It has been reported to be involved in phytochrome A signaling (Tepperman et al. Citation2001), growth, grain size, and flowering time in rice (Iwamoto et al. Citation2009). Another protein of interest is the DEAD/DEAH box RNA helicase protein (). This protein plays a role in DNA repair and was identified as a new candidate for engineering plant stress tolerance (Vashisht & Tuteja Citation2006). Moreover, it is of note that 28% of the up-regulated proteins in KDML105 were assigned as transposable elements (TEs) e.g. transposon or retrotransposon-encoded proteins ( and ). Of these, retrotransposon protein (gi|108862435) was increased about 4.06–4.57 times after drought stress treatment in KDML105 while slightly changed in NSG19 and IR20. TEs contribute to the organization and evolution of plant genome (Sabot et al. Citation2004). It may assist in adaptation to extreme environment by insertion causing null alleles, genome restructuring, sequence reorganization, coding sequences generation, increase in genome size, chromosomal breaks and translocations, splicing, and intron creation (Sabot et al. Citation2004). The finding that much higher percentage of TE-related proteins was found in KDML105 than NSG19 and hardly any found in IR20 might implicate to the ability of cellular adaptation to drought of these cultivars. KDML105 and NSG19 should have better adaptation to drought than IR20. However, at the cellular level, NSG19 may experience less water stress due to longer root structure (data not shown) and rapid stomatal closing () and these may consequently result to less stimuli for enhancing the expression of TE-related proteins in NSG19. TEs also play important role in rice epigenetic regulation (Zhao & Zhou Citation2012). Generally, DNA methylation is maintained for repressing the rice TE expression but the expression can be triggered by the environmental stresses coincide with the loss of DNA methylation (Zhao & Zhou Citation2012). Therefore, the major responses in KDML105 might be involved with phytochrome A signaling, DNA repair and alteration of the epigenetic regulation resulting in higher water stress tolerance.
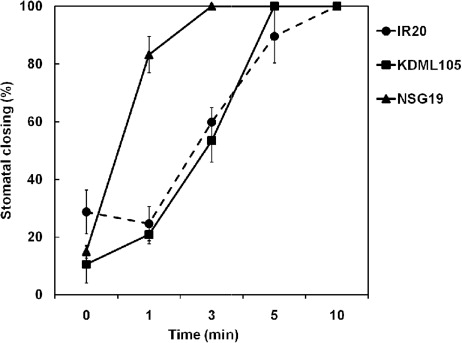
4.4.3. Proteins highly up-regulated in NSG19
Our results showed that 14% of proteins highly expressed in NSG19 are involved in cell and DNA repair (). Among those proteins, the tetratricopeptide repeat (TPR) protein was induced 2.36-fold in NSG19, while it was reduced in KDML105 and IR20 (). Proteins containing TPR motifs can act as molecular chaperones and regulate the cell cycle (D'Andrea & Regan Citation2003). The TPR protein also plays an important role in hormone signaling under stress (Rosado et al. Citation2006) and is also induced in perennial rye grass under drought stress (Liu & Jiang Citation2010). In addition, a protein similar to coronatine-insensitive 1 (COI1), which thought to be involved in stomatal closing, was increased about 1.8-fold, after treatment (). The COI1 protein is a part of the SCFCOI1-ubiquitin ligase complex that catalyzes ubiquitination of the JAZ repressor. Degradation of JAZ repressor triggers the induction of jasmonate response genes and subsequently results in an increase in the innate immune response and stomatal closure (Munemasa et al. Citation2007; Melotto et al. Citation2008). Our results also demonstrate that stomata closure in NSG19 occurred faster than in KDML105 and IR20 (). Thus, an increase in COI1 proteins might involve with rapid stomata closure to limit water loss via guard cells to help maintain water balance in photosynthetic cells. This may result in protection of photosynthetic activity, as shown by the stability of Fv/Fm in NSG19 under drought stress ().
4.5. Unknown and common stress-inducible proteins
We were able to identify not only proteins known to play important roles in stress responses but also hypothetical or unknown proteins that may have critical functions. Results from our preliminary analysis indicate that among the 623 detected proteins, 254 proteins were hypothetical or unknown proteins and 23 of those proteins have significant differential expression patterns in the three rice cultivars. Those proteins were induced more than three to four times in a specific cultivar, indicating cultivar-specific functions under water stress. Although many stress-responsive proteins with unknown functions have been reported, only a few proteins have been further characterized. Overexpression of a novel protein of unknown function may result in increased tolerance to environmental stresses, as shown for overexpression of AtSAP10 (a novel stress associated protein) and OsCEST (a novel chloroplast protein in rice; chloroplast protein-enhancing stress tolerance) in Arabidopsis (Dixit & Dhankher Citation2011; Yokotani et al. Citation2011). Moreover, we also observed proteins that are expressed in a similar pattern in all three rice cultivars under water stress, indicating that in addition to cultivar-specific response there is also common response to water stress. Therefore, studies of these proteins may provide new candidate genes for future rice improvement.
5. Conclusion
In summary, this study suggests that PEG6000 is a better osmoticum for drought stress studies in rice seedlings than mannitol and that a complex mixture of protein samples from rice leaves can be effectively analyzed using GeLC-MS/MS shotgun proteomics together with an appropriate bioinformatics. We also report for the first time the proteomes under water stress of Thai jasmine rice (KDML105) and the two indica cultivars (NSG19 and IR20) that have been used as check cultivars for breeding drought-tolerant rice. The different responses to drought stress in these three contrasting cultivars were revealed by changes in Fv/Fm, stomatal closure, and protein expression patterns. For future perspective, proteins that exhibited specific highly increase under drought stress in the tolerant cultivars would have potential for developing to a drought-tolerant marker. This study suggests avenues for further research that may lead to a comprehensive understanding of the highly-complex drought stress responses in each of these rice cultivars.
Acknowledgements
This research was supported by Mahidol University and the program Strategic Scholarships for Frontier Research Network for the Joint Ph.D. Program Thai Doctoral degree. We are also thankful to the Office of the Higher Education Commission, Thailand and BIOTEC, NSTDA.
References
- Ali GM, Komatsu S. 2006. Proteomic analysis of rice leaf sheath during drought stress. J Proteome Res. 5:396–403. doi: 10.1021/pr050291g
- Baker NR. 2008. Chlorophyll fluorescence: a probe of photosynthesis in vivo. Annu Rev Plant Biol. 59:89–113. doi: 10.1146/annurev.arplant.59.032607.092759
- Banuelos MA, Garciadeblas B, Cubero B, Rodriguez-Navarro A. 2002. Inventory and functional characterization of the HAK potassium transporters of rice. Plant Physiol. 130:784–95. doi: 10.1104/pp.007781
- Blum H, Beier H, Gross H. 1987. Improved silver staining of plant protein, RNA and DNA in polyacrylamide gels. Electrophoresis. 8:93–9. doi: 10.1002/elps.1150080203
- Bosch M, Mayer CD, Cookson A, Donnison IS. 2011. Identification of genes involved in cell wall biogenesis in grasses by differential gene expression profiling of elongating and non-elongating maize internodes. J Exp Bot. 62:3545–61. doi: 10.1093/jxb/err045
- Cabuslay GS, Ito O, Alejar AA. 2002. Physiological evaluation of responses of rice (Oryza sativa L.) to water deficit. Plant Sci. 163:815–27. doi: 10.1016/S0168-9452(02)00217-0
- Cao PJ, Bartley LE, Jung KH, Ronald PC. 2008. Construction of a rice glycosyltransferase phylogenomic database and identification of rice-diverged glycosyltransferases. Mol Plant. 1:858–77. doi: 10.1093/mp/ssn052
- Cha-um S, Supaibulwatana K, Kirdmanee C. 2006. Water relation, photosynthetic ability and growth of Thai jasmine rice (Oryza sativa L. ssp. indica cv. KDML105) to salt stress by application of exogenous glycinebetaine and choline. J Agron Crop Sci. 192:25–36. doi: 10.1111/j.1439-037X.2006.00186.x
- Cha-um S, Yooyongwech S, Supaibulwatana K. 2010. Water deficit stress in the reproductive stage of four indica rice (Oryza Sativa L.) genotypes. Pak J Bot. 42:3387–98.
- Chaves MM, Maroco JP, Pereira JS. 2003. Understanding plant responses to drought – from genes to the whole plant. Funct Plant Biol. 30:239–64. doi: 10.1071/FP02076
- D'Andrea LD, Regan L. 2003. TPR proteins: the versatile helix. Trends Biochem Sci. 28:655–62. doi: 10.1016/j.tibs.2003.10.007
- Delmotte N, Lasaosa M, Tholey A, Heinzle E, van Dorsselaer A, Huber CG. 2009. Repeatability of peptide identifications in shotgun proteome analysis employing off-line two-dimensional chromatographic separations and ion-trap MS. J Sep Sci. 32:1156–64. doi: 10.1002/jssc.200800615
- Dixit AR, Dhankher OP. 2011. A novel stress-associated protein ‘AtSAP10’ from Arabidopsis thaliana confers tolerance to nickel, manganese, zinc, and high temperature stress. PLoS ONE. 6:e20921. DOI 10.1371/journal.pone.0020921.
- Efeoglu B, Ekmekçi Y, Çiçek N. 2009. Physiological responses of three maize cultivars to drought stress and recovery. S Afr J Bot. 75:34–42. doi: 10.1016/j.sajb.2008.06.005
- Garg AK, Kim JK, Owens TG, Ranwala AP, Choi YD, Kochian LV, Wu RJ. 2002. Trehalose accumulation in rice plants confers high tolerance levels to different abiotic stresses. Proc Natl Acad Sci USA. 99:15898–903. doi: 10.1073/pnas.252637799
- Giardi MT, Cona A, Geiken B, Kucera T, Masojidek J, Mattoo AK. 1996. Long-term drought stress induces structural and functional reorganization of photosystem II. Planta. 199:118–25. doi: 10.1007/BF00196888
- Gilmore JM, Washburn MP. 2010. Advances in shotgun proteomics and the analysis of membrane proteomes. J Proteomics. 73:2078–91. doi: 10.1016/j.jprot.2010.08.005
- Granier F. 1988. Extraction of plant proteins for two-dimensional electrophoresis. Electrophoresis. 9:712–8. doi: 10.1002/elps.1150091106
- Hashiguchi A, Ahsan N, Komatsu S. 2010. Proteomics application of crops in the context of climatic changes. Food Res Int. 43:1803–13. doi: 10.1016/j.foodres.2009.07.033
- Hohl M, Schopfer P. 1991. Water relations of growing maize coleoptiles: comparison between mannitol and polyethylene glycol 6000 as external osmotica for adjusting turgor pressure. Plant Physiol. 95:716–22. doi: 10.1104/pp.95.3.716
- Iwamoto M, Higo K, Takano M. 2009. Circadian clock- and phytochrome-regulated Dof-like gene, Rdd1, is associated with grain size in rice. Plant Cell Environ. 32:592–603. doi: 10.1111/j.1365-3040.2009.01954.x
- Johansson C, Samskog J, Sundstrom L, Wadensten H, Bjorkesten L, Flensburg J. 2006. Differential expression analysis of Escherichia coli proteins using a novel software for relative quantitation of LC-MS/MS data. Proteomics. 6:4475–85. doi: 10.1002/pmic.200500921
- Khan MM, Komatsu S. 2004. Rice proteomics: recent developments and analysis of nuclear proteins. Phytochemistry. 65:1671–81. doi: 10.1016/j.phytochem.2004.04.012
- Kim S, Wang Y, Wu J, Kang K, Kim S. 2011. Physiological and proteomic analysis of young rice leaves grown under nitrogen-starvation conditions. Plant Biotechnol Rep. 5:309–15. doi: 10.1007/s11816-011-0184-1
- Lee S, Lee J, Paek KH, Kwon SY, Cho HS, Kim SJ, Park JM. 2010. A novel WD40 protein, BnSWD1, is involved in salt stress in Brassica napus. Plant Biotechnol Rep. 4:165–72. doi: 10.1007/s11816-010-0131-6
- Li R, Pei-pol G, Baumz M, Grando S, Ceccarelli S. 2006. Evaluation of chlorophyll content and fluorescence parameters as indicators of drought tolerance in barley. Agr Sci China. 5:751–7. doi: 10.1016/S1671-2927(06)60120-X
- Lichtenthaler HK. 1987. Chlorophylls and carotenoids: pigments of photosynthetic biomembranes. Methods Enzymol. 148:350–80.
- Liu S, Jiang Y. 2010. Identification of differentially expressed genes under drought stress in perennial ryegrass. Physiol Plant. 139:375–87. doi: 10.1111/j.1399-3054.2009.01341.x
- Liu S-H, Fu B-Y, Xu H-X, Zhu L-H, Zhai H-Q, Li Z-K. 2007. Cell death in response to osmotic and salt stresses in two rice (Oryza sativa L.) ecotypes. Plant Sci. 172:897–902. doi: 10.1016/j.plantsci.2006.12.017
- Lowry OH, Rosebrough NJ, Farr AL, Randall RJ. 1951. Protein measurement with the Folin phenol reagent. J Biol Chem. 193:265–75.
- Malmstrom J, Lee H, Aebersold R. 2007. Advances in proteomic workflows for systems biology. Curr Opin Biotechnol. 18:378–84. doi: 10.1016/j.copbio.2007.07.005
- Matros A, Kaspar S, Witzel K, Mock HP. 2011. Recent progress in liquid chromatography-based separation and label-free quantitative plant proteomics. Phytochemistry. 72:963–74. doi: 10.1016/j.phytochem.2010.11.009
- Melotto M, Underwood W, He SY. 2008. Role of stomata in plant innate immunity and foliar bacterial diseases. Annu Rev Phytopathol. 46:101–22. doi: 10.1146/annurev.phyto.121107.104959
- Money NP. 1989. Osmotic pressure of aqueous polyethylene glycols: relationship between molecular weight and vapor pressure deficit. Plant Physiol. 91:766–9. doi: 10.1104/pp.91.2.766
- Munemasa S, Oda K, Watanabe-Sugimoto M, Nakamura Y, Shimoishi Y, Murata Y. 2007. The coronatine-insensitive 1 mutation reveals the hormonal signaling interaction between abscisic acid and methyl jasmonate in arabidopsis guard cells. Specific impairment of ion channel activation and second messenger production. Plant Physiol. 143:1398–407. doi: 10.1104/pp.106.091298
- Nguyen GN, Hailstones DL, Wilkes M, Sutton BG. 2010. Drought stress: role of carbohydrate metabolism in drought-induced male sterility in rice anthers. J Agron Crop Sci. 196:346–57. doi: 10.1111/j.1439-037X.2010.00423.x
- Pandey R, Agarwal RM, Jeevaratnam K, Sharma GL. 2004. Osmotic stress-induced alterations in rice (Oryza sativa L.) and recovery on stress release. Plant Growth Regul. 42:79–87. doi: 10.1023/B:GROW.0000014893.45112.55
- Pandey S, Bhandari H, Hardy B. 2007. Economic costs of drought and rice farmers' coping mechanisms: a cross-country comparative analysis. Philippines: International Rice Research Institute.
- Perkins DN, Pappin DJC, Creasy DM, Cottrell JS. 1999. Probability-based protein identification by searching sequence databases using mass spectrometry data. Electrophoresis. 20:3551–67. doi: 10.1002/(SICI)1522-2683(19991201)20:18%3C3551::AID-ELPS3551%3E3.0.CO;2-2
- Piersma SR, Fiedler U, Span S, Lingnau A, Pham TV, Hoffmann S, Kubbutat MHG, Jiménez CR. 2010. Workflow comparison for label-free, quantitative secretome proteomics for cancer biomarker discovery: method evaluation, differential analysis, and verification in serum. J Proteome Res. 9:1913–22. doi: 10.1021/pr901072h
- Pieters AJ, Souki SE. 2005. Effects of drought during grain filling on PSII activity in rice. J Plant Physiol. 162:903–11. doi: 10.1016/j.jplph.2004.11.001
- Reddy PCO, Sairanganayakulu G, Thippeswamy M, Reddy PS, Reddy MK, Sudhakar C. 2008. Identification of stress-induced genes from the drought tolerant semi-arid legume crop horsegram (Macrotyloma uniflorum (Lam.) Verdc.) through analysis of subtracted expressed sequence tags. Plant Sci. 175:372–84. doi: 10.1016/j.plantsci.2008.05.012
- Rezaul K, Wu L, Mayya V, Hwang SI, Han D. 2005. A systematic characterization of mitochondrial proteome from human T leukemia cells. Mol Cell Proteomics. 4:169–81.
- Rosado A, Schapire AL, Bressan RA, Harfouche AL, Hasegawa PM, Valpuesta V, Botella MA. 2006. The arabidopsis tetratricopeptide repeat-containing protein TTL1 is required for osmotic stress responses and abscisic acid sensitivity. Plant Physiol. 142:1113–26. doi: 10.1104/pp.106.085191
- Sabot F, Simon D, Bernard M. 2004. Plant transposable elements, with an emphasis on grass species. Euphytica. 139:227–47. doi: 10.1007/s10681-004-3179-y
- Salekdeh GH, Siopongco J, Wade LJ, Ghareyazie B, Bennett J. 2002. Proteomic analysis of rice leaves during drought stress and recovery. Proteomics. 2:1131–45. doi: 10.1002/1615-9861(200209)2:9%3C1131::AID-PROT1131%3E3.0.CO;2-1
- Santoni V, Molloy M, Rabilloud T. 2000. Membrane proteins and proteomics: un amour impossible? Electrophoresis. 21:1054–70. doi: 10.1002/(SICI)1522-2683(20000401)21:6%3C1054::AID-ELPS1054%3E3.0.CO;2-8
- Sarkarung S. 2003. A regional breeding program to develop drought-tolerant rainfed lowland germplasm: IRRI's experience in South and Southeast Asia. In: Fischer KS, Lafitte R, Fukai S, Atlin G, Hardy B, editors. Breeding rice for drought-prone environments. Philippines: International Rice Research Institute, p. 70–4.
- Sayed OH. 2003. Chlorophyll fluorescence as a tool in cereal crop research. Photosynthetica. 41:321–30. doi: 10.1023/B:PHOT.0000015454.36367.e2
- Shabala SN, Shabala SI, Martynenko AI, Babourina O, Newman IA. 1998. Salinity effect on bioelectric activity, growth, Na+ accumulation and chlorophyll fluorescence of maize leaves: a comparative survey and prospects for screening. Aust J Plant Physiol. 25:609–16. doi: 10.1071/PP97146
- Slama I, Ghnaya T, Hessini K, Messedi D, Savouré A, Abdelly C. 2007. Comparative study of the effects of mannitol and PEG osmotic stress on growth and solute accumulation in Sesuvium portulacastrum. Environ Exp Bot. 61:10–7. doi: 10.1016/j.envexpbot.2007.02.004
- Tabb DL, Vega-Montoto L, Rudnick PA, Variyath AM, Ham AJ, Bunk DM, Kilpatrick LE, Billheimer DD, Blackman RK, Cardasis HL, et al 2010. Repeatability and reproducibility in proteomic identifications by liquid chromatography-tandem mass spectrometry. J Proteome Res. 9:761–76. doi: 10.1021/pr9006365
- Tepperman JM, Zhub T, Chang HS, Wang X, Quail PH. 2001. Multiple transcription-factor genes are early targets of phytochrome A signaling. Proc Natl Acad Sci USA. 98:9437–42. doi: 10.1073/pnas.161300998
- Thorsell A, Portelius E, Blennow K, Westman BA. 2007. Evaluation of sample fractionation using microscale liquid-phase isoelectric focusing on mass spectrometric identification and quantitation of proteins in a SILAC experiment. Rapid Commun Mass Spectrom. 21:771–8. doi: 10.1002/rcm.2898
- Vashisht AA, Tuteja N. 2006. Stress responsive DEAD-box helicases: a new pathway to engineer plant stress tolerance. J Photochem Photobiol B. 84:150–60. doi: 10.1016/j.jphotobiol.2006.02.010
- Vassileva V, Demirevska K, Simova-Stoilova L, Petrova T, Tsenov N, Feller U. 2012. Long-term field drought affects leaf protein pattern and chloroplast ultrastructure of winter wheat in a cultivar-specific manner. J Agron Crop Sci. 198:104–17. doi: 10.1111/j.1439-037X.2011.00492.x
- Wang J, Hou B. 2009. Glycosyltransferases: key players involved in the modification of plant secondary metabolites. Front Biol China. 4:39–46. doi: 10.1007/s11515-008-0111-1
- Wang W, Vinocur B, Altman A. 2003. Plant responses to drought, salinity and extreme temperatures: towards genetic engineering for stress tolerance. Planta. 218:1–14. doi: 10.1007/s00425-003-1105-5
- Washburn MP, Wolters D, Yates III JR. 2001. Large-scale analysis of the yeast proteome by multidimensional protein identification technology. Nat Biotechnol. 19:242–7. doi: 10.1038/85686
- Xiong JH, Fu BY, Xu HX, Li YS. 2010. Proteomic analysis of PEG-simulated drought stress responsive proteins of rice leaves using a pyramiding rice line at the seedling stage. Bot Stud. 51:137–45.
- Yamane K, Kawasaki M, Taniguchi M, Miyake H. 2003. Differential effect of NaCl and polyethylene glycol on the ultrastructure of chloroplasts in rice seedlings. J Plant Physiol. 160:573–5. doi: 10.1078/0176-1617-00948
- Yanagisawa S. 2004. Dof domain proteins: plant-specific transcription factors associated with diverse phenomena unique to plants. Plant Cell Physiol. 45:386–91. doi: 10.1093/pcp/pch055
- Yates III JR. 1998. Mass spectrometry and the age of the proteome. J Mass Spectrom. 33:1–19. doi: 10.1002/(SICI)1096-9888(199801)33:1%3C1::AID-JMS624%3E3.0.CO;2-9
- Yee D, Goring DR. 2009. The diversity of plant U-box E3 ubiquitin ligases: from upstream activators to downstream target substrates. J Exp Bot. 60:1109–21. doi: 10.1093/jxb/ern369
- Yokotani N, Higuchi M, Kondou Y, Ichikawa T, Iwabuchi M, Hirochika H, Matsui M, Oda K. 2011. A novel chloroplast protein, CEST induces tolerance to multiple environmental stresses and reduces photooxidative damage in transgenic Arabidopsis. J Exp Bot. 62:557–69. doi: 10.1093/jxb/erq290
- Yoshida S. 1981. Fundamentals of rice crop science. Philippines: International Rice Research Institute.
- Zang X, Komatsu S. 2007. A proteomics approach for identifying osmotic-stress-related proteins in rice. Phytochemistry. 68:426–37. doi: 10.1016/j.phytochem.2006.11.005
- Zhao Y, Zhou D-X. 2012. Epigenomic modification and epigenetic regulation in rice. J Genet Genomics. 39:307–15. doi: 10.1016/j.jgg.2012.02.009
- Zheng J, Zhao J, Tao Y, Wang J, Liu Y, Fu J, Jin Y, Gao P, Zhang J, Bai Y, Wang G. 2004. Isolation and analysis of water stress induced genes in maize seedlings by subtractive PCR and cDNA macroarray. Plant Mol Biol. 55:807–23.