Abstract
Arid regions of the Arabian Peninsula are characterized by a short wet season with erratic rainfall, high temperature, and high evaporation. In these arid regions, the leafless stem succulent Euphorbia fractiflexa S. Carter & J.R.I.Wood (Euphorbiaceae) is an abundant perennial. Work presented in this paper aimed at investigating crassulacean acid metabolism (CAM) as a physiological adaptation crucial for survival of E. fractiflexa in arid regions of the Arabian Peninsula. Work involved investigations of stomatal diffusive conductance, chlorophyll fluorescence, and cell sap titratable acidity. Results represent the first report of obligate CAM in E. fractiflexa. Low values of stomatal conductance and dampening of CAM acidification–deacidification cycles during the long dry season also denoted tendency of this species to shift from obligate CAM to CAM-idling. Results also showed water stress-induced reduction in Photosystem II (PSII) activity occurring in concomitance with increased non-photochemical quenching of chlorophyll fluorescence denoting operation of non-photochemical energy dissipation mechanisms that are important for photoprotection of the photosynthetic machinery under stress conditions.
Introduction
Arid regions of the Arabian Peninsula are characterized by high temperature, high evaporation, scarce water, and erratic rainfall (Fisher & Membery Citation1998). In such arid regions, leaf succulents reduce their transpirational surface area by seasonal partial leaf shedding (Sayed Citation1996, Sayed Citation1998, Sayed Citation2001a), whereas leafless stem succulents rely on ample water storage capacity, morphoanatomical traits, and physiological adaptations (Sayed Citation2001a; Masrahi et al. Citation2012a, Citation2012b). These physiological adaptations involve exhibiting crassulacean acid metabolism (CAM) photosynthesis for its unique nocturnal carbon acquisition which improves the plant water economy (Dodd et al. Citation1997; Borland et al. Citation2000; Sayed Citation2001b; Luttge Citation2002; Masrahi et al. Citation2011; Masrahi et al. Citation2012a, Citation2012b). Reports on photosynthesis in the genus Euphorbia from different parts of the world indicated that leaf succulent Euphorbia species exhibited C4 photosynthesis (Pearcy & Troughton Citation1975), whereas leafless stem succulent Euphorbia species were CAM plants (Pearcy & Troughton Citation1975; Webster et al. Citation1975; Ziegler et al. Citation1981; Willert et al. Citation1985; Martin et al. Citation1990; Van Damme Citation1991; Mies et al. Citation1996; Ameh Citation2006; Feakins and Sessions Citation2010). However, work on photosynthesis of the genus Euphorbia in the Middle East is scarce. A report on photosynthesis in the genus Euphorbia in Egypt indicated that all leaf succulent species were C4 plants, whereas leafless stem succulent species were CAM plants (Batanouny et al. Citation1988).
The leafless stem succulent Euphorbia fractiflexa S. Carter & J.R.I.Wood is a common stem succulent in arid regions of the Arabian Peninsula (Collenette Citation1999). Work presented in this paper aimed at investigating the presence of CAM in E. fractiflexa. Investigations involved field studies of seasonal and diurnal changes in stomatal diffusive conductance, and cell sap titratable acidity. Moreover, plants with the CAM pathway exhibit different CAM physiotypes namely; obligate CAM, facultative CAM, CAM-cycling, and CAM-idling (Luttge Citation2004; Herrera Citation2009). Of these modes, CAM-idling has early been recognized (Ting & Sipes Citation1985), and is considered to be a very strong modification of CAM occurring under extreme environmental conditions (Cushman Citation2001; Sayed Citation2001b; Dodd et al. Citation1997). This CAM-idling mode is characterized by stomatal closure during the entire day and night, no net CO2 uptake, and acidification fed by internal recycling of nocturnally re-fixed respiratory CO2 (Luttge Citation2004; Lüttge Citation2008; Herrera Citation2009). Work presented in this paper also aimed at describing possible modifications CAM in E. fractiflexa. Furthermore, during Phase III of CAM occurring under closed stomata, high irradiance, and high temperature, Photosystem II (PSII) becomes over-energized (Niewiadomska & Borland Citation2008) and plants are thought to invoke photoprotection of PSII by excess energy dissipation via non-radiative mechanisms and the xanthophyll cycle (Tallman et al. Citation1997; Tallman Citation2004; Herrera Citation2009). Therefore, our work also involved using pulse amplitude modulated chlorophyll fluorescence to investigate the involvement of CAM-idling in mitigating deleterious effects of water stress on the photosynthetic machinery.
Materials and methods
The study site southwest of Saudi Arabia (17°19′N–42°48′E) is characterized by sand-loam soil, high temperature, high irradiance, scarce water, erratic rainfall, and a climate influenced by tropical maritime air mass (Fisher & Membery Citation1998). Climatic records of mean monthly maximum air temperature, precipitation, and evaporation for the past 40 years (1970–2010) were obtained from the Ministry of Electricity and Water (Riyadh, Saudi Arabia).
Stomatal diffusive conductance was measured in intact stem using a porometer (AP4, Delta-T Devices, Cambridge, UK). Pulse amplitude modulated chlorophyll fluorescence was measured using a chlorophyll fluorescence monitoring system (FMS2, Hansatech Instruments, Norfolk, UK). Measured chlorophyll fluorescence parameters included Fv/Fm, and Φ PSII, reflecting efficiency of PSII antenna and the quantum yield of PSII, respectively (Bolhàr-nordenkampf & Öquist Citation1993; Sayed Citation2003). The value qNP reflecting non-photosynthetic quenching of chlorophyll fluorescence was calculated using standard fluorescence nomenclature (Sayed Citation2003) and Equation (1)
Changes in CAM acidification and deacidification were studied in chlorenchyma cell sap extracted by grinding a known weight of tissue in distilled water, expressing cell sap through two layers of muslin, and determining cell sap titratable acidity (Osmond et al. Citation1991). Studies were conducted in mid-May and Mid-July for the dry and wet season, respectively. All experiments were routinely repeated in samples taken from 10 different individual plants and the standard deviation was calculated.
Results
Climatic records indicated that the study site is characterized by a long dry season and a short wet season (June–August) during which air temperature, precipitation, and evaporation markedly increased (). These climatic data also showed that the mean annual maximum air temperature is 36.7°C and that the total annual precipitation is 93.4 mm.
Table 1. Climatic records at the site for the period 1970–2010.
Measurements of stomatal diffusive conductance during the wet season indicated that E. fractiflexa exhibited high night-time values and low day-time values (). On the other hand, measurements during the dry season indicated that E. fractiflexa exhibited markedly low values of stomatal conductance in the range of 3–5 mmol m−2 s−1 during both night and day (). Moreover, determinations of chlorenchyma cell sap titratable acidity indicated that E. fractiflexa exhibited diurnal acidity changes during the wet season, and that these acidity changes were markedly dampened during the dry season (). Measurement of chlorophyll fluorescence during the dry season showed reduction in the value of the parameters Fv/Fm, and Φ PSII compared to those measured during the wet season (). This reduction of the value of chlorophyll fluorescence parameters during the dry season occurred in concomitance with a marked increase in the value of the parameter qNP ().
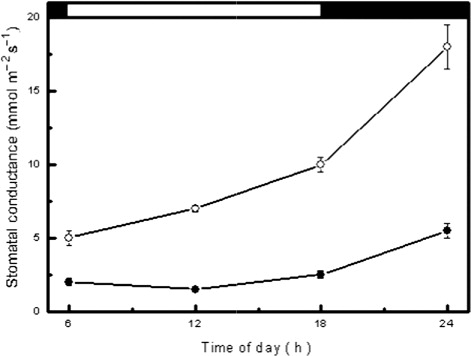
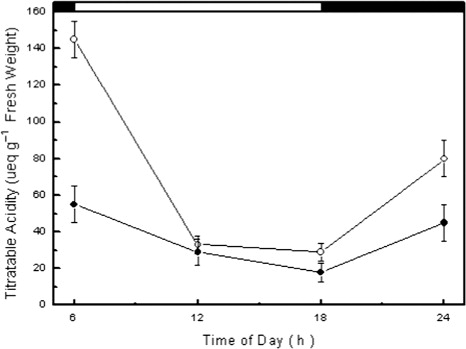
Table 2. Seasonal changes in chlorophyll fluorescence parameters of E. fractiflexa (±SD, n=10).
Discussion
Climatic records reflected the extreme aridity prevailing at the study site. This aridity was manifested by a nine-month-long dry season and a short wet season characterized by water scarcity, high temperature and high evaporation (). In such arid habitats, perennial stem succulents survive periods of protracted drought by morphoanatomical traits and physiological adaptations that enable them to tolerate harsh environmental conditions (Sayed Citation2001a, Citation2001b; Masrahi et al. Citation2011; Masrahi et al. Citation2012a, Citation2012b).
During the wet season, E. fractiflexa exhibited values of stomatal conductance that were high during the night and low during the day (), a stomatal behavior typical of CAM plants (Sayed Citation2001b; Luttge Citation2004; Herrera Citation2009). Determination of chlorenchyma cell sap titratable acidity during both the wet and the dry seasons indicated existence of diurnal acidification–deacidification cycles denoting operation of the obligate CAM. This CAM physiotype has previously been reported in some stem succulent Euphorbia species (Pearcy & Troughton Citation1975; Willert et al. Citation1985; Martin et al. Citation1990; Van Damme Citation1991; Mies et al. Citation1996; Elhaak et al. Citation1997; Winter et al. Citation2005; Ameh Citation2006; Feakins and Sessions Citation2010). Nocturnal CO2 uptake and daytime stomatal closure characteristic of CAM imply avoidance of gas exchange when environmental conditions favor transpirational water loss and hence enhanced plant water economy (Walker & Leegood Citation1996; Winter & Smith Citation1996; Borland et al. Citation2000; Sayed Citation2001b; Luttge Citation2004; Lüttge Citation2008; Herrera Citation2009). However, during the long dry season, E. fractiflexa exhibited very low values of stomatal conductance during both day and night () and markedly dampened diurnal oscillation of cell sap titratable acidity (). These results indicated that E. fractiflexa shifted from the obligate CAM physiotype to a CAM-idling mode in response to protracted drought. The CAM-idling mode is a strong modification of CAM that greatly enhances plant water economy by stomatal closure during the entire day and night and sustaining diurnal fluctuations in cell sap titratable acidity by nocturnally re-fixing respiratory CO2 (Sayed Citation2001b; Dodd et al. Citation1997; Nobel & De La Barrera Citation2002; Luttge Citation2004; Lüttge Citation2007; Herrera Citation2009).
Comparison of chlorophyll fluorescence parameters measured during the wet and the dry seasons in E. fractiflexa indicated reduction of PSII activity manifested by reduction of Fv/Fm and Φ PSII (). Reduction of Fv/Fm and Φ PSII denotes reduction of the efficiency of PSII antenna and PSII quantum yield, respectively (Bushmann Citation1999; Sayed Citation2003). Similar reduction of PSII activity has previously been shown for other CAM plants under protracted drought (Mattos et al. Citation1999) and was attributed to an over-energized PSII during Phase III of CAM that takes place behind closed stomata under high irradiance and high temperature (Mattos et al. Citation1999; Niewiadomska & Borland Citation2008). However, our observed reduction in PSII activity occurred in concomitance with a markedly increased qNP () denoting increased non-photochemical quenching of chlorophyll fluorescence and hence increased non-photochemical excess energy dissipation (Bushmann Citation1999; Sayed Citation2003). Similar increased qNP in Clusia minor performing CAM-idling (Lüttge Citation2007) was attributed to a relationship between zeaxanthin, a precursor of abscisic acid, and the onset of CAM-idling. Moreover, inhibition of zeaxanthin accumulation in guard cells of CAM-performing Mesembryanthemum crystallinum was also suggested to prevent stomatal opening in response to light (Tallman et al. Citation1997). In this case, daytime decarboxylation and high rate of respiration due to high temperature imply high intercellular CO2 concentration that ensures daytime stomatal closure by favoring Calvin cycle activity in guard cell chloroplasts, consumption of NADPH, and prevention of the destruction of endogenous guard cell abscisic acid (Tallman Citation2004). Furthermore, reports the literature suggested that CAM-idling plays a role in photoprotection by non-radiative excess energy dissipation via the xanthophyll cycle (Robinson & Osmond Citation1994; Tallman et al. Citation1997; Tallman Citation2004; Herrera Citation2009). The CAM-idling mode is thought to result in reduction of oxidative stress by processing reactive oxygen species that appear when PSII becomes over-energized during Phase III of CAM and hence confer photoprotection under protracted drought (Lüttge Citation2007; Niewiadomska & Borland Citation2008).
It can, therefore, be concluded that E. fractiflexa is an obligate CAM plant exhibiting stomatal behavior typical of CAM plants during both the wet and the dry seasons. Low stomatal conductance during the entire day and night and dampened acidification–deacidification cycles indicated that E. fractiflexa shifts from the obligate CAM to CAM-idling in response to protracted water stress during the long dry season. Under severe drought, PSII activity in E. fractiflexa was reduced due to oxidative stress during CAM-idling. This oxidative stress appears to be mitigated by operation of photoprotective non-radiative excess energy dissipation as reflected by increased non-photochemical quenching of chlorophyll fluorescence.
References
- Ameh, GI. 2006. Crassulacean acid metabolism in some succulent plants. Plant Prod Res J. 10:16–19.
- Batanouny, KH, Stichler, W, Ziegler, H. 1988. Photosynthetic pathways and ecological distribution of Euphorbia species in Egypt. Oecologia. 87:565–569. 10.1007/BF00320421
- Bolhàr-nordenkampf, HR, Öquist, GO. 1993. Chlorophyll fluorescence as a tool in photosynthesis research. In: Hall DO, Scurlock JMO, Bolhàr-nordenkampf HR, Leegood RC, Long SP, editors. Photosynthesis and production in a changing environment. London: Chapman and Hall; 3–37.
- Borland, AM, Maxwell, K, Griffiths, H. 2000. Ecophysiology of plants with crassulacean acid metabolism. In: Leegood R, Sharkey TD, Von Gaemmerer S, editors. Photosynthesis: physiology and metabolism. Amsterdam: Kluwer Academic; p. 583–605.
- Bushmann, C. 1999. Photochemical and non-photochemical quenching of the chlorophyll fluorescence: comparison of variation and limits. Photosynthetica. 37:217–224. 10.1023/A:1007003921135
- Collenette, S. 1999. Wildflowers of Saudi Arabia. Riyadh: NCWCD.
- Cushman, JC. 2001. Crassulacean acid metabolism. A plastic photosynthetic adaptation to arid environments. Plant Physiol. 127:1439–1448. 10.1104/pp.010818
- Dodd, AN, Borland, AM, Halsam, RP, Griffiths, H, Maxwell, K. 1997. Crassulacean acid metabolism: plastic fantastic. J Exp Bot. 53:569–580. 10.1093/jexbot/53.369.569
- Elhaak, MA, Migahid, MM, Wegmann, K. 1997. Ecophysiological studies on Euphorbia paralias under soil salinity and sea water spray treatments. J Arid Environ. 35:459–471. 10.1006/jare.1995.0151
- Feakins, SJ, Sessions, AL. 2010. Crassulacean acid metabolism influences D/H ratio of leaf wax in succulent plants. Organ Geochem. 41:1269–1276. 10.1016/j.orggeochem.2010.09.007
- Fisher, M, Membery, DA. 1998. Climate. In: Ghazanfar SA, Fisher M, editors. Vegetation of the Arabian Peninsula, p. 5–38. Amsterdam: Kluwer Academic Publishers.
- Herrera, A. 2009. Crassulacean acid metabolism and fitness under water deficit stress: if not for carbon gain, what is facultative CAM good for?. Ann Bot. 103:645–653. 10.1093/aob/mcn145
- Luttge, U. 2002. CO2-concentrating: consequences in crassulacean acid metabolism. J Exp Bot. 53(378), 2131–2142. 10.1093/jxb/erf081
- Luttge, U. 2004. Ecophysiology of crassulacean acid metabolism (CAM). Ann Bot. 93(6), 629–652. 10.1093/aob/mch087
- Lüttge, U. 2007. Clusia: a woody neotropical genus of remarkable plasticity and diversity. Heidelberg: Springer-Verlag.
- Lüttge, U. 2008. Stem CAM in arborescent succulents. Trees. 22(2), 139–148. 10.1007/s00468-007-0198-z
- Martin, CE, Loeschen, VS, Lloyd, LB, Coke, LB. 1990. Crassulacean acid metabolism in selected terrestrial succulents in southeastern Jamaica, including two species in the Commelinaceae. Oecologia. 84:99–102. 10.1007/BF00665601
- Masrahi, YS, Al-Yemeni, MN, Al-Turki, TA, Sayed, OH. 2011. Ecophysiological mechanisms of succulent survival in natural conditions: photosynthetic carbon fixation in Caralluma acutangula (Decne. Ne.Br.) (Asclepiadaceae). Polish J Ecol. 59:381–389.
- Masrahi, YS, Al-Yemeni, MN, Sayed, OH. 2012a. Nurse association of the stem succulent Caralluma acutangula in its natural habitat. Ekologia. 31(1), 46–53. 10.4149/ekol_2012_01_46
- Masrahi, YS, Al-Turki, TA, Sayed, OH. 2012b. Crassulacean acid metabolism permutation and survival of Caralluma Species (Apocynaceae) in arid habitats. Ecol Balkanica. 4:63–71.
- Mattos, EA, Herzog, B, Lüttge, U. 1999. Chlorophyll fluorescence during CAM-phases in Clusia minor L. under drought stress. J Exp Bot. 331:253–261.
- Mies, B, Jiménez, MS, Morales, D. 1996. Ecophysiology and distribution of the endemic leafless spurge Euphorbia aphylla and the introduced E. tirucalli (Euphorbiaceae, Euphorbia sect.Tirucalli) in the Canary Islands. Plant Systemat Evol. 202(1–2), 27–36. 10.1007/BF00985816
- Niewiadomska, E, Borland, AM. 2008. Crassulacean acid metabolism: a cause or consequence of oxidative stress in plants?. Prog Bot. 69:247–266.
- Nobel, PS, De La Barrera, E. 2002. Stem water relations and net CO2 uptake for a hemiepiphytic cactus during short-term drought. J Exp Bot. 48:129–137. 10.1016/S0098-8472(02)00016-3
- Osmond, CB, Adams, WW, Smith, ST. 1991. Crassulacean acid metabolism. In: Pearcy RW, Ehleringer J, Mooney HA, Rundel PW, editors. Plant Physiological Ecology, 255–280. London: Chapman and Hall, London.
- Pearcy, RW, Troughton, J. 1975. C4 photosynthesis in tree form Euphorbia species from Hawaiian rainforest sites. Plant Physiol. 55(6), 1054–1056. 10.1104/pp.55.6.1054
- Robinson, S, Osmond, C. 1994. Internal gradients of chlorophyll and carotenoid pigments in relation to photoprotection in thick leaves of plants with crassulacean acid metabolism. Aust J Plant Physiol. 21:497–506. 10.1071/PP9940497
- Sayed, OH. 1996. Adaptational responses of Zygophyllum qatarense Hadidi to stress conditions in a desert environment. J Arid Environ. 32(4), 445–452. 10.1006/jare.1996.0037
- Sayed, OH. 1998. Phenomorphology and ecophysiology of desert succulents in Eastern Arabia. J Arid Environ. 40(2), 177–189. 10.1006/jare.1998.0434
- Sayed, OH. 2001a. Aridity and plant survival in desert environments. In: I Prakash, editor. Ecology of desert environments. Jodhpur: Scientific Publishers; p. 87–103.
- Sayed, OH. 2001b. Crassulacean acid metabolism 1975–2000: a check list. Photosynthetica. 39(3), 339–352. 10.1023/A:1020292623960
- Sayed, OH. 2003. Chlorophyll fluorescence as a tool in cereal crop research. Review. Photosynthetica. 41(3), 321–330. 10.1023/B:PHOT.0000015454.36367.e2
- Tallman, G. 2004. Are diurnal patterns of stomatal movement the result of alternating metabolism of endogenous guard cell ABA and accumulation of ABA delivered to the apoplast around guard cells by transpiration?. J Exp Bot. 55(405), 1963–1976. 10.1093/jxb/erh212
- Tallman, G, Zhu, J, Mawson, BT, Amodeo, G, Nouhi, Z, Levy, K, Zeiger, E. 1997. Induction of CAM in Mesembryanthemum crystallinum abolishes the stomatal response to blue light and light-dependent zeaxanthin formation in guard cell chloroplasts. Plant Cell Physiol. 38(3), 236–242. 10.1093/oxfordjournals.pcp.a029158
- Ting, IP, Sipes, D. 1985. Metabolic modifications of crassulacean acid metabolism in CAM-idling and CAM-cycling. In: Luden PW, Burris JE, editors. Night fixation and CO2 metabolism; Amsterdam: Elsevier. p. 371–378.
- Van Damme, P. 1991. Plant ecology of the Namib desert. Africa Focus. 7:355–400.
- Walker, RP, Leegood, RC. 1996. Phosphorylation of phosphoenolpyruvate carboxykinase in plants: studies in plants with C4 photosynthesis and Crassulacean acid metabolism and in germinating seeds. Biochem J. 317:653–658.
- Webster, GL, Brown, WV, Smith, BN. 1975. Systematics of photosynthetic carbon fixation pathways in Euphorbia. Taxon. 24(1), 27–33. 10.2307/1218995
- Willert, DJ, Brinckmann, E, Scheitler, B, Eller, BM. 1985. Availability of water controls Crassulacean acid metabolism in succulents of the Richtersveld (Namib Desert, South Africa). Planta. 164(1), 44–55. 10.1007/BF00391024
- Winter, K, Smith, JAC. 1996. An introduction to crassulacean acid metabolism. Biochemical principles and ecological diversity. In: Winter K, Smith JAC, editors. Crassulacean acid metabolism. Biochemistry, ecophysiology and evolution. Berlin: Springer Verlag; p. 1–13.
- Winter, K, Aranda, J, Holtum, JAM. 2005. Carbon isotope composition and water-use efficiency in plants with crassulacean acid metabolism. Funct Plant Biol. 32:381–388. 10.1071/FP04123
- Ziegler, H, Batanouny, KH, Sankhla, N, Vyas, OP, Stichler, W. 1981. The photosynthetic pathway types of some desert plants from India, Saudi Arabia, Egypt, and Iraq. Oecologia. 48:93–99. 10.1007/BF00346993