Abstract
Abiotic stresses, including nitrogen stress (NS), can hamper photosynthesis and cause oxidative damage to plants. Upregulation of the antioxidative defense system and photosynthesis induced by exogenous glycinebetaine (GB) and humic acid (HA) can mitigate the inhibitory effects of NS on plants. In the present investigation, the beneficial effects of exogenously applied GB and HA were examined on growth, leaf N status, photosynthesis, lipid peroxidation, and activities of some key antioxidant enzymes in the seedlings of maize cv. Zhengdan 958 (ZD958) exposed to NS. NS caused a significant reduction in total dry matter of seedlings of ZD958, but both GB and HA proved effective in mitigating this inhibition, hence, the beneficial effects of GB being more pronounced than those of HA. NS led to a considerable decrease in leaf total N and endogenous GB contents, stomatal conductance (g s), net photosynthetic rate (P n), intercellular CO2 concentration (C i), and activities of two key C4 photosynthesis enzymes phosphoenolpyruvate carboxylase (PEPCase) and ribulose-1,5-bisphosphate carboxylase (RuBPCase) as well as of superoxide dismutase (SOD) and peroxidase (POD). This treatment caused an increase in lipid peroxidation, but showed no effect on POD activity. Exogenous application of varying doses of GB resulted in a decrease in lipid peroxidation and C i, and an increase in leaf total N and endogenous glycinebetaine (EGB) content, P n, and activities of RuBPCase, PEPCase, SOD, and catalase (CAT) under NS. In contrast, application of different doses of HA resulted in a decrease in lipid peroxidation, an increase in P n, g s, and C i as well as SOD, CAT, and POD activities without increasing leaf total N and EGB content, and enhanced RuBPCase and PEPCase activities. The present study suggests that exogenous application of GB and HA can induce tolerance in maize plants to NS, but through the regulation of different mechanisms.
Introduction
Although C4 crops such as maize (Zea mays L.) are known for their higher use efficiency of nitrogen (N), CO2, and solar radiation than most C3 crops, N stress (NS) is still one of the major limiting factors for crop yield in arid and semiarid areas of the world (Greenwood Citation1976; Uhart & Andrade Citation1995; Pandey et al. Citation2000; Sage & Zhu Citation2011). Understanding the physio-biochemical mechanisms in maize under NS could be vital for achieving increased crop yield by increasing N use efficiency (Chapin et al. Citation1988; Ding et al. Citation2005). Physiological attributes such as net photosynthetic rate (P n) and stomatal conductance (g s), and activities of some key C4 photosynthesis enzymes, ribulose-1,5-bisphosphate carboxylase (RuBPCase) and phosphoenolpyruvate carboxylase (PEPCase), are directly associated with plant N status and plant dry matter (DM) production under NS (Chapin et al. Citation1987; Meinzer & Zhu Citation1998; Zhao et al. Citation2005; Zhang et al. Citation2011). Leaf N content can reflect the N status in most plants, and some N-containing organic osmolytes such as glycinebetaine (GB) could be a prospective N source in some plants under moderate NS (McCullough et al. Citation1994; Gorham Citation1996). For example, Grattan and Grieve (Citation1985) reported that wheat (Triticum aestivum L.) plants can utilize GB as a source of available N under conditions of moderate NS.
Similar to other environmental stresses, NS disturbs the transport and accumulation of nutrients and water in different plant parts, and causes oxidative stress. Due to the oxidative stress a variety of reactive oxygen species (ROS) are produced in plants subjected to environmental cues including NS (Arora et al. Citation2002; Ashraf Citation2009). The ROS interfere with different organic molecules thereby perturbing key metabolic processes, which result in reduced photosynthesis and subsequently retarded growth (Arora et al. Citation2002; Zhang et al. Citation2007). It has been observed that under stress conditions most plants are capable of counteracting the ROS by overproducing a variety of antioxidant molecules, both enzymatic and non-enzymatic (Arora et al. Citation2002; Ashraf Citation2009). The key antioxidant enzymes which play an effective role in scavenging the ROS are superoxide dismutase (SOD, EC 1.15.1.1), catalase (CAT, EC 1.11.1.6), and peroxidase (POD, EC 1.11.1.7). The activities of these enzymes usually increase under stress conditions, perhaps under NS as well.
GB significantly accumulates in many crop plants, including maize under drought and salt stress (Ashraf & Foolad Citation2007; Zhang et al. Citation2011). However, under NS GB content may possibly decline in shoots of some crop plants (Bowman & Rohringer Citation1970). It could be due to the reason that under NS, GB is degraded to supply N to plants experiencing N deficiency. For example, Grattan and Grieve (Citation1985) observed a maximal decrease in GB in wheat plants growing under N-free regimes. They guessed that GB is degraded in wheat plants to utilize it as a source of N under N deficiency. Under such conditions, exogenous application of GB is reported to be beneficial for improving endogenous N status (Bowman & Rohringer Citation1970) and thereby enhancing photosynthesis and upregulating antioxidant defense system in plants (Yang & Lu Citation2005; Ashraf & Foolad Citation2007; Hoque et al. Citation2008).
Another prospective plant regulator involved in plant stress tolerance is humic acid (HA) (Chen & Aviad Citation1990; Cimrin et al. Citation2010). HA could promote plant growth and enhance stress tolerance by increasing cell membrane permeability, potassium and phosphate uptake, rates of photosynthesis and respiration, and accelerating protein synthesis as well as modulating plant hormone pool (Chen & Aviad Citation1990; Böhme & Thi Citation1997; Cimrin et al. Citation2010; Saruhan et al. Citation2011).
All the above-cited studies report the beneficial effects of GB or HA application on plants exposed to drought stress and salinity stress, but reports on the effects of these regulators on crops, especially maize, under NS are lacking in the literature (Chen & Aviad Citation1990; Yang & Lu Citation2005; Ashraf & Foolad Citation2007; Hoque et al. Citation2008; Ashraf Citation2010; Cimrin et al. Citation2010; Saruhan et al. Citation2011). Therefore, the present study aimed to investigate whether or not exogenous application of varying levels of GB and HA could alleviate the adverse effects of NS on growth, N status, photosynthesis, and antioxidant defense system in maize plants.
Materials and methods
Plant material and growth conditions
Experiments were conducted in a controlled growth room at the College of Life Sciences of Northwest A&F University, Yangling (34°20′ N, 108°24′ E), China. Maize cultivar Zhengdan 958 was used in this study.
Plant growth and experimental design
The seeds were germinated at 28°C for 72 h in Petri dishes placed in dark. The young seedlings were inserted into holes of styrofoam boards placed over plastic containers (inner length, 26 cm; width 18 cm; height 12 cm) containing deionized water initially, which was replaced by half-strength and then by full-strength nutrient solution 4 and 8 d later, respectively (Hoagland & Arnon Citation1950). The growth containers were placed in a growth chamber under the following environmental conditions: average day/night temperature 25/18°C, relative humidity (RH) 60–70%, light intensity 350 µmol m−2 s−1 for 16 h. A black plastic was wrapped around the containers to prevent the roots from exposure to light. The pH of the nutrient solution was maintained regularly at 6.30 (±0.05).
Three seedlings at three-leaf stage were grown in plastic pots (3.8 l) in a growth room. Each pot was filled with acid-washed and sterilized quartz sand up to 20 cm and supplied with distilled water. The seedlings in each pot were thinned to one plant per pot and then treatments initiated.
The non-NS treatment solution (control) and that of NS were supplied with full-strength Hoagland's nutrient solution containing 15 mM NO3 − and Hoagland's nutrient solution containing 0.15 mM NO3 −, respectively (Hoagland & Arnon Citation1950). The 100 and 200 mg l−1 GB media were NS medium supplemented with 100 and 200 mg l−1 GB, respectively (GB supplied by Shiying Chemical Plant, Changping, Beijing). The 100 and 200 mg l−1 HA media were the NS medium supplemented with 100 and 200 mg l−1 HA, respectively (derived from lignitic coal using 0.1 M NaOH, manufactured by Yangling Lvdu Bioecology Technology Co., Ltd., Yangling) (Hai & Mir Citation1998). The seedlings were irrigated with nutrient solutions containing appropriate treatments for 12 d. Each treatment had four replicates. The experiment was repeated once under the same ecological conditions so as to confirm the repeatability of the data for different attributes. Thus, the data of both experiments were pooled (n =8).
Total dry matter determination
The plants were harvested from all pots on day 12 after the initiation of treatments. For determining total dry matter (TDM) the plant material was dried in an oven at 105°C for 15 min, and then at 75°C until constant dry weight attained.
Sampling of leaves and measurement of different parameters
Leaves were excised from four plants on day 12 after the initiation of treatments. All measurements were made on fully developed third or fourth leaf from the top of each plant. Soon after harvesting the leaves at 10:30–11:00 am, they were placed on ice and brought to the laboratory for analysis.
Nitrogen and GB determination
Harvested leaves were first oven-dried at 75°C and then crushed in an electric blender. Total nitrogen was determined following the standard micro-Kjeldahl method (Bao Citation2000). GB was determined in the aqueous extracts of the ground leaf samples following the slightly modified method of Grieve and Grattan (Citation1983).
Photosynthetic parameters
For the measurement of different photosynthetic parameters an automatic portable photosynthesis system (LI-6400; LI-COR Inc., Lincoln, NE, USA) was used. All measurements were made on the second fully developed leaf from the top at 9:00 to 11:00 am. The ambient/instrument conditions were ambient CO2 concentration of 360 µmol mol−1, photosynthetically active radiation 1200 µmol m−2 s−1, leaf temperature 25.5±2°C, and the RH in the leaf chamber 45%.
RuBPCase was determined following Lilley and Walker (Citation1974). Fresh leaf material (0.5 g) was homogenized in 2.5 ml of extraction medium [0.1 M Tricine–HCl (pH 8.4), containing 10 mM MgCl2, 1 mM EDTA, 7 mM β-mercaptoethanol, 5% glycerol (v/v), and 1% polyvinylpyrrolidone (PVP)] and then centrifuged at 10,000 g for 10 min at 4°C. The clear supernatant was used as the crude RuBPCase source. For the quantification of the enzyme, the crude extract was first reacted with 200 M NaHCO3 and 1 M MgCl2, at 25°C for 10 min. The reaction mixture contained 50 mM Tricine–HCl (pH 7.8), 10 mM KCl, 1 mM EDTA, 15 mM MgCl2, 0.21 mM NADH, 5 mM dithiothreitol, 5 mM phosphocreatine, 5 mM ATP, 2 U of creatine phosphokinase, 15 U of phosphoglyceric phosphokinase, 5 U of glyceraldehyde-phosphate dehydrogenase, and 10 mM NaHCO3. The reaction started with the addition of 0.5 mmol of RuBP. The activity of RuBPCase was determined as the oxidation rate of NADH at 340 nm. The activity of PEPCase was appraised following Arnozis et al. (Citation1988). Leaf samples were homogenized in 0.1 M Tricine–HCl buffer (pH 8.4) comprising 10 mM MgCl2, 1 mM EDTA, 7 mM β-mercaptoethanol, 5% glycerol (v/v), and 1% PVP. This whole mixture was centrifuged at 10,000 g for 10 min. The enzyme extract was added to the reaction mixture which contained 0.5 mmol PEP, 3.68 mmol NaHCO3, 0.16 mmol NADH, 11.2 mmol MgCl2, and 112 mmol Tris–NaOH (pH 9.2). PEPCase activity was measured spectrophotometrically by coupling the reaction to the NADH oxidation at 340 nm mediated by 15 U of malate dehydrogenase. The activity of each enzyme was expressed on protein basis (U mg−1 protein).
Preparation of extracts for measuring antioxidant enzyme activities
The fresh leaf material was homogenized in ice-cold 4 ml of 50 mM phosphate buffer (pH 7.8) containing 1% PVP (v/v) in a prechilled pestle and mortar. The homogenates were centrifuged (10,000 g for 20 min at 4°C) and the supernatants used for appraising the activities of the following antioxidant enzymes:
SOD activity was determined by recording a decrease in absorbance at 560 nm of superoxide–nitroblue tetrazolium (NBT) complex by the enzyme. One unit of SOD was considered to be the amount of enzyme required to inhibit NBT reduction by 50% (Dhindsa et al. Citation1981). POD activity was assayed as described by Putter (Citation1974). POD activity was measured with guaiacol. One unit of enzyme activity was taken as the rate of guaiacol which was oxidized in three minutes. CAT activity was assayed by measuring the residual H2O2 by the Tris–HCl reagent following Dhindsa et al. (Citation1981). Absorbance was recorded immediately at 240 nm every 4 min and one unit of enzyme determined the amount necessary to decompose 1 µmol of H2O2 per minute at 25°C.
The activity of each enzyme measured above was expressed as U mg−1 protein. Protein concentration of the crude extract was measured by the method of Bradford (Citation1976).
Determination of malondialdehyde contents
Malondialdehyde (MDA) was extracted with 10% trichloroacetic acid and determined at 450, 532, and 600 nm with 0.6% thiobarbituric acid as described by Zhang et al. (Citation2007).
Data statistical analysis
Data for each of the growth and physio-biochemical parameters were analyzed using the SAS software package (SAS Institute Inc. Citation1996) and analysis of variance for each attribute worked out. Standard errors of all means were worked out. The least significant difference (LSD) was calculated at 5% probability level to examine the difference among mean values.
Results
Seedling growth
The seedlings of maize cv. ZD958 subjected to limited N supply, i.e. NS (0.15 mM NO2 − in growth medium), showed inhibited growth measured as DM, being approximately 40% of control (15 mM NO2 −) at the end of the treatment (). At 12 d after treatments, TDM increased with increasing GB rates, whereas such increase in TDM occurred only at the first dose of HA. However, increase in TDM was greater due to exogenous GB than that due to the same dose of HA under NS ().
Notes: Total dry weights (TDW) were measured at 0 and 12 d after inoculation. Values represent the means±SE (n=8). At the same incubation day, bars with the same letters are not significantly different at P<0.05. Non-stress, NS, NS+GB1, NS+GB2, NS+HA1, and NS+HA2 indicate the standard medium (15 mM NO3 −), NS medium (0.15 mM NO3 −), 100 mg l−1 GB and NS medium, 200 mg l−1 GB and NS medium, 100 mg l−1 HA and NS medium, and 200 mg l−1 HA and NS medium, respectively, as described in Materials and methods.
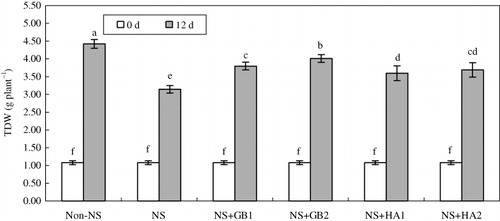
Total N and GB contents
NS induced a decrease in total N content and endogenous glycinebetaine (EGB) content in the seedlings of maize cultivar ZD958. Exogenous application of 100 and 200 mg l−1 GB significantly increased total N content by 8% and 13%, and endogenous GB content by 35% and 55%, respectively, under NS. However, neither total endogenous N content nor EGB content was affected by the application of HA ().
Photosynthesis
The NS treatment decreased leaf P n, g s, C i, and RuBPCase and PEPCase activities significantly in the seedlings of maize cv. ZD958 (). Exogenous application of 100 and 200 mg l−1 GB or HA significantly increased P n by 22% and 33% or 11% and 12%, respectively, under NS. The g s and C i increased due to the application of HA, but there was no difference between the two levels of HA used. However, C i decreased, whereas g s remained unaffected due to the application of GB. The RuBPCase and PEPCase activities increased by 18% and 13%, respectively, due to application of 100 mg l−1 GB, and 33% and 15% at 200 mg l−1 of GB under NS. In contrast, no effect of HA was found on the activities of these two enzymes under NS ().
Table 1. Effects of exogenous GB and HA on photosynthesis in 12-d-old seedlings of cv. Zhengdan 958 under NS.
Lipid peroxidation
NS caused a significant increase in MDA contents in ZD958 seedlings. Exogenously applied 100 and 200 mg l−1 GB significantly decreased MDA levels by 22% and 31%, respectively, under NS. Exogenous application of 100 and 200 mg l−1 of HA also caused a significant decrease by 15% and 16% in MDA content under NS ().
Table 2. Effects of exogenous GB and HA on antioxidant parameters in 12-d-old seedlings of cv. Zhengdan 958 under NS.
Activity of antioxidant enzymes
A significant decrease (25% and 31%) in SOD and CAT activities whereas no change in POD activity was observed in the maize seedlings due to NS (). Exogenously applied 100 and 200 mg l−1 GB resulted in a significant increase in SOD activity by 9% and 18%, and CAT activity by 15% and 27%, respectively. However, POD activity remained unchanged. Exogenously applied 100 and 200 mg l−1 HA resulted in a significant increase in SOD activity by 7% and 12%, CAT activity by 14% and 17%, and POD activity by 7% and 7%, respectively, under NS ().
Discussion
Although maize plants being C4 use N more efficiently than most C3-type crops (Uhart & Andrade Citation1995; Pandey et al. Citation2000; Sage & Zhu Citation2011), NS suppressed their plant growth and DM accumulation (). Decreased TDM production due to N shortage in the maize plants was found to be associated with reductions in leaf photosynthetic capacity as earlier observed by Uhart and Andrade (Citation1995) and it was mainly ascribed to a smaller total N content and GB content in the plants ( and ). The ameliorative effects of GB and HA on most plants exposed to various stresses have been widely reported in the literature (Chen & Aviad Citation1990; Demiral & Turkan Citation2004; Ashraf & Foolad Citation2007; Cimrin et al. Citation2010). For example, GB was reported to be utilized as an N source in wheat under N-deficit conditions (Grattan & Grieve Citation1985). However, the defensive roles of GB and HA in plants exposed to NS are yet to be fully explored. In this study, the evidence of NS can be seen from growth inhibition of the seedlings of maize cv. ZD958 (). Despite the protective roles of both GB and HA in plants against NS, the mitigation effects of GB increased with increasing external GB levels, whereas such increasing effect of HA occurred only at its lower level. Overall, the mitigating effect of GB was more pronounced than that of HA ().
Plants have adopted a variety of metabolic strategies to judiciously utilize endogenous N sources under the conditions of N shortage (Grattan & Grieve Citation1985). Except the availability of inorganic N source, the N bound in organic molecules can also be a good source of N under N deficiency. For example, in Hibiscus tiliaceus the methylated onium compounds comprise 6% of the N in old leaves and 10% in the young leaves (Gorham Citation1996). In drought stressed plants of cotton (Gossypium hirsutum L.), approximately 300 mmol GB kg−1 dry weight was reported to be accumulated which accounts for about 8–10% of the total N (Gorham Citation1996). GB could be degraded in plants such as wheat to utilize it as a source of N under N-deficit conditions (Grattan & Grieve Citation1985). In our study, NS led to a decrease in total N and GB contents in the maize seedlings (). The improvement in NS tolerance by exogenous GB but not HA was found to be associated with increased intracellular GB accumulation (Zhang et al. Citation2009; Cimrin et al. Citation2010). Thus, it could be suggested that exogenously applied GB contributes to increased total N and GB content in the maize seedlings exposed to NS (). However, the increased total N content in the maize seedlings was not related to exogenously applied HA, suggesting that HA mitigates NS not by enhancing N accumulation, but by other mechanisms not fully known yet (Cimrin et al. Citation2010; Saruhan et al. Citation2011). Conversely, GB could mitigate NS by its own degradation to the N pool within the plant (Demiral & Turkan Citation2004; Ashraf & Foolad Citation2007). GB improved N tolerance more than HA did in the maize seedlings.
Leaf photosynthetic rate depends on a multitude of physio-biochemical processes, including g s, E, C i, functioning of PSII, and activities and levels of key enzymes involved in carbon dioxide fixation. A study in wheat has shown that N deficiency caused a marked reduction in leaf P n and level and activity of Rubisco (Ding et al. Citation2005). In sorghum (Sorghum bicolor L.; a C4 plant) N deficiency reduced the quantities of both PEPcase and Rubisco (Maranville & Madhavan Citation2002). In sunflower, Ciompi et al. (Citation1996) reported reduced leaf P n under N deficiency accompanied by an increase in g s and C i. The suppression in leaf photosynthetic rate in N-deficient sunflower plants at light saturation level was found to be due to a more decrease in mesophyll activity than that in stomatal regulation. Cruz et al. (Citation2003) have drawn similar inferences while working with cassava (Manihot esculenta L.). In contrast, our findings show that under NS, both g s and C i decreased simultaneously with decrease in leaf P n of maize (a C4 crop); therefore, the suppression in stomatal conductance seems to be one of the major causes of decrease in maize leaf P n under N-deficient conditions (). These results agree with those of Zhao et al. (Citation2005) in maize. However, decrease in RuBPCase and PEPCase activities under NS have also been noted in this study. The mesophyllic activity is another cause of limiting maize leaf P n under NS (Cruz et al. Citation2003). Decrease in P n due to N deficiency has been reported to be one of the major causes that limit plant growth and productivity (Uhart & Andrade Citation1995; Zhao et al. Citation2003, Citation2005).
GB accumulates in most plants under drought stress. It accumulates primarily in chloroplast where it protects thylakoid membrane, thereby sustaining photosynthetic capacity (Ashraf & Foolad Citation2007). In a study with maize, Yang and Lu (Citation2005) reported that exogenous application of GB can effectively promote rate of photosynthesis in salt-stressed plants. HA is also known to increase rates of photosynthesis and respiration in plants, especially under stressful environments (Chen & Aviad Citation1990; Cimrin et al. Citation2010; Saruhan et al. Citation2011). Although a comprehensive knowledge on the use of GB and HA is a prerequisite for their commercial utilization for achieving improved crop drought or salinity stress tolerance particularly in high-valued crops, unfortunately, little research has so far been carried out to appraise the effectiveness of GB or HA on photosynthesis in plant species under N-deficit conditions (Demiral & Turkan Citation2004; Ashraf & Foolad Citation2007; Cimrin et al. Citation2010; Saruhan et al. Citation2011). In our study, either exogenous GB or HA increased P n under NS. The g s and C i were increased by the application of HA, while C i was decreased, but g s not affected by exogenous application of GB. The RuBPCase and PEPC activities were also increased only due to GB, but not due to HA application (). Therefore, the mitigating role of HA on photosynthesis in maize plants under NS is suggested to be due to stomatal factors, while that of GB due to mesophyllic activity.
NS can indirectly cause the production of ROS that can cause oxidative damage to cellular ultrastructures and biomolecules including lipids (Greenwood Citation1976; Maranville & Madhavan Citation2002). In our study, increased levels of MDA have been found in N-stressed maize seedlings (). These results agree with those of Pandey et al. (Citation2000) in maize. Compatible solutes such as GB protect cellular components from different stresses. However, in our study reduced levels of MDA in N-stressed maize plants due to exogenous application of GB or HA protected the plants from oxidative damage most probably to lipid membranes (). However, GB was found to be more effective than HA probably because of their differential role in protecting the N-stressed plants against oxidative damage.
It is now evident that both GB and HA promote antioxidant defense systems and improve stress tolerance to drought or salinity (Cimrin et al. Citation2010; Saruhan et al. Citation2011). GB is believed to have a beneficial effect on enzyme and membrane integrity in plants subjected to stressful environments (Demiral & Turkan Citation2004; Ashraf & Foolad Citation2007). Zhang et al. (Citation2011) reported that exogenous GB application increased antioxidant enzyme activities, but reduced MDA accumulation in maize under drought. Under salt stress, GB increased CAT activity in cultured tobacco (Nicotiana tabacum L.) cells (Hoque et al. Citation2008). The HA compounds may have various biochemical effects in terms of enhanced protein synthesis such as antioxidative enzymes (Cimrin et al. Citation2010; Saruhan et al. Citation2011). In the current study, NS decreased SOD and CAT activities; however, this decrease was found to be mitigated by exogenously applied GB or HA (). HA but not GB provides protection against NS also by increasing the activity of POD. However, the increase in POD activity was not correlated with NS tolerance in our study, suggesting that this enzyme is not sensitive to N supply (Zhao et al. Citation2005). In contrast, from the present study it is evident that the activities of SOD and CAT could be used as biomarkers for N deficiency in maize plants (). The above results suggest that SOD, CAT, and POD may function differentially under NS as well as other stresses. For example, increased POD activity positively correlated with salt tolerance, but not with NS tolerance, suggesting that POD activity may be more responsive to salt than to NS.
From the above-reported data it is possible to infer that GB and HA acted differently to alleviate NS in maize plants, because the former caused improvement in tissue N status, endogenous GB content, and activities of RuBPCase and PEPCase, while the latter caused stomatal regulation by increasing g s and C i.
Conclusion
Nitrogen deficiency decreased leaf total N and endogenous GB contents, P n, g s, C i, and RuBPCase and PEPCase activities as well as the activities of SOD and POD in maize plants, thereby causing reduced DM accumulation. Decreased maize leaf photosynthetic capacity due to N deficiency was found to be associated with limited stomatal regulation and mesophyllic activity. Exogenously applied GB and HA induced tolerance to NS in cv. ZD958 seedlings by increasing P n and activities of SOD and CAT, and decreasing lipid peroxidation. In addition, GB offers greater protection against NS compared to HA, but the specific mechanism of GB-induced NS tolerance was different from that of HA-induced NS tolerance. Exogenous GB only increased leaf total N and endogenous GB content and activities of RuBPCase and PEPCase, while it decreased C i under NS. In contrast, exogenous HA only increased g s and C i as well as POD activities. Further research is required to explicate the molecular mechanisms and signaling pathways involved in GB- or HA-induced NS tolerance in plants.
Acknowledgments
This work was jointly supported by the China Postdoctoral Science Foundation, Chinese Universities Scientific Fund (QN2009069), and the Foundation of State Key Laboratory of Soil Erosion and Dryland Farming (10501-J-2).
References
- Arnozis PA, Nelemans JA, Findenegg GR. 1988. Phosphoenolpyruvate carboxylase activity in plants grown with either NO3− or NH4+ as inorganic nitrogen source. J Plant Physiol. 132:23–27. 10.1016/S0176-1617(88)80177-9
- Arora A, Sairam RK, Srivastava GC. 2002. Oxidative stress and antioxidative systems in plants. Curr Sci. 82:1227–1238.
- Ashraf M. 2009. Biotechnological approach of improving plant salt tolerance using antioxidants as markers. Biotechnol Adv. 27:84–93. 10.1016/j.biotechadv.2008.09.003
- Ashraf M. 2010. Inducing drought tolerance in plants: some recent advances. Biotechnol Adv. 28:169–183. 10.1016/j.biotechadv.2009.11.005
- Ashraf M, Foolad MR. 2007. Roles of glycinebetaine and proline in improving plant abiotic stress resistance. Environ Exp Bot. 59:206–216. 10.1016/j.envexpbot.2005.12.006
- Bao SD. 2000. Soil and agricultural chemistry analysis. Beijing: China Agriculture Press.
- Böhme M, Thi LH. 1997. Influence of mineral and organic treatments in the rizosphere on the growth of tomato plants. Acta Hort. 450:161–168.
- Bowman MS, Rohringer R. 1970. Formate metabolism and betaine formation in healthy and rust-affected wheat. Can J Bot. 48:803–811. 10.1139/b70-110
- Bradford MM. 1976. A rapid and sensitive method for the quantitation of microgram quantities of protein utilizing the principle of protein-dye binding. Anal Biochem. 72:248–254. 10.1016/0003-2697(76)90527-3
- Ciompi S, Gentili E, Guidi L, Soldatini GF. 1996. The effect of nitrogen deficiency on leaf gas exchange and chlorophyll fluorescence parameters in sunflower. Plant Sci. 118:177–184. 10.1016/0168-9452(96)04442-1
- Chapin FS, Clarkson DT, Lenton JR, Walter CHS. 1988. Effect of nitrogen stress and abscisic acid on nitrate absorption and transport in barley and tomato. Planta. 173:340–351. 10.1007/BF00401021
- Chapin FS, Walter CHS, Clarkson DT. 1987. Growth response of barley and tomato to nitrogen stress and its control by abscisic acid, water relations and photosynthesis. Planta. 173:352–366. 10.1007/BF00401022
- Chen Y, Aviad T. 1990. Effect of humic substances on plant growth. In: Mc Carthy P, Clap CE, Malcom RL, Bloom PR, editors. Humic substances in soil and crop sciences: selected readings. Madison, (WI): ASA and SSSA; p. 161–186.
- Cimrin KM, Türkmen O, Turan M, Tuncer B. 2010. Phosphorus and humic acid application alleviate salinity stress of pepper seedling. Afr J Biotechnol. 9:5845–5851.
- Cruz JL, Mosquim PR, Pelacani CR, Araujo WL, DaMatta FM. 2003. Photosynthesis impairment in cassava leaves in response to nitrogen deficiency. Plant Soil. 257:417–423. 10.1023/A:1027353305250
- Demiral T, Turkan I. 2004. Does exogenous glycinebetaine affect antioxidative system of rice seedlings under NaCl treatment? J Plant Physiol. 161:1089–1100. 10.1016/j.jplph.2004.03.009
- Dhindsa RS, Plumb-Dhindsa P, Throne TA. 1981. Leaf senescence: correlation with increased levels of membrane permeability and lipid peroxidation and decreased levels of superoxide dismutase and catalase. J Exp Bot. 32:93–101. 10.1093/jxb/32.1.93
- Ding L, Wang KJ, Jiang GM, Biswas DK, Xu H, Li LF, Li YH. 2005. Effects of nitrogen deficiency on photosynthetic traits of maize hybrids released in different years. Ann Bot. 96:925–930. 10.1093/aob/mci244
- Gorham J. 1996. Glycinebetaine is a major nitrogen-containing solute in the Malvaceae. Phytochemistry. 43:367–369. 10.1016/0031-9422(96)00312-3
- Grattan SR, Grieve CM. 1985. Betaine status in wheat in relation to nitrogen stress and to transient salinity stress. Plant Soil. 85:3–9. 10.1007/BF02197795
- Greenwood EAN. 1976. Nitrogen stress in plants. Adv Agron. 28:1–35.
- Grieve CM, Grattan SR. 1983. Rapid assay for determination of water soluble quarter NRA-amino compounds. Plant Soil. 70:303–307. 10.1007/BF02374789
- Hai SM, Mir S. 1998. The lignitic coal derived HA and the prospective utilization in Pakistan's agriculture and industry. Sci Tech Dev. 17:32–40.
- Hoagland DR, Arnon DI. 1950. The water culture method for growing plants without soils. Calif Agric Exp Station Circular. 347:1–32.
- Hoque MA, Banu MNA, Nakamura Y, Shimoishi Y, Murata Y. 2008. Proline and glycinebetaine enhance antioxidant defense and methylglyoxal detoxification systems and reduce NaCl-induced damage in cultured tobacco cells. J Plant Physiol. 165:813–824. 10.1016/j.jplph.2007.07.013
- Lilley RM, Walker DA. 1974. An improved spectrophotometric assay for ribulose bisphosphate carboxylase. Biochim Biophys Acta. 358:226–229. 10.1016/0005-2744(74)90274-5
- Maranville JW, Madhavan S. 2002. Physiological adaptation for nitrogen use efficiency in sorghum. Plant Soil. 245:25–34. 10.1023/A:1020660504596
- McCullough DE, Giradin P, Mihajlovic M, Guilera AA, Tollenaar M. 1994. Influence of N supply on development and dry matter accumulation of an old and new maize hybrid. Can J Plant Sci. 74:471–477. 10.4141/cjps94-087
- Meinzer FC, Zhu J. 1998. Nitrogen stress reduces the efficiency of the C4CO2 concentrating system, and therefore quantum yield, in Saccharum (sugarcane) species. J Exp Bot. 49:1227–1234.
- Pandey RK, Maranville JW, Admou A. 2000. Deficit irrigation and nitrogen effects on maize in a Sahelian environment. I. Grain yield and yield components. Agric Water Manage. 46:1–13. 10.1016/S0378-3774(00)00073-1
- Putter J. 1974. Peroxidases. In: Bregmeyer HU, editor. Methods of enzymatic analysis. New York: Academic Press; p. 685–690.
- Sage RF, Zhu XG. 2011. Exploiting the engine of C4 photosynthesis. J Exp Bot. 62:2989–3000. 10.1093/jxb/err179
- Saruhan V, Kusvuran A, Babat S. 2011. The effect of different humic acid fertilization on yield and yield components performances of common millet (Panicum miliaceum L.). Sci Res Essays. 6:663–669.
- SAS Institute Inc. 1996. Getting started with PROC ANOVA. Cary (NC): SAS Institute Inc.
- Uhart SA, Andrade FH. 1995. Nitrogen deficiency in maize. I. Effects on crop growth, development, dry matter partitioning, and kernel set. Crop Sci. 35:1376–1383. 10.2135/cropsci1995.0011183X003500050020x
- Yang X, Lu C. 2005. Photosynthesis is improved by exogenous glycinebetaine in salt-stressed maize plants. Physiol Plant. 124:343–352. 10.1111/j.1399-3054.2005.00518.x
- Zhang LX, Gao M, Li SQ, Li SX, Liang ZS. 2011. Modulation of plant growth, water status and antioxidative system of two maize (Zea may L.) cultivars induced by exogenous glycinebetaine under long term mild drought stress. Pak J Bot. 43:1587–1594.
- Zhang LX, Li SX, Zhang H, Liang ZS. 2007. Nitrogen rates and water stress effects on production, lipid peroxidation and antioxidative enzyme activities in two maize (Zea mays L.) genotypes. J Agron Crop Sci. 193:387–397. 10.1111/j.1439-037X.2007.00276.x
- Zhang LX, Li SX, Liang ZS. 2009. Differential plant growth and osmotic effects of two maize (Zea mays L.) cultivars to exogenous glycinebetaine application under drought stress. Plant Growth Regul. 58:297–305.
- Zhao D, Raja KR, Kakania VG, Reddyb KR. 2005. Nitrogen deficiency effects on plant growth, leaf photosynthesis, and hyperspectral reflectance properties of sorghum. Europ J Agron. 22:391–403. 10.1016/j.eja.2004.06.005
- Zhao D, Reddy KR, Kakani VG, Read JJ, Carter GA. 2003. Corn (Zea mays L.) growth, leaf pigment concentration, photosynthesis and leaf hyperspectral reflectance properties as affected by nitrogen supply. Plant Soil. 257:205–217. 10.1023/A:1026233732507