Abstract
Nonstructural carbohydrate (NSC) accumulation in submergence tolerant rice cultivars (cv) was studied in six Indica rice [Oryza sativa (L.)] cv under control and simulated submerged conditions. Tolerant cultivars accumulated greater contents of NSC compared to the susceptible cultivars. Starch and total NSC content showed significant positive association with survival percentage. On the other hand, elongation due to submergence was significantly a negative association with survival. The CO2 photosynthetic rate, chlorophyll content, maximum photochemical efficiency of PS II (Fv/Fm), and activities of Rubisco were not significantly different between tolerant and susceptible cv under control condition. The ADP glucose pyrophosphorylase (AGPPase) activity was significantly higher in the tolerant cv and was a positive association with starch/NSC, whereas Fructose 1,6-diphosphatase (FDPase) activity was significantly higher in susceptible cv compared to tolerant cv and was a negative association with starch/NSC. Greater activities of AGPPase along with lower activities of FDPase might facilitate greater accumulation of NSC in tolerant rice cultivars.
Abbreviations | ||
AGPPase | = | ADP glucose pyrophosphorylase |
cv | = | cultivar |
d | = | days |
FDPase | = | Fructose 1,6-diphosphatase |
LSD | = | least significant difference |
NSC | = | nonstructural carbohydrate |
PS | = | photosystem |
Rubisco | = | ribulose 1,5-bisphosphate carboxylase/oxygenase |
RUBP | = | ribulose 1,5-bisphosphate |
SD | = | standard deviation |
SSyn | = | sucrose synthase |
SPSyn | = | sucrose phosphate synthase |
Introduction
Rice production is adversely affected by numerous abiotic stresses such as submergence, waterlogging, drought, and salinity, which normally prevail through part or most of the season. Climate change is anticipated to lead to greater variability in hydrometeorological aberration, resulting in increased frequencies of extreme events, such as flash floods, with fresh or saline water, higher temperatures, salt intrusion, and in some areas, even longer dry spells. Nonstructural carbohydrate (NSC) content is crucial in surviving plant under different abiotic stresses in making agriculture sustainable (Mostajeran & Rahimi-Eichi Citation2009; Peleg et al. Citation2011). Tropical Asian rice varieties, immensely variable in many traits, were all similar in plant type. The tall, lodging susceptible rice had a low yield potential that could not be increased significantly when provided with improved crop management. However, it does not mean that the traditional varieties are inferior compared to fertilizers responsive high-yielding rice cultivars, rather these possess so many important traits, which could improve the rice yield both under nonstress and stressfull environmental conditions (Nagata Citation2006; Xu et al. Citation2006; Sarkar et al. Citation2009). In cereals such as rice, the process of grain filling demands carbon from photosynthesis assimilation as well as the remobilization of prestored carbohydrates from two organs, i.e. mature leaves and leaf sheaths plus culms. NSCs in leaf sheaths and culms are mainly translocated to panicles and play an important role in compensating for the lack of a source supply by photosynthesis after heading (Yang & Zhang Citation2006). It has been estimated that about 30% of the total yield in rice depends on the carbohydrate content accumulated in the stem before heading (Calviño et al. Citation2008). When photosynthesis is decreased by different adverse conditions after heading, the carbohydrate accumulated before heading increases in importance in grain filling. Increasing the capacity of the leaf to store greater quantity of NSC is one of the main targets in the improvement of rice yield (Dingkuhn et al. Citation1991; Moayedi et al. Citation2009). NSC accumulation is particularly important for grain filling in high-yielding cultivars that bear a large number of spikelets, which require a large supply of carbohydrates (Ishimaru et al. Citation2007) and, therefore, mechanism involved in carbohydrate metabolism and accumulation can lead to the development of improved rice crops.
Submergence tolerance is a metabolic adaptation in response to anaerobiosis that enables cells to maintain their integrity such that the plant survives hypoxia without major damage. Plants that survive or succumb to transient submergence differ in the timing and duration of carbohydrate consumption and anaerobic metabolism (Fukao & Bailey-Serres Citation2004; Bailey-Serres & Voesenek Citation2008). NSC concentration before and during submergence has long been recognized as an important factor in submergence tolerance in rice. These NSCs are used up during submergence and supply the required energy for survival (Das et al. Citation2005; Nagai et al. Citation2010), and management of carbohydrates during submergence appears to be crucial for tolerance (Fukao & Bailey-Serres Citation2004). Maintenance of high levels of stored carbohydrates in the seedlings prior to submergence coupled with minimum shoot elongation and retention of chlorophyll are all desirable traits for submergence tolerance (Fukao et al. Citation2006; Hattori et al. Citation2009). Indeed, extensive shoot elongation is not desired under flash flood conditions as it exhausts energy storage. However, it should be remembered that the fast growth of cultivars adapted to deep water conditions allows rice to remain above water level, where it can breathe and avoid drowning (Nagai et al. Citation2010). Biotechnological and genetic approaches to enhance the efficiency of pathways involved in carbohydrate metabolism under submergence could help in developing germplasm with enhanced tolerance (Das et al. Citation2005).
The mechanism under laying this phenomenon has not been worked out so far. Identification of an enzyme, or set of enzymes, which help in accumulation of NSC would be useful in identification of genes responsible for higher accumulation of NSC (Calviño et al. Citation2008). Understanding the physiological and biochemical mechanism for cv differences in NSC accumulation in rice seedlings may facilitate future breeding program not only to develop cultivars tolerant to complete submergence but also lead to develop high-yielding rice cultivars adapted to fluctuating environment. So the present investigation was undertaken to study NSC accumulation in relation to photosynthesis and key enzymes for carbohydrate metabolism, which could provide evidences for unraveling the mechanism behind the greater accumulation of NSC at vegetative stage in rice.
Materials and methods
Plant material and growth conditions
The experiment was conducted with six Indica rice cv [Oryza sativa (L.)], namely, FR 13A, Kalaputia, Khoda, and Khadara (tolerant to submergence), Sarala and IR 42 (susceptible to submergence). All cultivars were sown directly in earthen pots containing 2 kg of farm soil and farmyard manure in a 3:1 ratio. Each pot was supplied with 80 mg urea, 192 mg single super phosphate (P2O5), and 70 mg murate of potash (K2O). Plants were thinned to five seedlings per pot 10 d after germination. Plants were grown in a greenhouse, subjected to natural solar radiation, with daily maximum photosynthetic photon flux density, air temperature, and relative humidity being about 1450 µmol m−2 s−1, 34.6°C, and 70–75%, respectively. Twenty-one-day-old seedlings were completely submerged for 8 d in a concrete tank under 110 cm depth of water. Measurements were made without submergence (control) condition and 8 d after submergence. Plant survival was recorded 8 d after submergence as reported by Panda et al. (Citation2008) and is defined as the ability of a seedling to produce new, growing leaves. The experiments were carried out in three replications and were statistically analyzed.
Measurement of NSC content and plant height
NSC (soluble sugar and starch) content of the shoot was estimated following the procedure of Yoshida et al. (Citation1976) with three replications. Briefly, for each measurement, shoot samples of five plants were oven-dried and ground to a fine powder and extracted, using 80% ethanol (v/v). The extract was then used for soluble sugar analysis after an addition of anthrone reagent, followed by a measurement of absorbance at 630 nm, using a spectrophotometer (model SL 164 double beam, ELICO, Hyderabad, India). The residue remaining after soluble sugars extraction was dried and extracted using perchloric acid and then analyzed for starch (as glucose equivalent), using the anthrone reagent as for soluble sugars. Plant height and extent of elongation of the plant shoot were determined by subtracting plant height of respecting control plant from that after submergence and expressing it as percentage of elongation due to submergence.
Measurement of leaf CO2 photosynthetic rate, chlorophyll fluorescence, and chlorophyll content
Measurements of CO2 photosynthetic rate were made on the fully expanded leaves of three different plants, using an open system photosynthetic gas analyzer (PP Systems, USA) under normal ambient environmental conditions. The second and third leaf from the top were selected and kept inside the chamber under natural irradiance until stable reading was recorded as described by Panda et al. (Citation2008).
After measuring the CO2 photosynthetic rate, the same leaves were used for the measurement of chlorophyll fluorescence, using a Plant Efficiency Analyzer, Handy PEA (Hansatech Instruments Ltd., Norfolk, UK). Leaves were maintained in darkness for 20 min before taking the data on chlorophyll fluorescence. The maximal intensity of the light source, providing an irradiance saturating pulse of 3000 µmol photons m−2 s−1 was used. Different chlorophyll fluorescence parameters such as minimal fluorescence (Fo), maximal fluorescence (Fm), variable fluorescence (Fv = Fm − Fo), maximum photochemical efficiency of PS II (Fv/Fm) were calculated using the software supplied by the manufacture.
For chlorophyll estimation, 100 mg of finely chopped fresh leaves were placed in a 25 mL capped measuring tube containing 25 mL of 80% cold acetone and kept dark inside a refrigerator (4 °C) for 28 h (Panda et al. Citation2008). The chlorophyll was measured spectrophotometrically using a spectrophotometer (model SL 164 double beam, ELICO, Hyderabad, India) following the procedure of Porra (Citation2002).
Assay of carbohydrate metabolic enzymes
Rubisco (EC 4.1.1.39) activity was measured following the method of Yu-Chun et al. (Citation1996) with some minor modifications described in Panda et al. (Citation2008). The activity was expressed as µmol NADH oxidized min−1 mg−1 protein by using extinction coefficient of 6.22 µmol cm−1 s−1.
ADP glucose pyrophosphorylase (AGPPase, EC 2.7.7.27) activity was measured according to the method of Racker (Citation1962). The activity was expressed as µmol NAD+ reduced min−1 mg−1 protein by using extinction coefficient of 6.22 µmol cm−1 s−1.
Sucrose phosphate synthase (SPSyn, EC 2.4.1.14) and sucrose synthase (SSyn, EC 2.4.1.13) activities were determined by Rocher et al. (Citation1989). Results were obtained from a standard curve of different sucrose concentrations at 520 nm. Enzyme activity was expressed in mM sucrose synthesized h−1 mg−1 protein.
The assay of fructose 1,6-diphosphatase (FDPase, EC 3.1.3.11) was based on the method developed by Racker (Citation1962). Enzyme activity was expressed as mg inorganic phosphorous released h−1 mg−1 protein.
Enzyme invertase (EC 3.2.1.26) was assayed according to the procedure of Sadasivum and Manickum (Citation1997). Using standard curve of glucose, the enzyme activity was expressed as mg glucose released h−1 mg−1 protein.
Statistical analysis
Differences between various physiological and biochemical parameters were compared by ANOVA using IRRISTAT (International Rice Research Institute, Philippines) software's least significant difference (LSD), as this is a good test for determining whether means were significantly different. Correlation coefficients and regression analysis were done following the standard procedure.
Results and discussion
Submergence imposes a complex abiotic stress (Bailey-Serres & Voesenek Citation2008; Nagai et al. Citation2010), and the extent of injury caused by complete submergence is largely depends on floodwater conditions (Das et al. Citation2009). In this experiment, the six cultivars gave distinctly different response to submergence in terms of survival. Tolerant cv FR 13A and Kalaputia showed more than 85% and Khoda and Khadara showed more than 70% of survival after 8 d of complete submergence, whereas it was less than 15% in IR 42 and Sarala (). NSC contents before and after submergence are important for providing energy needed for maintenance metabolism during submergence and for regeneration and recovery of seedlings after submergence (Das et al. Citation2005; Panda et al. Citation2008). In the present study, tolerant cv had significantly more NSC (sugar and starch) content compared to the susceptible cv both without submergence (control) and 8 d after submergence treatment (). A critical evaluation of submergence-tolerant and -intolerant rice cv reveals that seedlings of tolerant species have 30–50% more NSC compared to the susceptible cv (Das et al. Citation2005). Starch and total NSC content were showed significant positive association with survival percentage (r=0.992**, 0.870**, respectively) (). On the other hand, percentage of shoot elongation due to submergence was significantly negative association with a survival percentage r= − 0.958** as well as sugar, starch, and total NSC content r= − 0.523**,−0.945**,−0.992**, respectively (). Percentage of shoot elongation due to submergence varies from 79 to 94% in susceptible cv, but it was 25–35% in tolerant cv, whereas percentage of decrease in NSC content was 32–35% and 13–17% in susceptible and tolerant cv, respectively (). Generally, our results demonstrated that elongation competes for essential energy supply and that reducing elongation can conserve carbohydrates and increase submergence tolerance as Fukao et al. (Citation2006) demonstrated that genotypes lacking submergence-induced Sub 1A-1 rapidly consume leaf starch and soluble sugars to maintain elongation growth during submergence. By contrast, genotypes with the Sub 1 haplotype introgressed from FR13A consume carbohydrate energy reserves more slowly during submergence, maintaining growth at a rate similar to plants in air.
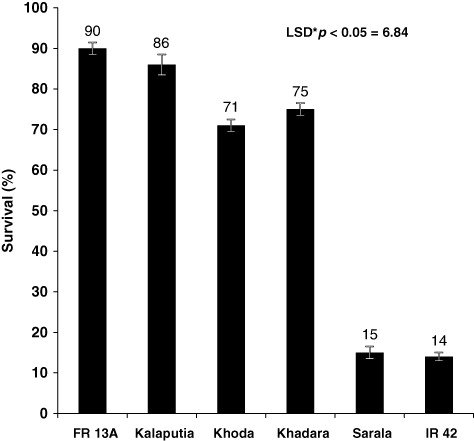
Table 1. NSC (sugar and starch) content and plant height in different rice cultivars (21-days-old) without submergence (C) and 8 d after submergence (S).
The CO2 photosynthetic rate, chlorophyll content, and maximum photochemical efficiency of PS II (Fv/Fm) were not significantly different between tolerant and susceptible cv under control condition (). Similar to other abiotic stresses, leaf photosynthesis is one of the earliest plant responses to submergence, and submergence resulted in significant reduction of CO2 photosynthetic rate after 8 d submergence in both susceptible and tolerant cv along with the decrease of chlorophyll (). But the tolerant cultivars maintained significantly higher values of these after 8 d submergence. The Fv/Fm ratio is the most widely studied chlorophyll fluorescence parameter under stress. Decrease in Fv/Fm ratio is indicative of photoinhibition as well as damage to photosynthetic apparatus (Meheta et al. Citation2010). It is also known to decrease due to different abiotic stresses (Sayed Citation2003). The Fv/Fm ratio did not change much under submergence compared to control plants, however, there was a significant reduction of the values of Fv/Fm after 8 d submergence for the susceptible cv – Sarala and IR 42 – only ().
Table 2. CO2 photosynthetic rate, chlorophyll content, and maximum photochemical efficiency of PS II (Fv/Fm) in different rice cultivars (21-days-old) without submergence (C) and 8 d after submergence (S).
Plant growth is not only regulated by carbon assimilation but also carbon partitioning. Leaf photosynthesis is influenced by primarily on carbon fixation enzymes and carbohydrate levels in leaf and source–sink balance (Prasad et al. Citation2004). Photosynthetically fixed carbon is partitioned into sucrose and starch: sucrose is exported to sink organs, whereas starch is stored transiently in chloroplast (Zeeman et al. Citation2007). Carbon partitioning between starch and sucrose appears to be genetically controlled (Huber Citation1983). SPSyn is a key regulatory enzyme in the partitioning of photoassimilate to either sucrose or starch in leaves, whereas AGPPase is a key regulatory enzyme in starch synthesis (Gesch Citation2002). The flux of carbon into the starch biosynthetic pathway in leaves was thought to be controlled by modulation of AGPPase activity (Gesch Citation2002). Sucrose is converted to UDP-glucose and fructose by SSyn and to glucose and fructose by invertase (Fernie et al. Citation2002). The cytosolic FDPase catalyzes the step of triose phosphate conversion to sucrose (Daie Citation1993). The activities of Rubisco, SSyn, invertase, and SPSyn in different rice cultivars under control and submergence conditions are presented in . Rubisco activity was significantly decreased under submergence in both the tolerant and susceptible cv compared to the control plant (). AGPPase activity was found to be significantly higher in the tolerant cv compared to the susceptible cv both control and 8 d after submergence (). FDPase activity was significantly higher in susceptible cv compared to tolerant cv both control and 8 d after submergence and showed negative association with starch/NSC accumulation (). It has been reported that decreased cFDPase expression leads to restriction in the conversion of triose-P to sucrose and stimulation of starch synthesis (Strand et al. Citation2000). The results of the present study correlate higher FDPase activity and also AGPPase reduction with low accumulation of starch in sensitive rice varieties. However, some reports conclude that loss of cFDPase limits sucrose synthesis and cause growth retardation in rice (Lee et al. Citation2008). Greater activities of AGPPase along with lower activities of FDPase might facilitate in translocating the newly synthesized photosynthates from the site of synthesis, which in turn helped in the accumulation of greater quantities of NSC in leaf sheaths and culm. Sucrose, which is the major energy source and transport form of carbohydrates in rice, can be hydrolyzed via two distinct pathways: the more energy-efficient SSyn pathway and the invertase pathway (Fukao & Bailey-Serres Citation2004). SSyn activity was not changed due to submergence except cv khadara, the activity was increased after submergence compared to the control plant, but the activity was not different among tolerant and susceptible cv (). The tolerant cv FR 13A and susceptible cv IR 42 showed significantly higher SPSyn activity compared to other cultivars under control but after submergence activity was not significantly different among tolerant and susceptible cv (). Invertase activity was significantly increased after submergence in IR 42 and Khadara over control plants (). It has been shown that the transcript level and the enzymatic activity of SSyn increase, whereas those of invertase decrease, during oxygen deprivation in maize (Zea mays) roots, and potato (Solanum tuberosum) tubers, suggesting that SSyn is the principal enzyme that converts sucrose to phosphorylated hexose sugars under low oxygen stress (Fukao & Bailey-Serres Citation2004). Carbohydrate utilization and transport are likely to be up regulated to accommodate excess assimilate resulting from increase photosynthetic rate and rice accumulates sucrose in response to high photosynthetic rate (Gesch Citation2002). The association of sugar content with photosynthetic rate and Fv/Fm ratio was negatively significant (). Long et al. (Citation2006) suggested that lack of correlation between leaf photosynthesis and yield and biomass. In the present study, the depletion of sugar content was less than that of photosynthetic rate and Fv/Fm ratio in rice during submergence.
Table 3. Activities of different carbohydrate metabolic enzymes in different rice cultivars (21-days-old) without submergence (C) and 8 d after submergence (S).
Table 4. Association of sugar, starch, and total NSC contents with different enzymes of carbohydrate metabolism, photosynthetic parameters, elongation (%), and survival (%) in different rice cultivars during vegetative stage.
In conclusion, the results of the present study support the view that greater activities of AGPPase along with lower activities of FDPase might facilitate greater accumulation of NSC in submergence-tolerant rice cultivars. Further research is needed aiming to identify and/or to modify crop cultivars, which may accumulate greater quantities of NSC more efficiently. It would not only help to develop cultivars tolerant to different abiotic stresses but also lead to develop high-yielding rice cultivars adapted to climate change.
Acknowledgements
The authors are grateful to the director, Central Rice Research Institute, Cuttack, for providing necessary facilities for the work.
References
- Bailey-Serres J, Voesenek LACJ. 2008. Flooding stress: acclimations and genetic diversity. Annu Rev Plant Biol. 59:313–339. 10.1146/annurev.arplant.59.032607.092752
- Calviño M, Bruggmann R, Messing J. 2008. Screen of genes linked to high-sugar content in stems by comparative genomics. Rice. 1:166–176. 10.1007/s12284-008-9012-9
- Daie J. 1993. Cytosolic Fructose-1, 6-bisphosphatase: a key enzymes in the sucrose biosynthetic pathway. Photosynthetic Res. 28:5–14. 10.1007/BF00015056
- Das KK, Panda D, Sarkar RK, Reddy JN, Ismail AM. 2009. Submergence tolerance in relation to variable floodwater conditions in rice. Environ Exp Bot. 66:425–434. 10.1016/j.envexpbot.2009.02.015
- Das KK, Sarkar RK, Ismail AM. 2005. Elongation ability and non-structural carbohydrate levels in relation to submergence tolerance in rice. Plant Sci. 168:131–136. 10.1016/j.plantsci.2004.07.023
- Dingkuhn M, Penning deVries FWT, De Datta SK, Van Laar HH. 1991. Concepts for a new plant type for direct seeded flooded tropical rice. In: Penning de Vries F, Teng P, Matselaar K, editors. Direct seeded flooded rice in the tropics. Los Baños: International Rice Research Institute; 17–38.
- Fernie AR, Willmitzer L, Trethewey RN. 2002. Sucrose to starch: a transition in molecular plant physiology. Trends in Plant Sci. 7:35–41. 10.1016/S1360-1385(01)02183-5
- Fukao T, Bailey-Serres J. 2004. Plant responses to hypoxia- is survival a balancing act? Trends in Plant Sci. 9:449–456. 10.1016/j.tplants.2004.07.005
- Fukao T, Xu K, Ronald PC, Bailey-Serres J. 2006. A variable cluster of ethylene response factor-like genes regulates metabolic and developmental acclimation responses to submergence in rice. The Plant Cell. 18:2021–2034. 10.1105/tpc.106.043000
- Gesch RW. 2002. Sucrose phosphate synthase activity in mature rice leaves following changes in growth CO2 unrelated to sucrose pool size. New Phytol. 184:77–84. 10.1046/j.1469-8137.2002.00348.x
- Hattori Y, Nagai K, Furukawa S, Song XJ, Kawano R, Sakakibara H, Wu J, Matsumoto T, Yoshimura A, Kitano H, et al. 2009. The ethylene response factors SNORKEL1 and SNORKEL2 allow rice to adapt to deep water. Nature. 460:1026–1030. 10.1038/nature08258
- Huber SC. 1983. Role of sucrose phosphate synthase in partitioning in carbon in leaves. Plant Physiol. 71:818–821. 10.1104/pp.71.4.818
- Ishimaru K, Hirotsu N, Madoka Y, Kashiwagi T. 2007. Quantitative trait loci for sucrose, starch and hexose accumulation before heading in rice. Plant Physiol Biochem. 45:799–804. 10.1016/j.plaphy.2007.07.004
- Lee SK, Jean J-S, Bornke F, Cho LVJ. 2008. Loss of cytosolic fructose-1, 6-bisphosphatase limit photosynthetic sucrose synthesis and cause severe growth retardation in rice (Oryza sativa). Plant Cell Environ. 31:1851–1863. 10.1111/j.1365-3040.2008.01890.x
- Long SP, Zhu X, Naidu SL, Ort DR. 2006. Can improvement in photosynthesis increase crop yields? Plant Cell Environ. 29:315–330. 10.1111/j.1365-3040.2005.01493.x
- Meheta P, Jajoo A, Mathur S, Bharati S. 2010. Chlrophyll a fluorescence study revealing effect of high salt stress on photosystem II in wheat leaves. Plant Physiol Biochem. 48:16–20. 10.1016/j.plaphy.2009.10.006
- Moayedi AA, Boyce AN, Barakbah SS. 2009. Influence of water deficit during different growth and developmental stages on the contribution of stored pre-anthesis assimilates to grain in selected durum and bread wheat genotypes. Aus J Basic Appl Sci. 3:4408–4415.
- Mostajeran A, Rahimi-Eichi V. 2009. Effects of drought Stress on growth and yield of rice (Oryza sativa L.) cultivars and accumulation of proline and soluble sugars in sheath and blades of their different ages leaves. American-Eurapian J Agric & Environ Sci. 5:264–272.
- Nagai K, Hattori Y, Ashikari M. 2010. Stunt and elongate? Two opposite strategies by which rice adopts to flood. J Plant Res. 123:303–309. 10.1007/s10265-010-0332-7
- Nagata K. 2006. Ecophysiological traits and genetic analysis of yield and ripening in high-yielding semi-dwarf Indica rice varieties. Jpn Agr Res Q. 40:307–316.
- Panda D, Sharma SG, Sarkar RK. 2008. Chlorophyll fluorescence parameters, CO2 Photosynthetic rate and regeneration capacity as a result of complete submergence and subsequent re-emergence in rice (Oryza sativa L.). Aquatic Bot. 88:127–133. 10.1016/j.aquabot.2007.08.012
- Peleg Z, Reguera M, Tumimbang E, Walia H, Blumwald E. 2011. Cytokinin-mediated source/sink modifications improve drought tolerance and increase grain yield in rice under water-stress. Plant Biotech J. 9:747–758. 10.1111/j.1467-7652.2010.00584.
- Porra RJ. 2002. The chequered history of the development and use of simultaneous equations for accurate determination of chlorophylls a and b. Photosynth Res. 73:149–156. 10.1023/A:1020470224740
- Prasad PVV, Boote KJ, Vu JCV, Allen LH Jr. 2004. The carbohydrate metabolism enzymes sucrose-p synthase and ADG- pyrophosphorylase in phaseolus bean leaves are upregulated at elevated growth carbon dioxide and temperature. Plant Sci. 166:565–1573.
- Racker E. 1962. Fructose 1,6 diphosphate from spinanch leaves. In: Colowisk SP, Kaplan NO, editors. Methods in enzymology. New York and London: Academic Press; p. 37–45.
- Rocher JP, Prioul JL, Lecharny A, Reyss A, Joussaume M. 1989. Genetic adoptability in carbon fixation, sucrose- P synthase and ADP- glucose pyrophosphorylase in maize plants of differing growth rate. Plant Physiol. 89:416–420. 10.1104/pp.89.2.416
- Sadasivum S, Manickum A. 1997. Biochemical methods. New Delhi: New Age International (P) Ltd, p. 121–124.
- Sarkar RK, Panda D, Reddy JN, Patnaik SSC, Mackil DJ, Ismail AM. 2009. Performance of submergence tolerant rice (Oryza sativa) genotypes carrying the Sub1 quantitative trait locus under stressed and non-stressed natural field conditions. Ind J Agric Sci. 79:876–883.
- Sayed OH. 2003. Chlorophyll fluorescence as a tool in cereal crop research. Photosynthetica. 41:321–330. 10.1023/B:PHOT.0000015454.36367.e2
- Strand A, Zrenner R, Trevanion S, Stiff M, Gustafison P. 2000. Decrease expression of two key enzymes in sucrose biosynthetic pathway, cytosolic triose-1, 6-bisphosphatase and sucrose phosphate synthase was remarkably different consequences for photosynthetic carbon metabolism in transgenic Arabidopsis thaliana. The Plant J. 23:759–770. 10.1046/j.1365-313x.2000.00847.x
- Xu K, Xu X, Fukao P, Canlas R, Maghirang-Rodriguez R, Heuer S, Ismail AM, Bailey-Serres J, Ronald PC, Mackill DJ. 2006. Sub1A is an ethylene-response-factor-like gene that confers submergence tolerance. Nature. 442:705–708. 10.1038/nature04920
- Yang J, Zhang J. 2006. Grain filling of cereals under soil drying. New Phytol. 169:223–236. 10.1111/j.1469-8137.2005.01597.x
- Yoshida S, Forno DA, Cock JH, Gomez KA. 1976. Laboratory manual for physiological studies of rice. Philippines: IRRI. p. 14–46.
- Yu-Chun D, Nose A, Kawamitsu Y, Murayama S, Wasano K, Uchida Y. 1996. An improved spectrophotometric determination of the activity of ribulose 1,5,-bisphosphate carboxylase. Jpn J Crop Sci. 65:714–721. 10.1626/jcs.65.714
- Zeeman S, Delatte T, Meeerli G, Umbang M, Sletter M, Mettler T, Streb S, Reinhold H, Kotting O. 2007. Starch breakdown: recent discoveries suggest distinct pathways and novel mechanisms. Fun Plant Biol. 34:465–473. 10.1071/FP06313