Abstract
Stevia rebaudiana Bertoni is a unique medicinal plant which is mostly utilized as a sugar substitute for diabetic patients. In this research, regenerated plantlets of stevia in tissue culture is transferred to pots in greenhouse and inoculated with plant-growth-promoting rhizobacteria (PGPRs) (Bacillus polymixa, Pseudomonas putida, and Azotobacter chroococcum) and arbuscular mycorrhizal fungus (AMF) (Glomus intraradices). The results showed that in comparison to control, inoculation with a single microorganism, significantly increased root and shoot biomass as well as stevioside, chlorophyll, and NPK content in plants. However, such increased effects have been found to be further enhanced significantly due to dual compatible mixtures of inoculants resulting from their strong synergistic relationships among themselves. All growth parameters recorded the highest in 60-days-old plants in the treatment of Glomus+Azotobacter and followed with Glomus+Bacillus and Azotobacter+Pseudomonas treatments, respectively. Furthermore, high-performance liquid chromatography (HPLC) chromatograms revealed that the highest stevioside content have been produced in same treatments. Triple treatments had less positive effects compared to dual inoculations. Probably competence between microorganisms in triple inoculations has reduced their efficiency. Thus, suitable combination of mycorrhizal fungi and PGPR as biotic elicitors can enhance growth and stevioside content in tissue culture-regenerated plantlets of stevia.
Keywords:
Introduction
Stevia rebaudiana Bertoni belongs to Asteraceae family. The plant is native to certain regions of South America such as Paraguay and Brazil (Sivaram & Mukundan Citation2003). The leaves of Stevia are the source of diterpene glycosides such as stevioside and rebaudioside (Ahmed & Salahin Citation2007). Stevioside, the main sweet component in the leaves of S. rebaudiana, tastes about 300 times sweeter than sucrose (0.4% solution) (Geuns Citation2003). Thus, it is considered to be a sugar substitute and commercial sweetener both in the form of stevioside and stevia extract. In addition to their sweetness, stevia extract and stevioside have been used as a traditional medicine by local people in South America for hundreds of years (Chatsudthipong & Muanprasat Citation2009). Hence, there is a need to enhance biomass through cultural techniques, application of manures, and fertilizers, including biofertilizers.
It has been demonstrated that chemical fertilizer has increased crop yield, but has also caused deleterious effects such as soil acidification and production of the greenhouse gas nitrous oxide (N2O) through denitrification on ecosystems (Biswas et al. Citation2000). One potential way to decrease negative environmental impacts resulting from continued use of chemical fertilizers is inoculation with biofertilizers such as plant-growth-promoting rhizobacteria (PGPR) and arbuscular mycorrhizal (AM) fungi (Adesemoye et al. Citation2009).
The PGPR are a group of rhizosphere colonizing bacteria that produces substances to increase the growth of plants and protect them against diseases (Miransari Citation2011). The enhancement in various agronomic yields by PGPR was because of the production of growth-promoting phytohormones, phosphate mobilization, production of siderophores and antibiotics, inhibition of plant ethylene synthesis, and induction of plant systemic resistances to pathogens. Among PGPR, N2-fixing and P-solubilizing bacteria (PSB) are important for crop plants as they increase nitrogen (N) and phosphorus (P) uptake and play a crucial role as PGPR in the biofertilization (Singh et al. Citation2011).
In addition, inoculation of plants with AM fungi resulted in enhancing plant water and nutrient uptake (Gosling et al. Citation2006). The most important benefit of AM fungi is the ability to scavenge the available P through their hyphae that have large surface areas on which the extraradical hyphae act as a bridge between the soil and plant roots (Liu et al. Citation2000; Bianciotto & Bonfante Citation2002). Also, the use of AM fungi often results in better plant growth (Wu et al. Citation2005) and improves the productivity of medicinal compounds such as organosulfides, polyphenols, phytosterols, stilbenes, vitamins, lignans, and terpenoids including carotenoids which can be important for plant tolerance to abiotic and biotic stresses or beneficial to human health through their antioxidant activities (Gianinazzi et al. Citation2008, Citation2010). These fungi can also induce plant systemic resistance against root pathogens (Gosling et al. Citation2006). AM fungi impart other benefits to plants, including enhanced enzymatic production (Adriano-Anaya et al. Citation2006), increased rate of photosynthesis (Wu & Xia Citation2006), enhancement of nitrogen fixation by symbiotic or associative N-fixing bacteria (Javaid et al. Citation1994; Antunes et al. Citation2006), and improving soil fertility (Charles et al. Citation2006).
Although AM fungi are important symbionts to plants, and their symbiosis can significantly enhance the growth of the host plant, they are also interactive with different soil bacteria. AM hyphae are able to produce C products in a little amount as a source of energy for soil microbes in the mycorrhizosphere. Bacterial communities are able to promote germination of AM fungal spores and increase the rate and extent of root plant colonization by AM fungi. Also, soil microbes produce plant hormones, which can influence AM establishment as well as spore and hyphal growth (Miransari Citation2011).
The major objective of this greenhouse experiment was to evaluate the effect of single, dual, or triple inoculation of Azotobacter chroococcum, Pseudomonas putida, Bacillus polymixa, and AM fungus (Glomus intraradices) as biofertilizers on the promotion of plant growth, stevioside, NPK, and chlorophyll content of 60-days-old stevia plants.
Materials and methods
Stevia plantlet preparation
The seeds of stevia show a very low germination percentage and genetic variability. Vegetative propagation is too limited by lower number of individuals that can be obtained from a single plant and having the possibilities of pathogen accumulation in the tissues. So plant tissue culture technology is the only alternative method for rapid propagation of S. rebaudiana plants (Debnath Citation2008).
Therefore, complete plantlets of S. rebaudiana were obtained by direct organogenesis from nodal segments (1.0–1.2 cm) on Murashige and Skoog medium (Citation1962), containing 3% (w/v) sucrose, 0.6% (w/v) agar, and 0.4% (w/v) activated charcoal. In stevia medium, 0.5 (mg/l) IBA and 1(mg/l) BAP were used as growth regulators. All media were adjusted to pH 5.7 with 1 N NaOH or 1 N HCl and autoclaved at 121°C for 20 min. All cultures were incubated in a growth chamber under the controlled photoperiod of 16 h light and 8 h dark cycle with a light intensity of 3000–4000 lux provided by white fluorescent lamps and 25±2°C temperature condition.
For acclimatization, 4-week-old rooted plantlets were transplanted from the agar medium to plastic bags (10×5 cm) containing sterilized peat moss. The plastic bags were covered with clear plastic bags (for maintenance of humidity) containing pores (for aeration) and lied in a phytotron under the same controlled photoperiod and temperature condition, as mentioned above, for nodal segment culture stage. Covers were removed after 2 weeks. In general, the hardened plantlets were allowed to remain in the plastic bags for 3–4 weeks before being transferred to pots.
Inoculum preparation
In this pot experiment, N-fixer (A.chroococcum strain PTCC 1658), P solubilizer (Pseudomonas putida strain recorded in NCBI (accession number JX 44133)), N-fixer and P solubilizer (Bacillus polymixa strain NCIB 8527), and AM fungus (Glomus intraradices) were used as biofertilizers. Strains of A.chroococcum PTCC 1658 and Bacillus polymixa NCIB 8527 were purchased from Iranian bacteria and fungi collection (Persian Type Culture Collection). Strain of Pseudomonas putida recorded in NCBI (accession number JX 44133) was kindly received from Professor Gity Emtiazi's bacterial collection. The AM fungus (G. intraradices) was purchased from the organic medical plant clinic, Hamedan, Iran. B. polymixa and P. putida were cultured in Luria Broth medium, and they were kept for 24 h in a shaking incubator under 28±1°C and 150 rpm. A.chroococcum was cultured in Burk's N-free liquid medium (Wilson & Knight Citation1952) and was kept for 24 h in a shaking incubator under 28±1°C and 150 rpm. In the treatments containing bacterium, 3×108 colony forming units (CFU)/ml bacterial concentrations were used, and the AM fungus inoculum used in the present experiment consisted of 250 g sandy loam soil containing six spores of AMF and colonized roots of legume plants.
Plant inoculation
Treatments used in this study were T1: control (treated by liquid media applied for bacteria growth without bacteria and sandy loam soil applied for AMF without AMF) and; T2: A. chroococum; T3: B. polymixa; T4: P. putida; T5: G. intraradices; T6: A. chroococcum+G. intraradices; T7: P. putida+G. intraradices; T8: B. polymixa+G. intraradices; T9: A. chroococcum+B. polymixa; T10: A. chroococcum+P. putida; T 11 : A. chroococcum+B. polymixa+G. intraradices; T 12 : A. chroococcum+P. putida+G. intraradices. The pots were filled with sterilized soil and watered a day prior to inoculation of bacteria.
Mycorrhizal treatments were inoculated with 250 g (per pot) soil inoculated with G. intraradices, by placing a thin layer of mycorrhizal inoculum around the roots of plantlets below the surface of the soil when plantlets were planted. For inoculation of PGPR, the roots were treated with 5 ml suspension inoculum (3×108 CFU/ml) of each bacterium. After 2 weeks, plantlets were reinoculated with 5 ml of the same cell suspension on the soil of roots planting in the pots. The pots were maintained at a temperature range of 28±5°C, 50% humidity, and 16 h of light, alternating with 8 h of darkness condition, and plants were watered three times a week, at 100% water-holding capacity of the pots (predetermined).
Plant growth measurement
The plants were harvested 60 days after transplanting. Growth parameters such as plant height, fresh and dry weight were recorded after harvest. Shoots were separated from roots at 0.5 cm above the soil surface, and the length of shoots was recorded. Roots were separated from the soil by washing with running tap water. Fresh weight of shoots and roots were measured immediately, and dry weight was measured by drying the samples in an oven at 40°C for 5 days.
NPK analysis
Total N in shoots was determined calorimetrically after Kjeldahl digestion of ground and dried samples (Anonymous Citation1980). Phosphorus content of the plants was estimated by vanadomolybdate phosphoric acid method (Jackson Citation1973), and available K was determined by atomic absorption spectrometer.
Chlorophyll content analysis
Chlorophyll content also measured in an 80% acetone extract of leaves at 60 days after transplanting by Arnon method (Citation1949) according to following equations:
In the equations V is the volume of solutions, W is the weight of leaf samples, D 663 and D 645 are the solution absorbance at 663 and 645 nm, respectively.
Stevioside analysis
For sample preparation, 100 mg powdered leaf samples were extracted by the modified method of Bovanova et al. (Citation1988). Stevioside extracted in 3 ml distilled water, using a shaking hot-water bath for 30 min at 80°C and 100 rpm. Then, they were centrifuged under 10,000 rpm for 5 min. The volume of extracts was adjusted to 10 ml with distilled water. All solvents of samples were filtered through a 0.45 µm syringe filter (Biofil) and passed through the SPE cartridges (C18, 3 ml) (Cronus) that were conditioned with 3 ml methanol and 3 ml deionized water. The samples (0.5 ml) were loaded. Then, they were washed with 3 ml deionized water, followed with 3 ml acetonitrile: water (20:80). Stevioside was eluted with 1 ml acetonitrile: water (80:20).
Concentration of stevioside in the extractions of samples was determined by high-performance liquid chromatography (HPLC). The standard curve was obtained after injection of three different concentration of stevioside standard (Sigma). The chromatographic separations were carried out by using NH2 column (Merck). Operating conditions for HPLC were as follows: the mobile phase, gradient acetonitrile: deionized water (80:20), a flow rate of the mobile phase of 1.2 ml/min, and UV detection at 210 nm (Kolb et al. Citation2001; Woelwer-Rieck et al. Citation2010).
Experimental design and statistical analysis
The experiment was carried out in a completely randomized design. The results were statistically analyzed by using the SAS software (version 8), and the means were separated by Duncan's multiple range test at 5% using generalized linear models (GLM).
Results
The effects of single or coinoculation with A. chroococcum, B. polymixa, P. putida, and G. intraradices on the growth parameters of 60-days-old plants are shown in . All four microorganisms showed stimulatory effect, in terms of plant height, root length, fresh and dry weight of shoots and roots when inoculated separately. Among the single treatments, the maximal increase for majority of the growth parameters (root length, fresh and dry weight of shoots and roots) was shown by G. intraradices plants in comparison to uninoculated plants. In contrast, dual inoculation significantly enhanced the plant height, root length, fresh and dry weight of shoots and roots. Despite the enhanced growth parameters of shoots and roots by biofertilizers, the stimulatory effect of biofertilizers (in particular, dual inoculation) on root dry weight was higher than shoot dry weight. Inoculation with triple microorganisms had less positive effects on plant growth of stevia in comparison to dual inoculations ().
Table 1. Effect of biofertilizer inoculation on plant growth after 60 days of growth.A
The maximum increase in shoot fresh weight (53.7%), dry weight (57%), and height (37%) was obtained in A. chroococcum+G. intraradices treatment. Furthermore, maximum root length was obtained in dual inoculations of A. chroococcum+G. intraradices by 21.54%. A. chroococcum+G. intraradices produced almost 2.52 and 2.9 times more fresh and dry root matter relative to the noninoculated plants. Dual symbiotic plants were approximately twofold larger than nonsymbiotic plants, and the shoot growth of the dual symbiotic plants was increased approximately 2.5 and 5-fold compared to single inoculated or uninoculated plants, respectively. Although slightly less pronounced, a similar pattern was observed for root growth with the same treatments ().
The effects of single or coinoculation with A. chroococcum, B. polymixa, P. putida, and G. intraradices on the chlorophyll content of 60-days-old plants are shown in . The maximum chlorophyll a content was recorded in the dual symbiotic plants with A. chroococcum+G. intraradices, B. polymixa+G. intraradices, and A. chroococcum+P. putida by 3.8, 3.5, and 3.3 times compared to the controls. The highest chlorophyll b content was found in treated plants with A. chroococcum+G. intraradices, A. chroococcum+P. putida and A. chroococcum+B. polymixa by increasing 3.2, 2.6, and 2.5 times compared to the uninoculated plants. Results also showed that highest total chlorophyll was found in treated plants with A. chroococcum+G. intraradices, A. chroococcum+P. putida and B. polymixa+G. intraradices by 3.5, 3, and 2.8 times compared to the controls. Among the single inoculations, A. chroococcum showed maximum chlorophyll a, b, and total content, and the uninoculated plants displayed the lowest chlorophyll a, b and total content. Moreover, the results showed that triple inoculations had more positive effects on chlorophyll a, b, and total content than had the single inoculation, but their effects were less than those of dual inoculations ().
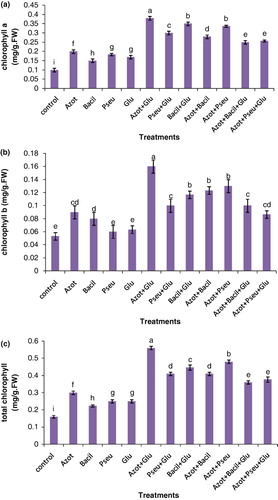
Single inoculation treatments, in general, increased the N content relative to the control (). Among the single inoculation, A. chroococcum increased the N content significantly by 28% relative to the control. Results also showed that dual inoculations significantly stimulated the N content in plants compared with the control. Among the dual inoculation treatments, coinoculation of A. chroococcum+G. intraradices, A. chroococcum+P. putida showed the highest positive effect on N content and increased the N content by 49 and 39%, respectively, in comparison to control ().
Table 2. Effect of biofertilizer inoculation on NPK and stevioside after 60 days of growth.A
The results also showed P content in plants significantly increased with the inoculation of AM fungi alone or in combination with PGPR (). Among the single inoculation treatments, P-solubilizing bacteria and AM fungi significantly increased P content compared to the control. However, the effect of P-solubilizing bacteria on P content had been less than AM fungi (). The P content was significantly enhanced in inoculated plants with A. chroococcum+G. intraradices, B. polymixa+G. intraradices, and P. putida+G. intraradices by 3.3, 3.09, and 3.05 times in comparison to the control ().
Yet once again, the dual symbiotic plants had higher K content than any of the other treatments (). The present results also showed that among all dually inoculated plants, plants inoculated with AM fungi and PGPR had maximum K content. In plantlets inoculated with B. polymixa+G. intraradices, A. chroococcum+G. intraradices, and P. putida+G. intraradices K content increased 62%, 60%, and 58% in comparison to noninoculated plants ().
The triple inoculation of A. chroococcum+B. polymixa+G. intraradices, and A. chroococcum+P. putida+G. intraradices significantly improved the NPK content compared to the control. The lowest N, P, and K uptake was detected in plants grown in uninoculated pots ().
The results also showed that among the single microbial inoculums, the AM fungi were better at improving stevioside content (). Among all inoculation treatments, dual inoculation of plants had the highest positive effect on stevioside. Coinoculation of A. chroococcum with G. intraradices increased stevioside by 83% in comparison with the control. The results showed inoculated plantlets with B. polymixa+G. intraradices also significantly increased stevioside by 53% compared to the control. Triple inoculation had less positive effects on stevioside compared to dual inoculations ().
Discussion
The synergistic benefits of the dual inoculation of stevia obviously improved the relative growth, biomass, and nutrition assimilation of the host plants ( and ). The improved growth of the dual symbiotic plants is in agreement with the previous study carried out by Hemavathi et al. (Citation2006), who reported improved growth and biomass yield of Ocimum basilicum that was inoculated with G. fasciculatum and PGPR. Vasanthakumar (Citation2003) also reported that combined inoculation of Azospirillum (AZUS10) and PSB isolate (PSB7) produced synergistic effect, resulting in increased root length, shoot length, stem girth, number of leaves, and number of branches in solanaceous crop plants. This improved growth is most likely a result of improved nutrition, leading to higher photosynthetic rates.
The increasing of chlorophyll in the dual symbiotic plants () probably resulted in higher photosynthetic rates and thus improved plant biomass. It has also been shown in the study of Aseri et al. (Citation2008) who reported that the highest total chlorophyll was observed in dual inoculated plants of Punica granatum by G. mosseae and A. brasilense after 4 months. The increasing of chlorophyll that could lead to higher rates of photosynthesis is dependent on two factors: first, a greater C sink below ground due to the two symbionts and second, by the improved nutrition of the host plants. Interestingly, despite the below-ground C sink, more C was invested into the above-ground tissue of the dual symbiotic plants in comparison to the other treatments. This was, likely, the result of cost-benefit effects between the C and nutrient sinks. To begin with, the higher demand for below-ground C resulted from having to support both symbionts may have caused an increase in the photosynthetic rates of these plants, as shown in the work of Kaschuk et al. (Citation2009). Additional evidence in favor of the argument regarding the C and nutrient sinks resulting in higher above-ground C investment is that the dual symbiotic plants were dramatically more efficient in terms of NPK capture than any of the other treatments; thus, allowing more C to be available for growth in place of nutrient assimilation. The high shoot NPK concentrations would allow for increased shoot growth and photosynthetic rates. The occurrence of increased above-ground growth was also reported by Singh et al. (Citation2012), who found that Coleus forskohlii plants colonized by both Pseudomonas monteilii and Glomus fasciculatum had higher N, P, and K uptake and greater above-ground biomass. Aseri et al. (Citation2008) assessed the effectiveness of PGPR (A.chroococcum and A. brasilense) and AM fungi (G. mosseae and G. fasciculatum) on the growth, nutrient uptake, and biomass production of pomegranate (Punica granatum L.) in the field experiments in India. Results showed that dual inoculation of PGPR and AM fungi led to higher biomass production and increase in the uptake of N as well as P, K, Ca, and Mg in pomegranate seedling. Aseri et al. (Citation2008) attributed this to the increased N, P levels, resulting from improved biological N fixation (BNF) and phosphatase activity. Das et al. (Citation2007) conducted a greenhouse study to evaluate the effects of three biofertilizers consisting of AM fungi, PSB, and Azospirillum on the growth of S. rebaudiana. They reported that AM fungi and PGPR inoculants increased the growth, nutritional assimilation, and microelements of stevia due to their ability to fix atmospheric nitrogen and transform native soil nutrients such as P, Zn, Cu, Fe, and sulfur from nonuasble (fixed) to soluble form. On the other hand, it is suggested that production of phytohormones by PGPR led to improved root and hairy roots growth. Thus, the levels of NPK uptake were increased from soil (Wu et al. Citation2005).
The AM-inoculated plants had dramatically increased levels of P in plants especially in comparison with the non-AM plants. It is also shown that the AM fungi plays an important role in both indirect and direct N nutrition of host plants. The high levels of N and K were found in single AM fungi inoculated plants, compared to the uninoculated plants. It indicated the direct influence of AM fungi on the levels of N in host plants. This is in line with previous studies showing that AM fungi are able to directly assimilate and provide N and K to the host plants (Toussaint et al. Citation2004; Mortimer et al. Citation2009).
In the present study, the high level of N concentrations was found in the dual inoculated plant by A. chroococcum with G. intraradices plants. It indicated the indirect role of AM fungi in the N nutrition of the host plants, by enhancing biological N fixation (BNF). Mortimer et al. (Citation2009) illustrated that in tripartite symbiosis between legumes, AM fungi, and nodule bacteria, the presence of AM fungi led to increased rate of BNF and plant N concentrations. These findings suggest that AM plays an important role both directly through enhancing nutrition and indirectly through enhancing synergistic benefits of the dual colonization in order to increase the ability of stevia to become established in soils low in N and P. The increased levels of P in roots in dual inoculation would ensure continued nitrogen fixation by Azotobacter, thus providing additional N to be used in the photosynthetic process of the host plants. This is evident from the higher transport of N to the shoots and subsequent increase in above-ground biomass, as discussed earlier.
In the present study, stevioside was also significantly increased in inoculated plants especially by exposure to A. chroococcum+G. intraradices (). Steviol glycosides are diterpenoids whose biosynthetic pathways share four steps in common with gibberellic acid formation (Brandle and Telmer Citation2007). Awasthi et al. (Citation2011) reported that while individual inoculation with an AM fungus (G. mosseae) or Bacillus subtilis was not effective in increasing artemisinin content, dual-inoculation (G. mosseae+B. subtilis) significantly increased the content of artemisinin. Banchio et al. (Citation2010) reported that biosynthesis of terpenoids depends on primary metabolism, photosynthesis, and oxidative pathways for carbon and energy supply. Both AM fungi and PGPR are capable of increasing the primary metabolites by improving the photosynthesis, mineral content, and growth of plants. They suggested that the promoting effect of biofertilizers on secondary metabolites production may be due to the good balance of nutrients and water in the root zone. Besides, we believe that the observed increases in the synthesis of stevioside, which is a secondary metabolite, may be related to a production of elicitors by AM fungi and PGPR. Maybe releasing of hormones and other biologically active molecules by microorganisms in soil acts as elicitors which stimulated stevioside production. Recently, the effect of rhizobacterial volatile organic compounds (VOCs) on the formation of plant secondary compounds in Mentha piperita has indicated an almost twofold increase in accumulation of essential oils in plants treated with P. fluorescens as compared to B. subtilis, A. brasilensis, or control (Santoro et al. Citation2011).
The physiological advantages associated with the triple inoculation of stevia were less than dual inoculations. It may be related to the fact that a triple symbiosis would involve a larger C sink burden than either symbionts on its own. Therefore, by limiting symbiotic development of both AM fungus and PGPR, the amount of C used by the respective symbionts can be regulated. In support of this fact, Pearson et al. (Citation1993) found that competition for root C could limit the extent of AM colonization. On the other hand, it is possible that reduction effect of triple inoculation, due to the release of inhibitory metabolites in the growing environment, in turn adversely affected the plant growth or other microorganisms. These hypotheses must be investigated further in future. However, these findings are contrary to other studies showing improved growth and development of plants under triple inoculations. Zaidi et al. (Citation2003) reported that in low P soils, the highest plant growth and nutrient uptake in chickpea were obtained after inoculation with tripartite culture of Mesorhizobium, PSB, and G. fasciculatum. Das and Dang (Citation2010) also reported that the highest increase of biomass and stevioside were recorded in combined application of AMF+PSB+Azospirillum in acidic soils. The difference in the results of these authors with our data may be due to difference in type, concentration and combination of applied microorganisms or different conditions of experiment performance.
Our results demonstrate that using an appropriate combination of mycorrhizal fungi and PGPR can significantly increase the production of leaf dry mass and concentration of stevioside. The application of dual inoculation with AM fungi and N-fixing bacteria seems to be the most effective treatment combination to improve plant growth, plant nutrient uptake, total chlorophyll, and stevioside content. In fact, dual root colonization usually increases the growth of host over-and-above that of single inoculation. One reason for this response is the improvement of the nutritional balance of hosts, leading to increase chlorophyll content and maintain a high rate of photosynthesis, which compensates the loss of carbon by a large sink of both symbionts. In addition, G. intraradices and A. chroococcum consortium seems to provide the most optimal mix of macronutrients for plants which in turn reflects the maximum stevioside content as well as yield compared to that obtained with the other bioinoculants tested (single, triple, or other dual combination). It is also known that P availability in soils is important for the uptake of soil nitrogen and its utilization in plants. Therefore, more available P due to its solubilization by inoculated AM fungus or PSB could lead to improved N uptake or nitrogen fixation by Azotobacter. These aspects have caused increased dry matter and stevioside content of S. rebaudiana.
Despite attempts to produce stevioside in heterologous systems till date, the S. rebaudiana is the only source of commercial stevioside in the world, and global attempts to increase the stevioside content of this plant, reduce its cost of production. To this end, the use of microbial consortia as G. intraradices and A. chroococcum is precisely aimed in this direction. In addition, it demonstrates the possibility of replacing chemical fertilizers with biofertilizers, which in turn will help to mitigate the major global problem of environmental pollution and also fetch a premium in the agriculture market.
Acknowledgments
We thank Prof. Emtiazi for the strain of Pseudomonas putida (accession number JX 44133). The authors are grateful to Dr. Abdol Reza Nabinejad for his kind cooperation and help in providing laboratory facilities for this research, and Dr. Javad Hashemi for HPLC analysis, and Prof. Asghari for his guidance. The work was supported by the research assistance of Shahrekord University and the Agricultural Biotechnology Research Institute, Central region of Iran (ABRICI), Isfahan, Iran.
References
- Adesemoye AO, Torbert HA, Kloepper JW. 2009. Plant growth-promoting rhizobacteria allow reduced application rates of chemical fertilizers. Microb Ecol. 58:921–929. doi: 10.1007/s00248-009-9531-y
- Adriano-Anaya ML, Salvador-Figueroa M, Ocampo JA, Garcia-Romera I. 2006. Hydrolytic enzyme activities in maize (Zea mays) and sorghum (Sorghum bicolor) roots inoculated with Gluconacetobacter diazotrophicus and Glomus intraradices. Soil Biol Biochem. 38:879–886. doi: 10.1016/j.soilbio.2005.08.004
- Ahmed MB, Salahin M. 2007. An efficient method for in vitro clonal propagation of a newly introduced sweetener plant Stevia rebaudianain in Bangladesh. Am J Sci Res. 2:121–125.
- Anonymous. 1980. Official methods of analysis. 13th ed. Washington, DC: The Association of Official Analytical Chemists.
- Antunes PM, Varennes Ade, Zhang T, Goss MJ. 2006. The tripartite symbiosis formed by indigenous arbuscular mycorrhizal fungi, Bradyrhizobium japonicum and soybean under field conditions. J Agron Crop Sci. 19:373–378. doi: 10.1111/j.1439-037X.2006.00223.x
- Arnon DI. 1949. Copper enzymes in isolated chloroplast: polyphenol oxidase in Beta vulgaris. Plant Physiol. 24:1–15. doi: 10.1104/pp.24.1.1
- Aseri GK, Jain N, Panwar J, Rao AV, Meghwal PR. 2008. Biofertilizers improve plant growth, fruit yield, nutrition, metabolism and rhizosphere enzyme activities of Pomegranate (Punica granatum L.) in Indian Thar desert. Scientia Hort. 117:130–135. doi: 10.1016/j.scienta.2008.03.014
- Awasthi A, Bharti N, Nair P, Singh R, Shukla AK, Gupta MM, Darokar MP, Kalra A. 2011. Synergistic effect of Glomus mosseae and nitrogen fixing Bacillus subtilis strain Daz26 on artemisinin content in Artemisia annua L. Appl Soil Ecol. 49:125–130. doi: 10.1016/j.apsoil.2011.06.005
- Banchio E, Bogino PC, Santoro M, Torres L, Zygadlo J, Giordano W. 2010. Systemic induction of monoterpene biosynthesis in Origanum×majoricum by soil bacteria. J Agric Food Chem. 58:650–654. doi: 10.1021/jf9030629
- Bianciotto V, Bonfante P. 2002. Arbuscular mycorrhizal fungi: a specialized niche for rhizospheric and endocellular bacteria. Anton van Leeuwenhoek. 81:365–371. doi: 10.1023/A:1020544919072
- Biswas JC, Ladha JK, Dazzo FB, Yanni YG, Rolfe BG. 2000. Rhizobial inoculation influences seedling vigour and yield of rice. Agron J. 92:880–886. doi: 10.2134/agronj2000.925880x
- Bovanova L, Brandsteterova E, Baxa S. 1988. HPLC determination of stevioside in plant material and food samples. Z Lebensm Unters Forsch A. 207:352–355.
- Brandle JE, Telmer PG. 2007. Steviolglycoside biosynthesis. Phytochemistry 68:1855–1863. doi: 10.1016/j.phytochem.2007.02.010
- Charles P, Raj ADS, Kiruba S. 2006. Arbuscular mycorrhizal fungi in the reclamation and restoration of soil fertility. Mycorrhiza News. 18:13–14.
- Chatsudthipong V, Muanprasat C. 2009. Stevioside and related compounds: therapeutic benefits beyond sweetness. Pharmacol Ther. 121:41–54. doi: 10.1016/j.pharmthera.2008.09.007
- Das K, Dang R. 2010. Influence of biofertilizers on stevioside content in Stevia rebaudiana grown in acidic soil condition. Archiv Appl Sci Res. 2:44–49.
- Das K, Dang R, Shivananda T, Sekeroglu N. 2007. Influence of bio-fertilizers on the biomass yield and nutrient content in Stevia rebaudiana Bert. grown in Indian subtropics. J Med Plants Res. 1:005–008.
- Debnath M. 2008. Clonal propagation and antimicrobial activity of an endemic medicinal plant Stevia rebaudiana. J Med Plants Res. 2:045–051.
- Geuns JMC. 2003. Molecules of interest stevioside. Phytochemistry 64:913–921. doi: 10.1016/S0031-9422(03)00426-6
- Gianinazzi S, Gollotte A, Binet MN, Van Tuinen D, Redecker D, Wipf D. 2010. Agroecology: the key role of arbuscular mycorrhizas in ecosystem services. Mycorrhiza. 20:519–530. doi: 10.1007/s00572-010-0333-3
- Gianinazzi S, Huchette O, Gianinazzi-Pearson V. 2008. New outlooks in mycorrhiza applications. In: Baar J, Estaun V, Ortas I, Orfanoudakis M, Alifragis D, editors. Proceedings of the COST870meeting “Mycorrhiza Application in Sustainable Agriculture and Natural Systems”; 2008 Sept 17–19; Thessaloniki, Greece, p. 20–22.
- Gosling P, Hodge A, Goodlass G, Bending GD. 2006. Arbuscular mycorrhizal fungi and organic farming. Agric Ecosystems Environ. 113:17–35. doi: 10.1016/j.agee.2005.09.009
- Hemavathi VN, Sivakumar BS, Suresh CK, Earanna N. 2006. Effect of Glomus fasciculatum and plant growth promoting rhizobacteria on growth and yield of Ocimum basilicum. Karnataka J Agric Sci. 19:17–20.
- Jackson ML. 1973. Soil chemical analysis. New Delhi: Prentice Hall, p. 239–241.
- Javaid A, Iqbal SH, Hafeez FY. 1994. Effect of different strains of Bradyrhizobium and two types of vesicular arbuscular mycorrhizae (VAM) on biomass and nitrogen fixation in Vigna radiata (L.) Wilczek var. NM 20–21. Sci Inter (Lahore), 6:265–267.
- Kaschuk G, Kuyper TW, Leffelaar PA, Hungaria M, Giller KE. 2009. Are the rates of photosynthesis stimulated by the carbon sink strength of rhizobial and arbuscular mycorrhizal symbioses? Soil Biol Biochem. 41:1233–1244. doi: 10.1016/j.soilbio.2009.03.005
- Kolb NJ, Herrera L, Ferreyra DJ, Uliana RF. 2001. Improved HPLC method. J Agric Food Chem. 49:4538–4541. doi: 10.1021/jf010475p
- Liu A, Hamel C, Hamilton RI, Ma BL, Smith DL. 2000. Acquisition of Cu, Zn, Mn, and Fe by mycorrhizal maize (Zea mays L.) grown in soil at different P and micronutrient levels. Mycorrhiza. 9:331–336. doi: 10.1007/s005720050277
- Miransari M. 2011. Interactions between arbuscular mycorrhizal fungi and soil bacteria. Appl Microbiol Biotechnol. 89:917–930. doi: 10.1007/s00253-010-3004-6
- Mortimer PE, Pérez-Fernández MA, Valentine AJ. 2009. Arbuscular mycorrhiza affects the N and C economy of nodulated Phaseolus vulgaris (L.) during NH4+ nutrition. Soil Biol Biochem. 41:2115–2121. doi: 10.1016/j.soilbio.2009.07.021
- Murashige T, Skoog F. 1962. A revised medium for rapid growth and bioassays with tobacco tissue cultures. Physiol Plantarum. 15:473–497. doi: 10.1111/j.1399-3054.1962.tb08052.x
- Pearson JN, Abbott LK, Jasper DA. 1993. Mediation of competition between two colonizing VA mycorrhizal fungi by the host plant. New Phytol. 123:93–98. doi: 10.1111/j.1469-8137.1993.tb04534.x
- Santoro MV, Zygadio J, Giordano W, Banchio E. 2011. Volatile organic compounds from rhizobacteria increase biosynthesis of essential oils and growth parameters in peppermint (Mentha piperita). Plant Physiol Biochem. 49:1177–1182. doi: 10.1016/j.plaphy.2011.07.016
- Singh JS, Pandey VC, Singh DP. 2011. Efficient soil microorganisms: a new dimension for sustainable agriculture and environmental development. Agric Ecosystems Environ. 140:339–353. doi: 10.1016/j.agee.2011.01.017
- Singh R, Soni SK, Kalra A. 2012. Synergy between Glomus fasciculatum and a beneficial Pseudomonas in reducing root diseases and improving yield and forskolin content in Coleus forskohlii Briq. under organic field conditions. Mycorrhiza. 23(1):35–44. doi: 10.1007/s00572-012-0447-x
- Sivaram L, Mukundan U. 2003. In vitro culture studies on Stevia rebaudiana. In Vitro Cell Dev Biol Plant. 39:520–523. doi: 10.1079/IVP2003438
- Toussaint JP, ST-Arnaud M, Charest C. 2004. Nitrogen transfer and assimilation between the arbuscular mycorrhizal fungus Glomus intraradices Schench and Smith and Ri T-DNA roots of Daucus carota L. an in vitro compartment system. Can J Microbiol. 50:251–260. doi: 10.1139/w04-009
- Vasanthakumar SK. 2003. Studies on beneficial endorhizosphere bacteria in solanaceous crop plants. [M.Sc. (Agri) thesis], Dharwad, Karnataka, India: University of Agricultural Sciences.
- Wilson PW, Knight SC. 1952. Experiments in bacterial physiology. Minneapolis: Burguess, p. 49.
- Woelwer-Rieck U, Lankes C, Wawrzun A, Wüst M. 2010. Improved HPLC method for the evaluation of the major steviol glycosides in leaves of Stevia rebaudiana. Eur Food Res Technol. 231:581–588. doi: 10.1007/s00217-010-1309-4
- Wu SC, Cao ZH, Li ZG, Cheung KC, Wong MH. 2005. Effects of biofertilizer containing N-fixer, P and K solubilizers and AM fungi on maize growth: a greenhouse trial. Geoderma. 125:155–166. doi: 10.1016/j.geoderma.2004.07.003
- Wu OS, Xia RX. 2006. Arbuscular mycorrhizal fungi influence growth, osmotic adjustment and photosynthesis of citrus under well-watered and water stress conditions. J Plant Physiol. 163:417–425. doi: 10.1016/j.jplph.2005.04.024
- Zaidi A, Khan MS, Amil M. 2003. Interactive effect of rhizotrophic microorganisms on yield and nutrient uptake of chickpea (Cicer arietinum L.). Eur J Agron. 19:15–21. doi: 10.1016/S1161-0301(02)00015-1