Abstract
The holostemparasitic plant Cuscuta parasitizes various plants and sucks nutrients from the host stem. We used Cuscuta japonica as the parasite and Momordica charantia as the host plant, and described their interaction. The parasitized Momordica stems started swelling as a hypertrophic response within 3 days after parasitization. Concurrently, the Cuscuta stem grew rapidly and developed bigger scale leaves than usual. Parasitized Momordica stems reduced photosynthetic activity. Histological observation revealed no programmed cell death but an increased number of vascular bundles in the Momordica stem, especially near the Cuscuta hyphae. The defensive response of Momordica mainly involved the SA pathway. Drastic increase of tZ- and DZ-type cytokinins in Momordica stems would play an important role for hypertrophy. Cuscuta had higher cZ endogenously and our results imply that each subtype of CK might play different roles during parasitization process. Comprehensive plant hormone analysis provides new insights into plant interaction studies.
Introduction
Plants are sessile organisms that have evolved unique strategies for interacting with various environmental changes as well as dealing with the biological influence of other living organisms. These can roughly be divided into abiotic stress responses and biotic responses (Huang et al. Citation2011; Kadioglu et al. Citation2012). Pathogenic responses are typical examples of biological interactions in plants. These include interactions with bacteria, virus, fungi, and animals (e.g. parasitic nematodes and herbivorous insects). In contrast, less is known about plant–plant interactions. One early focus of plant–plant interactions was on alleropathy (alleles and pathos mean ‘each other’ and ‘harm’, respectively in Latin), as initiated by Hans Moorish in 1937 (Blum Citation2011). He examined volatile compounds in plant–plant interactions. Another type of plant–plant interaction is between parasitic plants and host plants (Stewart & Press Citation1990; Yoder Citation1999). All parasitic plants develop haustoria and suck nutrients from host plants (Kuijt Citation1969; Heide-Jørgensen Citation2008). This interaction is based on a direct connection through haustoria of parasitic plants. As opposed to grafts, which also form direct plant connections but are limited to closely related species, parasite plant–host plant interactions normally involve different taxa. This point leads to an intriguing diversity in the interactions of parasitic plants. The full range of interactions, however, remains poorly understood.
Some previous studies on parasitic plant interactions have dealt with carbon and nitrogen flows (Hibberd & Jeschke Citation2001), and the translocation of chemical compounds (including metabolites, small proteins, and mRNA) (Birschwilks et al. Citation2006, Citation2007; LeBlanc et al. Citation2012). While, others focused on defensive responses from host plants to parasitic plants, i.e. on resistance or incompatibility by chemicals secreted from the hosts (Wanek & Richter Citation1993; Singh & Singh Citation1997; Bringmann et al. Citation1999; Werner et al. Citation2001; Farah Citation2007). For example, there is a variety to prevent Striga haustoria invasion (Yoshida & Shirasu Citation2009). In Cuscuta parasitization, it is widely known that Cuscuta cannot parasitize Ipomoea sp. due to its chemical defense, although Cuscuta can parasitize most other host plants without encountering special resistance.
There are several types of pathogenic defensive systems in host plant. For instance, microbial- or pathogen-associated molecular patterns (MAMPs or PAMPs), effectors triggered immunity that is a type of counter-defense strategy, and HR (hypersensitive response [HR]) that prevents the spread of infection by microorganisms to plants (Chisholm et al. Citation2006; Dodds & Rathjen Citation2010). Plant hormone plays an important role for these defensive system. In the trigger defense system, particularly SA accumulation leads to pathogenesis-related proteins (PR) gene induction and systemic acquired resistance (SAR). HR is also caused by SA leads to PR and results in SAR. SAR is a whole-plant response to the pathogen after the ectopic response. As the interaction between Cuscuta and the host plant is one between plants, it is uncertain whether host plants utilize the same defensive mechanism or not. Furthermore, other plant hormone (e.g. cytokinin [CKs], jasmonates [JA], ethylene [ET], and auxin [IAA]) are also involved in defensive response. This calls for studying the interaction between parasitic and host plants in view of comprehensive plant hormone analysis.
In Cuscuta parasitic plant interaction, there were a few reports about plant hormone. Previously, Runyon et al. (Citation2010) found hypersensitive-like response (HLR) in tomatoes (Solaum lycopersicum), which was provoked by Cuscuta pentagona. HLR was observed only in 20-day-old tomatoes (both at hypocotyls and petiole). SA, total JA (cis- and trans-JA) as well as fatty acids (C18:2 and C18:3) were increased in parasitized 20-day-old tomatoes. While, a tomato mutant (NahG: SA deficient mutant and Jai1: JA insensitive) did not show clear HLR, as opposed to the wild type. Although plant hormones play important roles for many plant interactions, including pathogenic responses, only little plant hormone research has been conducted on Cuscuta. Moreover, only few plant hormones were investigated in these studies. For example, indole-3-acetic acid was measured, but hormones conjugated with amino acids were not (Löffler et al. Citation1999). Cytokinins and gibberellins were rarely measured due to technical difficulties, but these are also very important. Moreover, little is known about influence of hormonal changes to Cuscuta, such as effect to haustoria induction and reciprocal interaction with host plant.
We firstly tested several host plant species for Cuscuta parasitization and also observed Cuscuta plant interaction in the field, in order to find interesting interactive relationship. From such preliminary test, we found outstanding hypertrophic response in the host plant stem of the family Cucurbitaceae during parasitic interaction. This hypertrophy can be a kind of resistance but did not appear to be typical serious resistance to Cuscuta. Appearance of this hypertrophy response is different from gall and other reported phenomena. We chose Momordica as host plant in a family Cucurbitaceae, because it grows big enough from seeds with short time and is easy to culture at green house. Since most host plants of Cuscuta do not resist or show such hypertrophy, it is interesting to investigate this enigmatic phenomenon further. Histological observation was necessary to compare other plant resistances and find out if the phenomenon includes programmed cell death (PCD) or not. Given that plant hormone play important role for many plant response, comprehensive plant hormone profiling would be particularly important. Here, we described general characteristics of Momordica hypertrophy response as well as morphological and physiological changes in Cuscuta.
Methods
Sample preparation
Cuscuta japonica seeds were soaked in concentrated sulfuric acid for 15 min and washed with water (the surface of the seeds was peeled off). Seeds were then placed on cotton gauze soaked with water and incubated at 25°C with white light (fluorescent lamp: Hitachi FL20SS ECW/18X Panasonic). Seedlings 7 days after germination (about 10 cm length) was used for parasitization.
The in vitro haustoria induction method was modified according to previously described articles (Furuhashi et al. Citation2012). Seedlings were then sandwiched between 2 plastic plates (25 mm×40 mm; 0.5 mm plate thickness) in order to stimulate them physically. The distance between plates for Cuscuta was 0.3. Then, the seedlings were placed under far-red light (1–2 W/m2) for 15 min, followed by placement in a dark room at 25°C for 2 days. Far-red light was provided by a lamp (Toshiba FL-20S FR-74) filtered with Deleglass A900 (Asahi kasei company). All seedling manipulations were done under green light in darkness.
Momordica charantia seeds (purchased from Yae Nougei Company in 2012) were in a flower pot filled with vermiculites. Plants were incubated in a greenhouse with daily watering, where room temperature was kept at 30°C and room light followed a 12 h light and dark cycle.
Seven-day-old Cuscuta seedlings were attached with surgical tape to 9-day-old Momordica seedling stems between the first leaf and cotyledon. Sampling was done at stage 1 (30–36 h after attachment), stage 2 (5 days after attachment), and stage 3 (8 days after attachment). Cuscuta and the Momordica stem were excised and collected. The apical part was 1.5 cm from the apex. As shown in a previous article (Furuhashi et al. Citation2012), the Cuscuta seedling apical part (near apex) and haustoria-forming part were not biochemically the same and thus we separated the apical and haustoria-forming part.
Growth measurement
Momordica stem and leaf length at all stages were measured using a caliper. Stems were defined as the sections between cotyledons to first/second leaf, between first/second leaf and third leaf, and between third and fourth leaf. Leaves were first/second leaf to seventh leaf (Figure S1Footnote1). Replication was n=12.
Chlorophyll fluorescence measurements by FluoroCAM
About 1 cm of the apical part of Cuscuta seedlings, Momordica stem tissue, and leaves were used for photosynthetic measurements (n=12). The induction kinetics of chlorophyll fluorescence was measured during illumination (350 µmol m−2 s−1, 120 s) using a chlorophyll fluorescence monitoring system (FluorCam; Photon Systems Instruments). Chlorophyll fluorescence parameters were determined using a pulse-modulated fluorometer (PAM 101/103; Walz) as previously described by Higuchi-Takeuchi et al. (Citation2011). The level of minimum fluorescence (Fo) was recorded after dark adaptation for at least 20 min. The level of maximum fluorescence (Fm) was obtained by applying a 0.8-s saturating light pulse (2000 µmol m−2 s−1) from a light source (KL 2500; Schott). The variable fluorescence (Fv) was calculated as Fm−Fo. Actinic light was supplied by a KL1500 lamp. The Fv/Fm was calculated as (Fm−Fo)/Fm. The level of maximum fluorescence during illumination (Fm′) was obtained with a saturating pulse during actinic illumination. The level of minimum fluorescence during illumination (Fo′) was obtained after turning off the actinic light. The Fs was the level of fluorescence yield during illumination. Variable fluorescence during illumination (Fv′) was calculated as Fm′−Fo′. Quantum yield of open PSII in the steady state (Fv′/Fm′) was calculated as (Fm′–Fo′)/Fm′. Effective quantum yield of FII was calculated as (Fm′–Fs)/Fm′. NPQ was calculated as (Fm–Fm′)/Fm′. qP was calculated as (Fs–Fo′)/(Fm′–Fo′).
Technobit histology
Cuscuta seedlings (apical and haustoria) and Momordica stem tissues were cut and fixed in FAA solution (ethanol:glacial acetic acid:formaline:water= 45:2.5:2.5:50) at room temperature overnight. Samples were then dehydrated in a graded series of ethanol (50, 70, 90, and 100% twice) for 30 min each. Samples were embedded in Technobit 7100 resin (Kluzer). Resin was trimmed and slice into 8–10 µm sections using a microtome (Leica RM 2135) with a TC65 tungsten knife (Thermo). Sections were stained with 0.1% toluidine blue in 50 mM phosphate buffer (pH 7.0) and examined under an Olympus light microscope.
Vibratome histology
Momordica stem tissues with Cuscuta were cut and fixed in FAA solution (ethanol:glacial acetic acid:formaline:water = 45:2.5:2.5:50) at room temperature overnight. Samples were then embedded into 1% agarose gel. Gel was trimmed into appropriate size for the vibratome machine (DSK, Liner Slicer pro7) with razor. Sections (60 µm thick) were stained with 0.1% toluidine blue in 50 mM phosphate buffer (pH 7.0) and examined under an Olympus light microscope.
Plant hormone measurement
About 1 cm of the apical part and haustoria part of Cuscuta seedlings, and Momordica stem tissue at all stages were used for plant hormone analysis. Extraction and determination of plant hormones were performed with an UPLC-MS/MS (AQITY UPLC™ System/Xevo-TQS; Waters) with an ODS column (AQUITY UPLC BEH C18, 1.7 µm, 2.1×100 mm, Waters) (Kojima et al. Citation2009; Kojima & Sakakibara Citation2012).
Statistical data analysis
Statistical analyses were performed with R statistical software (http://cran.r-project.org) and Microsoft Excel. Significant changes in anatomical measurements were used only when at p<0.05 according to the t-test.
Results
Plant feature and growth during parasitization process
Cuscuta twining and haustoria development was completed at stage 1 (a). At this stage, invasion of haustoria was not deep. Thus, only the epidermal region of the Momordica stem was damaged, and haustoria did not reach to Momordica vascular bundles. Cuscuta seedling color was greenish, even more so than before parasitization.
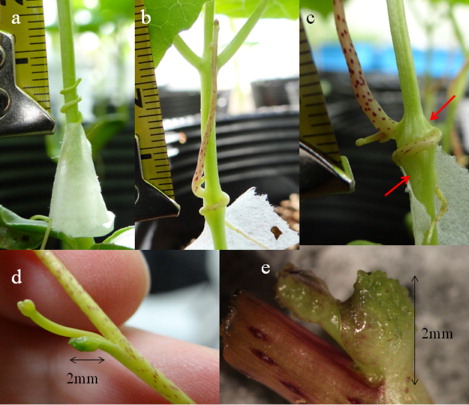
Momordica stems started showing hypertrophy (especially at the parasitized side) at stage 2 (b), which is within a few days after parasitization. The apical part of Cuscuta seedlings stood up and started elongation approximately 3.2 (±1.1, n=12) cm from the parasitized part. The Cuscuta seedling stem color became more yellowish and purple color dots were found on the stem (b).
At stage 3, Momordica stems were swelled into a regular round ball shape (c). Parasitized stems reached double the diameter size (4.5 mm±0.7, n=12) of non-parasitized Momordica stems (2.1 mm±0.2, n=12), and the color was less greenish with brownish color traces of hyphae invasion. From stage 2 to 3, Cuscuta grew rapidly. Cuscuta reached almost the same height as Momordica, because Cuscuta elongated approximately 16.7(±6.1, n=12) cm from the parasitized part. The diameters of Cuscuta stems were also bigger (a–c), and lateral shoot elongation from axillary buds started. Scale leaves of Cuscuta were always found at the nearby axillary buds. Scale leaves became bigger, reaching 2 mm in length (d,e). The apex part of the leaf was greenish, but it was formed into an irregular shape, which has not been seen in any Cuscuta plant interaction so far. In our experiments we never observed a scale leaf over 5 mm in length with a normal leaf shape.
Momordica formed a total of six to seven leaves until stage 3. The first and second leaves of Momordica were not significantly different in size and developed same round shape. The development of the stem between cotyledon and first/second leaf appeared to cease before stage 2. The growth of the first/second leaf ceased before stage 3. There was no statistically significant difference in Momordica leaf and stem length at each stage with and without parasitisation (Figures S2–S5).
Photosynthesis activity
There were no significant differences in Momordica leaves in all photosynthetic activity factors (Fv/Fm, Fv′/Fm′, qP, qN) with and without parasitisation (a). Nevertheless, the parasitized part of the Momordica stems decreased in Fv/Fm and Fv′/Fm′ from stage 2 (b). In Cuscuta, photosynthetic activity was not influenced at stage 1 but Fv/Fm was reduced from stage 2 (c). The qN value dropped conspicuously from stage 2. Cuscuta scale leaves showed relatively low intensity of Fv/Fm and Fv′/Fm′, and these values were indeed the lowest. Apart from scale leaf, the difference between the apical part and haustoria part as well as the intermediate purple part (only at stage 3) was not statistically significance.
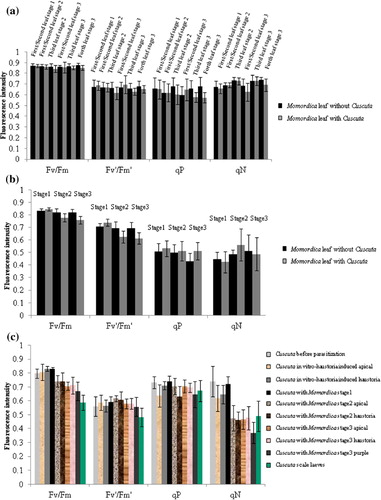
Histological observation of Momordica and Cuscuta stems during the parasitization process
Eight vascular bundles were formed in rectangular-shaped, non-parasitized Momordica stems (a–c). Four vascular bundles at the corner have several bigger lignified xylem vessels which are surrounded by phloems. At stage 1, Cuscuta haustoria invaded Momordica stems on the surface, and there were no significant morphological changes (d). At stage 2 with parasitization, Momordica stems started hypertrophy from the Cuscuta-attached side, and the shape became rounder (a and b). Parenchyma cells were clearly enlarged and proliferation was observed. The number of vascular bundles of Momordica was increased at stage 2. At this stage, the increase in Momordica vascular tissues occurred rather at the Cuscuta-attached side, and the increase appeared to originate from the cambium. At the same time, Cuscuta hypae reached the vascular bundles. At stage 3, the number of vascular bundles in Momordica increased further, and especially developed vascular bundles were typically observed near Cuscuta hypae, although non-parasitized Momordica stems at both stage 2 and stage 3 did not differ in their features or in the number of vascular bundles (a–d). The increased numbers of vascular bundles were xylem rich. In any stage, there was no PCD (as HR) and conspicuous resistance in view of morphology.
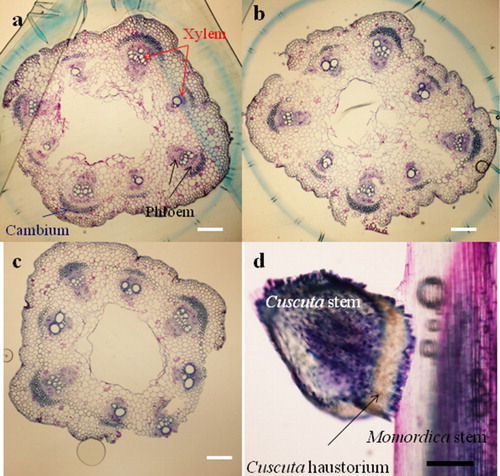
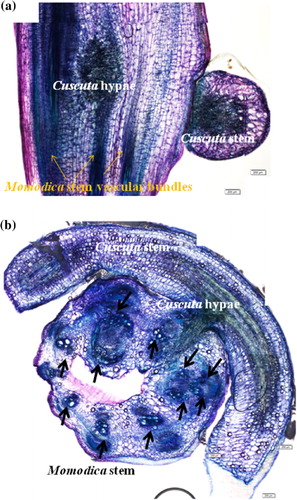
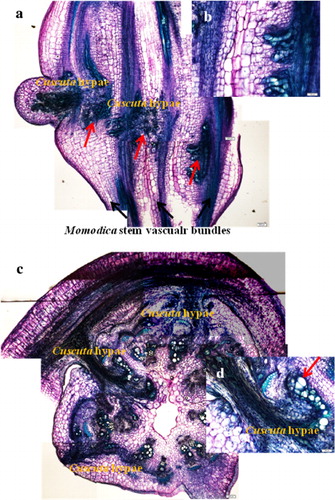
Cuscuta seedlings before parasitization have six vascular bundles. After parasitizing Momordica, Cuscuta fully developed vascular tissues. Thus, from stage 2, the haustoria part increased the number of vascular bundles, and secondary phloem and xylems were formed (a–e). At stage 3, the apical part, the intermediate purple part of the stems as well as the haustoria part grew in size and a more lignified xylem was clearly visible (f–h). Compared with seedlings before parasitization, the apical part was more developed and matured.
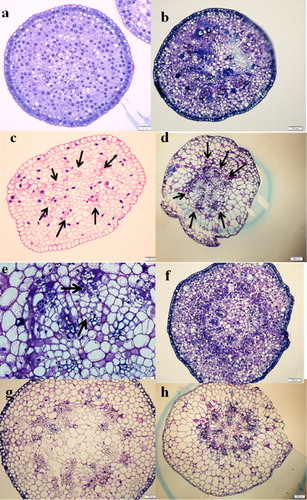
Plant hormonal changes in Momordica stems
Almost no hormonal changes were detected in Momordica stems at stage 1. All types of CKs (iP, tZ, DZ, cZ) increased in Momordica stems at stage 2 (). Especially, of tZ and DZ types of CKs increased conspicuously, and the absolute concentrations were much higher than those in Cuscuta haustoria. In general, the hormone levels at stage 3 tended to be lower than those at stage 2.
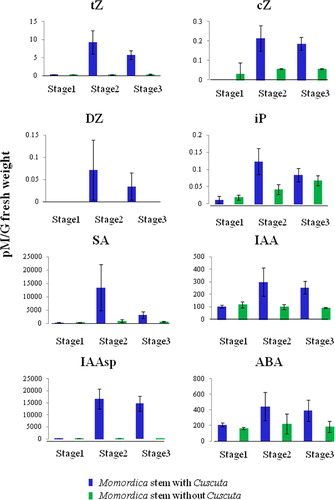
There was no significant change in GA groups (especially active form 1 and 4, and GA1 was not detectable); as SD was high and there was no correlation to the synthetic pathway.
SA increased at stage 2 (), i.e. a few days (1–3 days) after parasitization. The absolute concentration was much higher than that in Cuscuta. At stage 3, SA dropped. With regard to JA, there was no statistically significant change (Figure S6).
Both the IAA free form and the amino acid conjugated form (especially IAA-Asp) increased at stage 2 (). The abundance of the amino acid conjugated form was much higher than that of the free form. ABA increased at stage 2 and kept the same level at stage 3 ().
Plant hormonal changes in Cuscuta
The tZ- and DZ-type CKs in the haustoria-forming part increased at stage 2 and deceased at stage 3, but the absolute amounts were much lower than those in Momordica stems at the same stage (). At the apical part, the concentration peak tended to be at stage 3 (Figure S6).
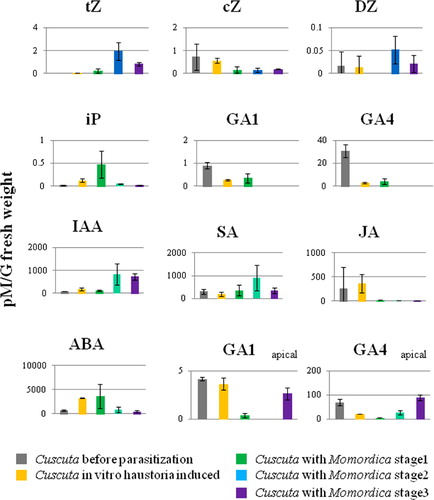
The cZ types of CK were endogenously high in Cuscuta, but tended to decrease after parasitization, especially at stage 2. The iP type CKs increased with haustoria development (). In particular, a transient increase was recorded only in Cuscuta at stage 1.
The amount of GA1 and 4 (active form of GA) dropped after haustoria induction and became non-detectable with subsequent plant interaction. GA1, 4, 9, 12 increased again only at the apical region at stage 3 ().
The IAA free form increased with haustoria development, and a drastic increase concomitantly occurred with parasitization, especially at stage 2 (). A marked increase of many amino acid conjugated forms was recorded at in vitro haustoria-induced seedlings, but tended to decrease after parasitization. In contrast, IAA-Asp remained at higher levels after parasitization (Figure S6).
SA increased at stage 2 and decreased at stage 3 (). In contrast, JA fell rapidly after the interaction with Momordica and remained at this low level ().
Endogenous ABA was 10 times higher than that in Momordica (). ABA was high in stage 1 and in in vitro haustoria-induced Cuscuta seedlings, but decreased at stage 2.
Discussion
Influence of parasitization on host plant growth and photosynthetic activity
In general, Cuscuta parasitization causes damage to host plants, but our present experimental system did not reveal a statistically significant difference in Momordica leaves and stem length growth with and without Cuscuta parasitization. Most likely, potential damage (e.g. loss of water and nutrients) was not serious for Momordica development. Photosynthetic activity in Momordica leaves was also not influenced by parasitization, although values in Momordica stems parasitized by Cuscuta fell with time. As Fv/Fm and Fv′/Fm′ values decreased, the PSII system is probably mainly affected by parasitization. This phenomenon is similar to the chlorophyll reduction in the host plant Zizyphus jujuba due to a stem parasitic plant (Cassytha filiformis) (Abubacker et al. Citation2005). The drop of photosynthetic activity in Cuscuta was Fv/Fm, and influence was also in the PSII system. Probably, Cuscuta does not need to obtain much energy from photosynthesis: it may be able to obtain sufficient nutrients from the host plant within a few days after parasitization. Consistently, stage 2 was the starting point for rapid growth of Cuscuta. Interestingly, scale leaves did not show higher photosynthetic activity than the apical and haustoria parts, although the scale leaves themselves were green.
Morphological change and vascular bundle differentiation in stems
In general, hypertrophy response did not include HR or any serious resistance. While, histological examination showed a vascular bundle increase in Momordica stems. Hypertrophy and increase in the number of vascular bundles in Momordica stems was clearly induced by Cuscuta hypae. No previous reports of vascular tissue differentiation caused by plant–plant interactions (especially induced by Cuscuta hypae) are available, although vascular tissue development of Cuscuta itself has been studied (Christensen et al. Citation2003). Interestingly, we observed similar hypertrophy in interactions between Cuscuta and other members of the Cucurbitaceae family (e.g. Cucumis sativas), to which Momordica also belong (unpublished data). Thus, the phenomenon appears to be related to common characteristics in Cucurbitaceae.
Relatively similar phenomena include gall formation induced by microorganisms (Joshi & Loria Citation2007). Gall-forming bacterial (Agrobactrium tumefaciens, Rodococcus fascians) or fungal (Puccinia striiformis) cytokinin tends to enhance proliferation of host plant tissue. This gall has an irregular shape, although such a phenomenon is also caused by CK changes. For example, Rodococcus fascians produces cZ, tZ, iP, and methyl thio derivatives (Choi et al. Citation2011). In the case of Agrobactrium tumefaciens, CK increases in host plant are provoked by the bacteria origin enzymes (Sakakibara et al. Citation2005). Note, however, that the Momoridca vascular bundle increase appears to reflect an endogenous cytokinins increase, because the absolute concentrations of tZ- and DZ-type CKs were much higher in Momordica than in Cuscuta.
Vascular tissue differentiation has been observed in grafts as well (Mohr & Schopfer Citation1995), and a vascular bundles connection between different plant species is critical for successful graft formation. Research has been done on grafts in Cucurbitaceae. For instance, Golecki et al. (Citation1999) sought P-protein translocation during grafts between Cucumis sativas and Cucurbita maxima. Disruption of the sieve (e.g. by injury) caused the formation of P-protein plag, leading to an accumulation of P-protein filaments at the sieve plates. During vascular tissue differentiation, polymerized P-proteins appear to accumulate, but no correlation with CKs has been reported. Our finding that parasitic plant parasitization induced new vascular tissue in different plant taxa is, in fact, completely different than that practiced in grafts.
Plant hormonal changes in Momordica
Hormonal changes in Momordica are mainly related to hypertrophy and the pathogenic response to Cuscuta. Firstly, all CKs are generally up-regulated in Momordica stems by Cuscuta parasitization, because the absolute amount of CKs was much higher than that in Cuscuta. Generally, plant-derived cytokinin plays important roles for plant resistance and virus infection (Choi et al. 2011). Hence, the increase of CKs in Momordica is considered to be for hypertrophy. Indeed, other host plants which do not show any clear hypertrophy response by Cuscuta parasitization did not increase CKs drastically like Momordica (data not shown).
Loss of nutrients and water by parasitization can be crucial in plant interactions. The increase of ABA in Momordica at stage 2 might reflect drought stress due to the loss of water and nutrients by Cuscuta parasitization. Therefore, Cuscuta indirectly enhanced water uptake in the host plant. Jeschke et al. (Citation1997) reported another interesting phenomenon: they observed that host plant (Coleus blumei) leaf chlorophyll concentration and nitrogen uptake were increased by Cuscuta reflexa parasitization. These phenomena appear to be important parasitic strategies for Cuscuta.
Hormonal control of pathogenic responses has been previously reviewed (Bari & Jones Citation2009; Pieterse et al. Citation2009, Citation2012). SA, JA, and ET are plant defense response hormones. SA is related to the SAR systems for biotrophic or hemi-biotrophic pathogens. The SA level is increased with infection and induces pathogen-related (PR) genes and enhances resistance. JA or ET, in turn, is involved in many herbivorous insects’ attacks or in necrotrophic pathogens, which are a local response to pathogen infection or tissue damage. Only few reports suggested a SA signaling activation by herbivores. One case is the silver whitefly Bemisia tabaci, which activates SA signaling and suppresses JA signaling in Arabidopsis. In principle, both SA and JA pathways are not activated at the same time. With regard to parasitization by parasitic plants, only few reports are available. The root parasite Orobanchae ramose induced JA-dependent genes but not SA-dependent genes in Arabidopsis (Dos Santos et al. Citation2003). As for Cuscuta, only one previous study by Runyon et al. (Citation2010) suggested that both JA and SA responded after Cuscuta pentagona parasitization of tomatoes (Solaum lycopersicum), and the JA peak was prior to SA. In our Cuscuta-Momordica interaction, JA was not changed but SA was increased in Momordica. The pathogenic response in Momoridca was clearly the SA pathway rather than the JA pathway. The SA response took place a few days after parasitization and the increase was transient. The data inferred that the response by Momordica is rather a SA-mediated defense response (against biotrophy rather than necrotrophy). Nevertheless, there was no HR and no clear resistance at Momordica side, indicating that SA increase (as well as other hormonal change) did not lead to final output for defense.
In Momordica stems, IAA-Asp increased after parasitization, but no significant change was recorded in the free form auxin, IAA. In contrast, in the Cuscuta haustoria part, the free form IAA increased and conjugated IAA did not change. Accordingly, Momordica apparently reduces the amount of free form auxin and increases the conjugate form auxin as a response to Cuscucta. This result is similar to the response of GH3-8, which enhances resistance of rice to pathogens (CitationDing et al. 2008). GH3-8 is considered to be independently regulated from SA and JA signaling and accumulates conjugated form of auxin. In studies on rice, GH3-8 overexpression reduced SA or JA responsive gene expression. In Momordica the response to Cuscuta was different. Gonzalez-Lamothe et al. (Citation2012) proposed the model that IAA-Asp, by way of GH3-2, promotes plant disease.
Plant hormonal changes in Cuscuta
The iP type CK up-regulation was evident at stage 1, especially at the haustoria-induced part as well as in in vitro haustoria-induced seedlings. As the Momordica stems did not significantly change the amount of iP type CKs, the increased iP is considered to reflect synthesis in Cuscuta both when the haustoria are formed as well as during invasion into the host plant. Given that the amount of iP type CKs was higher in Cuscuta at stage 1 than that which the haustoria induced in vitro, the role of iP type CKs is not restricted to haustoria formation. In addition, iP type CKs may increase in nutrient-depleted plants, especially when nutrients are added (Takei et al. Citation2004; Rubiom et al. Citation2009). Cuscuta seedlings before parasitization clearly do not have an opportunity obtain any nutrients from the environment, and stage 1 is the first time to acquire nutrients after germination.
With regard to the increase of tZ and DZ from stage 2, this increase seems to be implicated in axillary bud development and vascular bundle formation. Lateral shoot elongation from the axillary bud is related to inhibition by auxin or strigolactone as apical dominance (Beveridge & Kyozuka Citation2009). In our study, auxin did not correlate with this. The absolute amount of these CKs was much higher in Momordica stems. For this reason, it is possible that Cuscuta rather sucked tZ and DZ CKs from the host plant, and the thus obtained CKs caused vascular bundle and axillary bud development in Cuscuta. It is, therefore, currently not possible to know whether these CKs are derived from the host plant or are synthesized by Cuscuta.
The drastic increase in tZ- and DZ-type CKs correlates with the water and inorganic current. For Cuscuta, the upward flow (from the haustoria part to the apex) of water and inorganic elements is desired, because Cuscuta needs to suck water by way of the host plant xylem and transfer it to the apical part. With regard to cZ, the situation is totally opposite to tZ/DZ-type CKs (Werner & Schümlling Citation2009). There is a possibility that cZ increase is related to the sink-source system, so that sugars synthesized in the leaf are transferred to the root via phloem. The direction of movement is from apical to root. In the case of Cuscuta, obtained nutrients or sugars should be moved to the apex from the haustoria, and this is the opposite direction to normal plants with roots. As such, it can imply that Cuscuta take more nutrients with this current direction by decreasing cZ type CKs.
GA changes are implicated in apical growth conditions: the amounts were smaller during haustoria formation and increased when Cuscuta started to elongate for the next parasitization.
The absolute SA amount in Momordica at stage 2 was almost 10 times higher than that in the Cuscuta haustoria part. Consequently, the SA increase in Cuscuta could be simply due to sucking host plant SA. At the same time, plant interactions cause Cuscuta to decrease JA. A likely interpretation is that Cuscuta degrades JA from host plants in order to suppress potential activation of the JA-derived defensive pathway in the host. SA increase and JA decrease was also observed from Cuscuta parasitizing to other host plant which do not show hypertrophy response, therefore it is amenable to recognize such SA and JA change as common important change in Cuscuta as parasitic plant.
The ABA increase was clear at stage 1 but dropped at stage 2. Cuscuta was apparently under drought stress before the parasitization, but drought stress was minimized after sucking enough water and nutrients from the host plant. As such, the ABA change in Cuscuta appears to be correlated with drought stress conditions and water accessibility from host plants.
Concluding remarks
Cuscuta, as a generalist type holostemparasitic plant, interacts with various host plants in different manner. Most host plants of Cuscuta are passive; only few plants showed clear resistance (e.g. Ipomoea sp). The Cuscuta–Momordica plant interaction differed entirely from previously reported plant interactions. Here, we report the new, unique phenomenon that a parasitic plant induced hypertrophy together with vascular tissue differentiation in the host plant stem. Plant hormone analysis clarified that cytokinin played a major role in this process. Momordica hypertrophy response might be derived from resistance, while Cuscuta grow rapidly under the presence of hypertrophy response. Hence, we envisage that hypertrophy response seems to be even beneficial for Cuscuta at a glance, although ecological significance is still not clear. Future investigations should clarify the actual mechanism and the meaning of hypertrophy response.
Supplemental Material.pdf
Download PDF (326 KB)Acknowledgments
The authors thank Dr. Kawade, Dr. Yoshida, and Dr. Myoga (RIKEN Plant Science Center) for advice on the experiments. Dr. Stachowitsch (University of Vienna) improved my English.
Notes
1. Supplemental Content may be viewed online at http://dx.doi.org/10.1080/17429145.2013.816790.
References
- Abubacker MN, Prince M, Hariharan Y. 2005. Histochemical and biochemical studies of parasite–host interaction of Cassytha filiformis Linn and Zizyphus jujuba Lamk. Curr Sci. 89:2156–2159.
- Bari R, Jones JD. 2009. Role of plant hormones in plant defence responses. Plant Mol Biol. 69:473–488. 10.1007/s11103-008-9435-0
- Beveridge CA, Kyozuka J. 2009. New genes in the strigolactone-related shoot branching pathway. Curr Opin Plant Biol. 13:1–6 10.1016/j.cbpa.2009.02.022
- Birschwilks M, Haupt S, Hofius D, Neumann S. 2006. Transfer of phloem-mobile substances from the host plants to the holoparasite Cuscuta sp. J Exp Botany. 57:911–921. 10.1093/jxb/erj076
- Birschwilks M, Sauer N, Scheel D, Neumann S. 2007. Arabidopsis thaliana is a susceptible host plant for the holoparasite Cuscuta spec. Planta. 226:1231–1241. 10.1007/s00425-007-0571-6
- Blum U. 2011. Plant-plant allelopathic interactions. Phenolic acids, cover crops and weed emergence. New York: Springer. p. 1–230.
- Bringmann G, Schlauer J, Rückert M, Wiesen B, Ehrenfeld K, Proksch P, Czygan F C. 1999. Host-derived acetogenins involved in the incompatible parasitic relationship between Cuscuta reflexa (Convolvulaceae) and Ancistrocladus heyneanus (Ancistrocladaceae). Plant Biol. 1:581–584. 10.1111/j.1438-8677.1999.tb00787.x
- Chisholm ST, Coaker G, Day B, Staskawicz BJ. 2006 Host-microbe interactions: shaping the evolution of the plant immune response. Cell. 124:803–814. 10.1016/j.cell.2006.02.008
- Choi J, Choi D, Lee S, Ryu CM, Hwang I. 2011. Cytokinins and plant immunity: old foes or new friends? Trends Plant Sci. 16:388–394. 10.1016/j.tplants.2011.03.003
- Christensen NM, Dörr I, Hansen M, van der Kooij TAW, Schulz A. 2003. Development of Cuscuta species on a partially incompatible host: induction of xylem transfer cells. Protoplasma. 220:131–142. 10.1007/s00709-002-0045-4
- Ding X, Cao Y, Huang L, Zhao J, Xu C, Li X, Wang S. 2008. Activation of the indole-3-acetic acid-amido synthetase GH3-8 suppresses expansin expression and promotes salicylate- and jasmonateindependent basal immunity in rice. Plant Cell. 20:228–240.
- Dodds PN, Rathjen JP. 2010. Plant immunity: towards an integrated view of plant–pathogen interactions. Nature. 11:539–548.
- Dos Santos CV, Delavault P, Letousey P, Thalouarn P. 2003 Identification by suppression subtractive hybridization and expression analysis of Arabidopsis thaliana putative defence genes during Orobanche ramosa infection. Physiol Mol Plant Pathol. 62:297–303. 10.1016/S0885-5765(03)00073-0
- Farah AW. 2007 Resistance of some plant species to field dodder (Cuscuta campestris). Afr Crop Sci Conf Proc. 8:913–917.
- Furuhashi T, Fragner L, Furuhashi K, Valledor L, Sun X, Weckwerth W. 2012. Metabolite changes with induction of Cuscuta Haustorium and translocation from host plants. J Plant Interact. 7:84–93. 10.1080/17429145.2011.603059
- Golecki B, Schulz A, Thompson GA. 1999. Translocation of structural P proteins in the phloem. Plant Cell. 11:127–140.
- Gonzalez-Lamothe R, Oirdi ME, Brisson N, Bouarab K. 2012. The conjugated auxin indole-3-acetic acid–aspartic acid promotes plant disease development. Plant Cell. 24:762–777. 10.1105/tpc.111.095190
- Heide-Jørgensen HS. 2008. Parasitic flowering plants Leiden(the Netherlands): Brill; p. 421.
- Hibberd JM, Jeschke WD. 2001. Solute flux into parasitic plants. J Exp Bot. 52:2043–2049. 10.1093/jexbot/52.363.2043
- Higuchi-Takeuchi M, Ichikawa T, Kondou Y, Matsui K, Hasegawa Y, Kawashima M, Sonoike K, Mori M, Hirokawa H, Matsui M. 2011. Functional analysis of two isoforms of leaf-type Ferredoxin-NADP + -Oxidoreductase in rice using the heterologous expression system of arabidopsis. Plant Physiol. 157:96–108. 10.1104/pp.111.181248
- Huang GT, Ma SL, Bai LP, Zhang L, Ma H, Jia P, Liu J, Zhong M, Guo ZF. 2011. Signal transduction during cold, salt, and drought stresses in plants. Mol Biol Rep. 39:969–987. 10.1007/s11033-011-0823-1
- Jeschke WD, Baig A, Hilpert A. 1997. Sink-stimulated photosynthesis, increased transpiration and increased demand-dependent stimulation of nitrate uptake: nitrogen and carbon relations in the parasitic association Cuscuta reflexa-Coleus blumei. J Exp Bot. 48:915–925. 10.1093/jxb/48.4.915
- Joshi MV, Loria R. 2007. Streptomyces turgidiscabies Possesses a functional cytokinin biosynthetic pathway and produces leafy galls. Mol Plant Microbe Interact. 20:751–758. 10.1094/MPMI-20-7-0751
- Kadioglu A, Terzi R, Saruhan N, Saglam A. 2012. Current advances in the investigation of leaf rolling caused by biotic and abiotic stress factors. Plant Sci. 182:42–48. 10.1016/j.plantsci.2011.01.013
- Kojima M, Kamada-Nobusada T, Komatsu H, Takei K, Kuroha T, Mizutani M, Ashikari M, Ueguchi-Tanaka M, Matsuoka M, Suzuki K, Sakakibara H. 2009. Highly-sensitive and high-throughput analysis of plant hormones using MS-probe modification and liquid chromatography-tandem mass spectrometry: an application for hormone profiling in Oryza sativa. Plant Cell Physiol. 50:1201–1214. 10.1093/pcp/pcp057
- Kojima M, Sakakibara H. 2012. Highly sensitive high-throughput profiling of six phytohormones using MS-probe modification and liquid chromatography–tandem mass spectrometry. Methods Mol Biol. 918:151–164.
- Kuijt J. 1969. The biology of parasitic flowering plants. Berkley: University of California Press.
- LeBlanc M, Kim G, Westwood JH. 2012. RNA trafficking in parasitic plant systems. Front Plant Sci. 3:1–11. 10.3389/fpls.2012.00203
- Löffler C, Czygan FC, Proksch P. 1999. Role of lndole-3-acetic acid in the interaction of the phanerogamic parasite Cuscuta and host plants. Plant Biol. 1:613–617. 10.1111/j.1438-8677.1999.tb00271.x
- Mohr H, Schopfer P. 1995. Plant physiology. Berlin: Springer-Verlag.
- Pieterse CMJ, Leon-Reyes A, Van der Ent S, Van Wees SCM. 2009. Networking by small-molecule hormones in plant immunity. Nat Chem Biol. 5:308–316. 10.1038/nchembio.164
- Pieterse CMJ, Van der Does D, Zamioudis C, Leon-Reyes A, Van Wees SCM. 2012. Hormonal modulation of plant immunity. Annu Rev Cell Dev Biol. 28:1–33. 10.1146/annurev-cellbio-092910-154055
- Rubiom V, Bustos R, Irigoyen ML, Cardona-Lopez X, Rojas-Triana M, Paz-Ares J. 2009. Plant hormones and nutrient signaling. Plant Mol Biol. 69:361–373. 10.1007/s11103-008-9380-y
- Runyon JB, Mescher MC, Felton GW, Morares CMD. 2010. Parasitism by Cuscuta pentagona sequentially induces JA and SA defence pathways in tomato. Plant Cell Environ. 33:290–303. 10.1111/j.1365-3040.2009.02082.x
- Sakakibara H, Kasahara H, Ueda N, Kojima M, Takei K, Hishiyama S, Asami T, Okada K, Kamiya Y, Yamaya T, Yamaguchi S. 2005. Agrobacterium tumefaciens increases cytokinin production in plastids by modifying the biosynthetic pathway in the host plant. PNAS. 102:9972–9977. 10.1073/pnas.0500793102
- Singh A, Singh M. 1997. Incompatibility of Cuscuta Haustoria with the resistant Hosts – Ipomoea batatas L. and Lycopersicon esculentum Mill. J Plant Physiol. 150:592–596. 10.1016/S0176-1617(97)80324-0
- Stewart GR, Press MC. 1990. The physiology and biochemistry of parasitic angiosperms. Annu Rev Plant Physiol Plant Mol Biol. 41:127–51. 10.1146/annurev.pp.41.060190.001015
- Takei K, Ueda N, Aoki K, Kuromori T, Hirayama T, Shinozaki K, Yamaya T, Sakakibara H. 2004. AtIPT3 is a key determinant of nitrate-dependent Cytokinin biosynthesis in Arabidopsis. Plant Cell Physiol. 45:1053–1062. 10.1093/pcp/pch119
- Wanek W, Richter A. 1993. L-Idiol: NAD+5-oxidoreductase in Viscum album: utilization of host-derived sorbitol. Plant Physiol Biochem. 31:205–211.
- Werner M, Uehlein N, Proksch P, Kaldenhoff R. 2001. Characterization of two tomato aquaporins and expression during the imcompatible interaction of tomato with the plant parasite Cuscuta reflexa. Planta. 213:550–555. 10.1007/s004250100533
- Werner T, Schmülling T. 2009. Cytokinin action in plant development. Curr Opin Plant Biol. 12:527–538. 10.1016/j.pbi.2009.07.002
- Yoder JI. 1999. Parasitic plant responses to host plant signals: a model for subterranean plant–plant interactions. Curr Opin Plant Biol. 2:65–70.
- Yoshida S, Shirasu K. 2009. Multiple layers of incompatibility to the parasitic witchweed, Striga hermonthica. New Phytologist. 183:180–189.