Abstract
The effect of stress combinations on plants cannot be extrapolated from the response to each of the applied stressors. Greenhouse experiments were carried out on soil to which copper ions were introduced at four concentrations (0, 150, 400, and 600 mg kg−1). Copper treatments without or with Fusarium infection were established. Both stress factors, applied separately or together inhibited growth with the exception of the lowest Cu concentration, which stimulated growth of healthy plants. Depending on concentration, Cu did not change or increased the activity of root peroxidase and leaf catalase, and decreased ascorbate peroxidase (APO) activity in leaves and roots. Infection increased the activities of the enzymes with exception of root APO. The simultaneous presence of these two stress factors modified their individual effects. Generally, the stress combination aggravated the plant status though an opposite trend was observed in some cases.
1. Introduction
Agricultural plants are subjected to different biotic and abiotic stress factors throughout their life cycle which cause considerable crop losses. Fusarium culmorum is a highly virulent pathogen which causes economically important diseases in cereals, such as foot rot, scab, seedling blight, and head blight. It is one of the most widespread fungal pathogens and causes not only yield decrease but also mycotoxin contamination of the grain (Wagacha & Muthomi Citation2007). Almost all cereal species may be attacked, but wheat is considered the most susceptible. Cu containing compounds are widely used in agricultural practice to combat pests as constituents of many herbicides, insecticides, and fungicides. Copper is an essential micronutrient with a significant metabolic role in plants, but in high doses, it can cause morphological and physiological disorders. Copper toxicity might arise from excessive application of Cu pesticides, as well as around copper smelters and refineries because of industrial pollution (Yruela Citation2009).
The enhanced production of reactive oxygen species (ROS) is a common stress response of plants. Depending on their spatial and temporal regulation, ROS may act as damaging, protective, or signaling factors (Mehdy Citation1994; Mittler Citation2002; Apel & Hirt Citation2004; Edreva Citation2005; Torres et al. Citation2006; De Gara et al. Citation2010). Plant tissues and photosynthetic tissues in particular, produce a significant amount of H2O2, whose concentration is under homeostatic regulation. The greater amount of H2O2 must be metabolized, especially under stress conditions that lead to its higher production. Catalase (CAT), ascorbate peroxidase (APO), and unspecific peroxidase (GPO) are among the enzymes which degrade it (De Gara et al. Citation2010). Generally, the activity of one or more antioxidant enzymes is increased under metal stress and under excess Cu, in particular; however, contradictory effects have been observed. The enzyme reaction depends on enzyme type; plant species, variety, organ and prehistory; stress strength and duration; and environmental conditions (Luna et al. Citation1994; Mazhoudia et al. Citation1997; Zhao et al. Citation2010; Jouili et al. Citation2011). After pathogen recognition, both activation and deactivation of different scavenging systems have also been reported (Mehdy Citation1994; Torres et al. Citation2006).
Plants are subjected to the combined action of various stressors simultaneously. When a stress combination occurs, the strength and/or direction of the response to individual factors might be modified (Alexieva et al. Citation2003; Atkinson & Urwin Citation2012). High, potentially toxic, levels of metal ions might favor or hamper the development of biotic stress. Five different modes of action of metal defense against biotic stress have been proposed (Poschenrieder et al. Citation2011). On the other hand, a pathogen or pest attack may reduce or enhance the susceptibility to an abiotic stress. The stress combination cannot be directly extrapolated from the response of plants to each of the different stress factors applied individually (Mittler Citation2006). Thus, by activating a specific program of gene expression sensitive to the environmental condition encountered, plants respond to multiple stresses differently from how they do to individual stresses (Atkinson & Urwin Citation2012).
Hence, the objective of this study was to compare the effect of elevated soil copper concentration and F. culmorum infection, applied separately or together, on growth and antioxidative enzyme activities of wheat plants.
2. Materials and methods
2.1. Plant material, growth conditions, and stress treatments
Greenhouse experiments were carried out for 2 years. An alluvial-meadow type soil was used (pH 6.6, humus content 3.8%, Cu concentration 73 mg kg−1). Copper ions, as a solution of CuCl2·2H2O, were added to 3 kg sterilized soil in pots and left 20 days for stabilization. Four treatments were applied: no additional Cu (0Cu), 150 mg kg−1 Cu (150Cu), 400 mg kg−1 Cu (400Cu), and 600 mg kg−1 Cu (600Cu). After stabilization, the soil was infected with F. culmorum, preliminary grown on barley grains at 18–22°C. The inoculum was introduced into the soil in a quantity of 4 g kg−1 of soil, left for development at 20–25°C and kept wet. After pathogen's development (10–15 days later), the soil was cropped with wheat (Triticum aestivum L.), cv. Sadovo-1, at 50 seeds per pot. The plants were grown at 20–25°C air temperature and soil moisture was maintained by watering with distilled water until the 5–6 leaf growth stage. For each Cu concentration, treatments without (−Pat) and with Fusarium (+Pat) were set up. Apparently, healthy (for variants −Pat) or diseased (for variants +Pat) plants were selected for further analysis. Plants manifesting browning of the stem base were considered diseased without taking into account the severity of symptoms. Healthy plants grown on soil without additional Cu (0Cu − Pat) were designated as the control.
2.2. Biometrical measurements
For characterizing plant growth, shoot height and fresh weight, and root length and fresh weight were recorded immediately after the harvest. For each treatment, 20 plants (10 plants per year) were measured and the average was calculated. An Index of growth alteration (IGA), parameter characterizing the cumulative changes in the above four growth parameters (Miteva et al. Citation2005) was calculated as:
2.3. Enzyme assays
Samples from 10 plants at the 5th–6th leaf phase per treatment were made by cutting the leaves or roots. The oldest (yellowing) and the youngest (not fully developed) leaves were excluded from the sample. Fresh tissue was homogenized in cold Tris–HCl buffer (0.1 M, pH 8.0 containing 17% sucrose, 2 mM ascorbate, 1 mM EDTA) (1:5 w/v), and with Dowex 1×8 (200–400 mesh) (1:10 w/v). The homogenate was centrifuged at 11,000g for 50 min and the supernatant was used for spectrophotometric determination of enzyme activities and protein content. Peroxidase (EC 1.11.1.7) activity was assayed with guaiacol as the hydrogen donor by measuring the absorbance increase at 470 nm due to the formation of tetraguaiacol (Chance & Maehly Citation1955). CAT (EC 1.11.1.6) activity was determined by following the decrease in H2O2 concentration at 240 nm (Aebi Citation1983). APO (EC 1.11.1.11) activity was measured by following the decrease of ascorbate content at 290 nm (Nakano & Asada Citation1987). Protein was determined with Coomassie Brilliant Blue G 250 by the protein–dye binding method of Bradford (Citation1976) using bovine serum albumin as the standard. The four analyzed replications in each experiment were combined for the final presentation of the results.
2.4. Statistics
Statgraphics Plus (version 2.1) was used in order to discriminate among the means by Fischer's least significant difference procedure. Values, followed by the same letter are not significantly different at the 95% confidence level.
3. Results
3.1. Growth
Growth attributes were recorded twice – at the 2–3 leaf stage and at 5th–6th leaf stage. Differences in growth at the early stage varied usually by not more than ±5% to the control (0Cu − Pat) and, as a rule, were not significant (data not shown). The effects of Cu and Fusarium infection, separately and in combination, were well manifested later ().
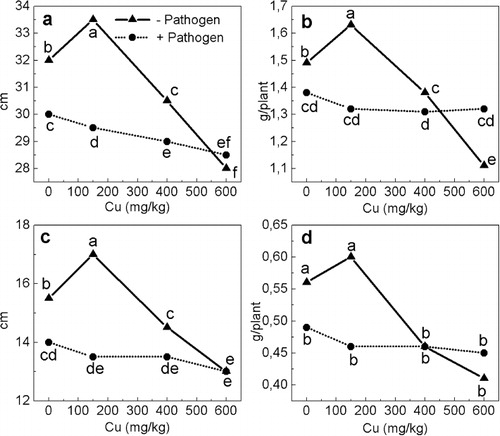
The lowest Cu concentration increased the length and the fresh biomass of shoots and roots by 5–10%. The IGA, reflecting the cumulative effect of a treatment on growth, increased by 8% (). Increasing levels of Cu hampered the growth of healthy plants with growth parameters decreased by 5–18% with 400Cu and 13–27% with 600Cu. IGA indicated a cumulative decrease of 9% and 20%, respectively. An imbalance in plant development might be indicated by assessing the change in RW. The RW for control plants was 47 and 36 mg cm−1 for shoots and roots, respectively. The RW did not change by more than±6% with all individual or combined treatments except for a decrease in healthy plants of 15% for the shoots with 600Cu, and of 12–13% for the roots with 400Cu or 600Cu.
Table 1. Index of growth alteration (%) of wheat plants with excess copper and Fusarium culmorum infection.
Fusarium infection alone decreased growth comparable to treatment with 400Cu (the decreased growth parameters varied between 6% and 12% and IGA = − 9%).
When the infection was combined with excess copper, there was a trend to slightly more growth reduction than with infection alone (0Cu + Pat). The IGA was −13% with both 150Cu and 400Cu, and −15% with 600Cu. The stress combinations compared to the respective separate treatments of Cu excess had different effects, depending on the Cu level. Growth was reduced more when infected plants were grown with 150Cu than with 400Cu because the lowest Cu concentration by itself stimulated growth. At 600Cu, infection did not affect growth negatively, and even provided a slight mitigation of Cu toxicity symptoms. The IGA was −20% with 600Cu − Pat and only −15% with 600Cu + Pat.
3.2. Peroxidase activity
GPO activity in roots increased as much as 10% by both Cu excess and Fusarium infection separately (). This was increased 47% and 31%, respectively, in infected plants supplied with 400Cu and 600Cu. Cu alone did not affect GPO activity in leaves, while Fusarium infection tripled it. Both lower Cu treatments (150Cu and 400Cu) increased additionally GPO activity in infected plants by 26% and 43%, respectively, compared to 0Cu + Pat. No additional increase was observed with 600Cu. Generally, Fusarium increased GPO activity in roots and leaves as compared with healthy plants regardless of Cu level.
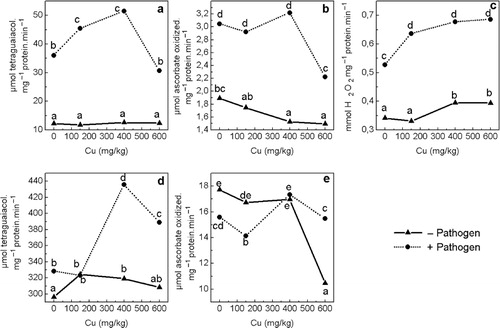
3.3. Ascorbate peroxidase activity
Fusarium infection or the highest Cu concentration decreased APO activity in roots by 12% and 40%, respectively, when applied separately. Different trends were observed in infected plants depending on the Cu level: with 150Cu, APO decreased as with 0Cu; it was similar to healthy plants at 400Cu; while at 600Cu infection raised APO activity by 48%. APO activity in leaves of healthy plants decreased when Cu was added to the soil, the effect being greater at the highest Cu concentration. A similar decrease was observed in diseased plants only with 600Cu. Thus, 600Cu decreased APO by 21% in healthy plants, and by 27% in infected plants compared to the respective 0Cu treatments. Regardless of the Cu concentration, Fusarium infection increased leaf APO activity by 61%, 67%, 111%, and 49% with 0Cu, 150Cu, 400Cu, and 600Cu, respectively.
3.4. Catalase activity
CAT activity was measured only in shoots because the activity in roots was too low to measure. Both Cu and Fusarium infection applied separately increased CAT activity: Cu excess by up to 16% and infection by 54%. The effect of Cu on infected plants was similar, but about 15% greater than observed on healthy plants. In both healthy and infected plants, a concentration dependent trend was observed. The effect of Fusarium on polluted soil was more pronounced, the rise in activity being between 87% and 101%.
4. Discussion
This study imitated common stress situation in the field, namely wheat plants growing on copper polluted soils and infected with one of the most widespread pathogens, F. culmorum. The individual stressors evoked significant changes in plant growth and enzyme activity.
4.1. Separate effect of excess copper
As demonstrated in our previous study, the addition of Cu to the soil increases its mobile form (Bogoeva et al. Citation2007). Bulgarian standards limit the maximum admissible Cu concentration in arable lands with pH 6.0–7.4 to 150 mg/kg. This level had a favorable effect on plant growth of healthy plants in this study. Such beneficial effect induced by low doses of a toxicant, termed hormesis, has been demonstrated since long ago, including for Cu salts (Calabrese & Blain Citation2009). So the 150Cu treatment might be regarded as a mild stimulating stress for healthy plants, i.e. as eu-stress, according to Lichtenthaler (Citation1996). This was not the case with diseased plants as discussed later. Still increased GPO activity in roots indicated an injury there since stress damage at the tissue, ultrastructural or functional level is a prerequisite for peroxidase induction (Edreva Citation1991). Roots, exposed directly to elevated Cu concentrations accumulate it and serve as a first barrier to its transport toward the shoots (Bouazizi et al. Citation2010). As we found earlier the 150Cu treatment brought about a fourfold increase in root Cu content, while the increase in shoots was only 65%. Increasing the Cu concentration in soil, increased its concentration in root and shoot tissues 14 and 5 times, respectively, with the 600Cu treatment as compared to the control (Bogoeva et al. Citation2007). This excess Cu hampered plant growth and caused growth imbalance (more pronounced at 600Cu) because of direct Cu phytotoxicity (e.g. through oxidative damages or by interaction with proteins) or indirectly through disrupting absorption, transport, and functioning of other nutrient elements (Yruela Citation2009). In comparing tissue Cu content in our experiments with that of Bravin et al. (Citation2010) for durum wheat, one may assume that the shoot content at 600Cu (18 mg kg−1) was not toxic; however, at 400Cu the root content (187 mg kg−1) was already rhizotoxic. These authors observed that Cu excess caused abnormal lateral root formation and inhibited root growth. They speculated that the latter might be induced by increased rigidity of the cell wall to seriously impact permeability and nutrient uptake. Cell wall stiffening through enhanced cross-linking of lignin monomers with the contribution of peroxidase might be a structural defense against metal stress to reduce the uncontrolled influx of copper (Bouazizi et al. Citation2010). The changes in root GPO were not concentration dependent, but this has been the case in other studies too (Zhao et al. Citation2010). The unchanged leaf GPO suggested the lack of direct stress damages to aboveground parts, and corresponded to the nontoxic tissue Cu content there. The maintenance of nontoxic ROS concentrations in leaves might have been ensured by other components of antioxidant plant defense such as CAT, whose activity increased. Shoot growth was probably hindered by disrupted nutrient supply due to inhibited root growth (Kopittke & Menzies Citation2006).
Elevated Cu concentrations, especially the higher ones, increased leaf CAT activity, and decreased APO in both shoots and roots. The different enzyme reactions might be explained by their specific physiological roles. The reduction of H2O2 to water is regarded as a secondary function of GPO in plants, while its main role is related to the oxidation of diverse substrates, thus playing a central role in controlling the cell wall biogenesis and in regulating its function, as well as in controlling the hormonal metabolism (De Gara et al. Citation2010; Jouili et al. Citation2011). On the other hand, the main physiological role of the two other enzymes evaluated in this study is supposed to be the elimination of excess H2O2. Because of very high catalytic turnover, CAT can quickly remove a large amount of H2O2, while APO has a much higher affinity for H2O2 and is responsible for fine regulation of its content (De Gara et al. Citation2010). An inverse effect of excess Cu on these enzymes was observed in the present study. It is unlikely that the decrease in APO was due to enzyme inactivation as supposed by Luna et al. (Citation1994) because there was higher activity in diseased plants in combination treatments. Thus, while increased CAT might act as an effective scavenger of excess H2O2, the lower APO could be biologically significant by leaving a low concentration of H2O2 available for regulatory or other physiological functions (De Gara et al. Citation2010). Such enzyme reactions have been observed by other authors (Mazhoudia et al. Citation1997).
4.2. Separate effect of Fusarium infection
Fusarium infection alone decreased growth and root APO and GPO activities comparable to treatment with 400Cu. In contrast to roots and to the changes in shoots caused by excess Cu, Fusarium induced a much larger increase of all three enzymes in leaves. Fusarium tended to induce opposite changes in APO activity in both organs (a decrease in roots and increase in leaves).
In this study, F. culmorum was supplied through the soil so roots were the initial place of infection. A ROS burst and additional cell walls strengthening at the site of attack are well-documented phenomena and are regarded as a plant's attempt to limit the pathogen penetration (De Gara et al. Citation2003; Apel & Hirt Citation2004; Torres et al. Citation2006). Since APO takes part in the former, and GPO in the latter, one might consider decreased APO and increased GPO in roots as a plant reaction to limit further infection. Desmond et al. (Citation2008) reported experimental evidences to support the hypothesis that the mycotoxin elicits programmed cell death in wheat, at least partially mediated by ROS. Cell wall reinforcement and lignification, achieved by peroxidases and polyphenol oxidases are one step of the sophisticated plant response to restrict the influx of Fusarium toxins and the efflux of plant nutrients during the development of the head blight disease (Walter et al. Citation2010). Caruso et al. (Citation1999) attributed the increase in GPO activity in F. culmorum inoculated wheat seedlings to the induction of a neutral peroxidase isoform. It is possible that changes in APO and GPO might have been much greater at specific sites of pathogen penetration than recorded in this study as the root system was analyzed as a whole.
Obviously, the plant reaction to arrest fungal development was insufficient since diseased plants with well manifested foot rot were investigated. Aleandri et al. (Citation2008) propose that increased GPO activity measured under optimum conditions might be lowered by the pathogen by increasing the pH in the apoplast beyond the enzyme optimum. Thus, the capacity of the host to oppose the pathogen might be inhibited. There are indications that both intracellular fungal growth as well as the release of its mycotoxin may cause oxidative stress, which the plant attempts to decrease by detoxification of ROS. At the same time, an increase in cell wall thickness and antifungal properties also occur to fortify the plant's barriers (Walter et al. Citation2010). One might speculate that this occurred in the leaves in the present study: a rise in the activities of all three antioxidative enzymes as an attempt to control the ROS excess and to strengthen cell walls. De Gara et al. (Citation2003) also noted that leaf cells that were unable to stop pathogen penetration had increased APO to limit propagation of the oxidative process. Thus, while roots, as a site of infection try, though unsuccessfully, to limit pathogen penetration, leaves have to cope with a ROS surplus caused by fungal toxins and/or fungal spread. Infection creates a greater stress in leaves than a Cu excess, which, as already mentioned, most likely had not reached the toxicity threshold. This might explain the larger increase of enzyme activities and the opposite changes in APO (increased by infection and decreased by Cu) in leaves.
4.3. Combined effect of excess Cu and Fusarium infection
In our previous study, excess copper reduced the percentage of F. culmorum diseased plants from 77% without additional Cu to 48%, 41%, and 30% with 150Cu, 400Cu, and 600Cu, respectively (Bogoeva & Karadjova Citation2005). This reduction in disease might be attributed to the well-known fungistatic effect of Cu in directly killing or inhibiting pathogen growth outside the plant; however, metal-induced induction of defense responses in plants either directly or through metal-induced ROS should also not be ruled out (Poschenrieder et al. Citation2011).
The present study showed that the simultaneous application of both infection and excess Cu availability modified the effect of each of them on the plant. The manifestation of typical of Fusarium infection alterations varied depending on soil Cu concentrations. The combined treatments tended to decrease slightly more the growth if compared to 0Cu + Pat. This suggests a more severe disease development under Cu excess. In most cases, excess Cu did not shift the direction of enzyme activity associated with infection, though the degree of alterations might have been modified. With one exception (the leaf APO at the 600Cu + Pat), irrespective of Cu concentration the activity of all three enzymes increased in diseased leaves similar to infection alone. The GPO and CAT further increased under the combination when compared to 0Cu + Pat, with the only exception being the GPO once more at 600Cu +Pat treatment. Although Fusarium did not increase additionally root GPO at 150Cu (which was the highest among healthy plants), infection increased GPO at the two higher Cu concentrations. Weakened plant defenses and increased susceptibility to pests and pathogens after prolonged exposure to abiotic stress conditions such as high metal levels, has been often observed (Miteva et al. Citation2005; Mittler Citation2006; Atkinson & Urwin Citation2012). A plant's response may vary depending on metal level and place of pathogen penetration (Maneva et al. Citation2009). According to Spann and Schumann (Citation2010), unoptimal nutrient supply might increase disease susceptibility by affecting the formation of mechanical barriers or the synthesis of natural defense compounds so that plants with an optimal nutritional status usually have the highest resistance to diseases.
Conversely, protection against Fusarium and other fungal pathogens by metals has also been found. Pepper plants stressed by high Cu showed fewer symptoms of Verticillium wilt, probably because the defense reactions induced by Cu such as a higher peroxidase activity, an increase in soluble phenolics, and activated defense-related genes cope not only with the toxic metal but also with the pathogen (Chmielowska et al. Citation2010). Reduced susceptibility to Fusarium infection has been found for wheat plants pretreated with a mild dose of Cd (Mittra et al. Citation2004) and for Indian mustard plants containing Se (Hanson et al. Citation2003). The effect of Cd has been attributed to the protective role of a high molecular mass protein that was produced in response to Cd pretreatment, while Se in leaves was thought to be directly toxic to fungal growth. When explaining the prevention of Fusarium infection in Cajanus cajan seedlings by presoaking the seeds with Al, Satapathy et al. (Citation2012) emphasized the importance of controlled modulation of endogenous ROS levels through up- or downregulation of antioxidant enzymes. In our study, some peculiarities in enzyme activities were observed in diseased plants at the highest Cu concentration (600Cu + Pat treatment). The lack of increased growth makes the use of the term ‘protection’ inappropriate in this case even though the effect of Fusarium on leaf and root GPO, and leaf APO was less pronounced than the effect at 400Cu. That is to say that excess Cu did not increase damage by the pathogen, as discussed above, and values, closer (root GPO) or equal to 0Cu + Pat (leaf GPO) or even to the control (leaf APO) were observed.
Healthy and diseased plants occasionally differed in their reactions to Cu excess. In fact, the 600Cu + Pat treatment reduced plant growth less than 600Cu alone. The effect was most pronounced with regards to shoot biomass. The opposite effects of each separate stressor on leaf APO offset each other when combined and resulted in values close to the control. Even though the two separate stress factors decreased APO in roots (600Cu stronger than +Pat), at 600Cu + Pat the activity remained at the level of the lighter stress. Yet, GPO and CAT activities remained high with the combined treatment. Their levels were equal to or higher than the greater level caused by the separate stress factors. The 21% decrease in root Cu in diseased plants (Bogoeva et al. Citation2007) might give a plausible explanation of how infection decreased toxicity of 600Cu. This could be explained since F. culmorum is a root rotting pathogen, so there would be less healthy root absorptive tissue to assimilate Cu with infection.
In contrast to its effect in healthy plants, 150Cu did not stimulate growth of diseased plants (even an opposite trend was observed), and GPO and CAT activities in leaves were enhanced only in diseased plants. Thus, infected plants were more susceptible to 150Cu than healthy plants. Kafel et al. (Citation2010) reported that aphides infestation of Philadelphus coronarius plants cultivated on polluted soil affected both the contamination of plants with metals and their antioxidant response. In particular, infestation increased leaf Cu and decreased GPO, but had no effect on CAT. The 14% increase in leaf Cu with Fusarium infection (Bogoeva et al. Citation2007) could not explain their greater susceptibility to 150Cu, as the Cu concentration remained far below the values with the higher Cu treatments and below the toxicity levels. Pathogen infection might have altered the plant's physiology by disturbing nutrient uptake, assimilation, translocation, and utilization of other nutrients (Spann & Schumann Citation2010). Discussing the results in light of stress concept might imply that the stress-coping mechanisms and the capacity of damage repair had been exhausted by infection, thus rendering the plant susceptible to the additional stress (Lichtenthaler Citation1996; Alexieva et al. Citation2003).
Metal toxicity and biotic stress share many common signaling molecules and can interact at the metabolic level. Our research shows that they are interrelated physiologically. However, many differences between both stresses exist, including ROS production and detoxification (Mittler Citation2002; Apel and Hirt Citation2004). The interaction between stresses is controlled by hormones and by a number of molecular mechanisms, which as a whole act as a complex regulatory network (see for review, Atkinson & Urwin Citation2012). The modes of signal transduction are highly stress specific due to specificity of receptors, subcellular compartmentalization, differences in activated genes, etc. so that broad co-resistance among and between different biotic and ion toxicity stresses would not be possible (Poschenrieder et al. Citation2011).
In summary, excess Cu and F. culmorum infection change plant growth and the activities of peroxidase, CAT, and APO. The simultaneous occurrence of both stress factors modified their separate effects. Generally, a combination of stresses aggravated the plant status though an opposite trend was observed in some cases. A parallel response in plant growth and enzymes activities was not always the rule.
Acknowledgments
The authors thank the phytopathologist Mrs Elena Boncheva for her scientific assistance with Fusarium infection, Mrs Ana Trifonova for excellent technical support, Prof. Aglika Edreva for reviewing the manuscript and giving valuable suggestions and anonymous reviewer for scrutinizing the text in order to improve its readability.
References
- Aebi H. 1983. Catalase. In: Bergmeyer H, editor, Methods of enzymatic analysis. 3rd ed. Weinhein: Verlag Chemie; p. 273–286.
- Aleandri MP, Magro P, Chilosi G. 2008. Influence of environmental pH modulation on efficiency of apoplastic PR proteins during Fusarium culmorum – wheat seedling interaction. Plant Pathol. 57:1017–1025. 10.1111/j.1365-3059.2008.01859.x
- Alexieva V, Ivanov S, Sergiev I, Karanov E. 2003. Interaction between stresses. Bulg J Plant Physiol. XXIX:1–17.
- Apel K, Hirt H. 2004. Reactive oxygen species: metabolism, oxidative stress, and signal transduction. Ann Rev Plant Biol. 55:373–399. 10.1146/annurev.arplant.55.031903.141701
- Atkinson N, Urwin P. 2012. The interaction of plant biotic and abiotic stresses: from genes to the field. J Exp Bot. 63:3523–3544. 10.1093/jxb/ers100
- Bogoeva I, Boncheva E, Stefanova R, Blajev B. 2007. Influence of soil copper pollution and Fusarium culmorum on the heavy metal content in wheat. Bulg J of Agric Sci. 13:333–339.
- Bogoeva I, Karadjova J. 2005. Influence of copper ions on Fusarium pathogens in wheat. In: Stara Zagora Union of Scientists, editor. Proceedings of the International Science conference; 2005 Jun 2–3; Stara Zagora, Bulgaria, v.2 Plant-growing. 198–201 ( in Bulgarian).
- Bouazizi H, Jouili H, Geitmann A, Ferjani E. 2010. Structural changes of cell wall and lignifying enzymes modulations in bean roots in response to copper stress. Biol Trace Elem Res. 136:232–240. 10.1007/s12011-009-8530-7
- Bradford M. 1976. A rapid and sensitive method for the quantitation of microgram quantities of protein utilizing the principle of protein-dye binding. Analyt Biochem. 72:248–254. 10.1016/0003-2697(76)90527-3
- Bravin M, Le Merrer B, Denaix L, Schneider A, Hinsinger P. 2010. Copper uptake kinetics in hydroponically- grown durum wheat (Triticum turgidum durum L.) as compared with soil's ability to supply copper. Plant Soil. 331:91–104. 10.1007/s11104-009-0235-3
- Calabrese EJ, Blain RB. 2009. Hormesis and plant biology. Env Pollution. 157:42–48. 10.1016/j.envpol.2008.07.028
- Caruso C, Chilosi G, Caporale C, Leonardi L, Bertini L, Magro P, Buonocore V. 1999. Induction of pathogenesis-related proteins in germinating wheat seeds infected with Fusarium culmorum. Plant Sci. 140:87–97. 10.1016/S0168-9452(98)00199-X
- Chance B, Maehly A. 1955. Assay of catalases and peroxidases. In: Colowik SP, Kaplan NO, editors. Methods in enzymology, Vol. 2, New York: Academic Press; p. 764–781.
- Chmielowska J, Veloso J, Gutiérrez J, Silvar C, Díaz J. 2010. Cross-protection of pepper plants stressed by copper against a vascular pathogen is accompanied by the induction of a defense response. Plant Sci. 178:176–182. 10.1016/j.plantsci.2009.11.007
- De Gara L, de Pinto M, Tommasi F. 2003. The antioxidants systems vis-à-vis reactive oxygen species during plant-pathogen interaction. Plant Physiol Biochem. 41:863–870. 10.1016/S0981-9428(03)00135-9
- De Gara L, Locato V, Dipierro S, de Pinto M. 2010. Redox homeostasis in plants. The challenge of living with endogenous oxygen production. Respir Physiol Neurobiol. 173:S13–S19. 10.1016/j.resp.2010.02.007
- Desmond OJ, Manners JM, Stephens AE, Maclean DJ, Schenk PM, Gardiner DM, Munn AL, Kazan K. 2008. The Fusarium mycotoxyin deoxynivalenol elicits hydrogen peroxide production, programmed cell death and defence responses in wheat. Mol Plant Pathol. 9:435–445. 10.1111/j.1364-3703.2008.00475.x
- Edreva A. 1991. The tissue, ultrastructural or functional damage – a prerequisite for peroxidase induction. In: Lobarzewski J, Greppin H, Penel C, Gaspar T, editors. Biochemical, molecular and physiological aspects of plant peroxidases. Switzerland: University of Geneva; p. 387–390.
- Edreva A. 2005. Generation and scavenging of reactive oxygen species in chloroplasts: a submolecular approach. Agric Ecosyst Environ. 106:119–133. 10.1016/j.agee.2004.10.022
- Hanson B, Garifullina GF, Lindblom SD, Wangeline A, Ackley A, Kramer K, Norton AP, Lawrence CB, Pilon-Smits EAH. 2003. Selenium accumulation protects Brassica juncea from invertebrate herbivory and fungal infection. New Phytol. 159:461–469. 10.1046/j.1469-8137.2003.00786.x
- Jouili H, Bouazizi H, Ferjani EE. 2011. Plant peroxidases: biomarkers of metallic stress (Review). Acta Physiol Plant. 33:2075–2082. 10.1007/s11738-011-0780-2
- Kafel A, Nadgórska-Socha A, Gospodarek J, Babczyńska A, Skowronek M, Kandziora M, Rozpedek K. 2010. The effects of Aphis fabae infestation on the antioxidant response and heavy metal content in field grown Philadelphus coronarius plants. Sci Total Environ. 408:1111–1119. 10.1016/j.scitotenv.2009.11.013
- Kopittke P, Menzies N. 2006. Effect of Cu toxicity on growth of cowpea (Vigna unguiculata). Plant Soil. 279:287–296. 10.1007/s11104-005-1578-z
- Lichtenthaler H. 1996. Vegetation stress: an introduction to the stress concept in plants. J Plant Physiol. 148:4–14. 10.1016/S0176-1617(96)80287-2
- Luna C, Gonzalez C, Trippi V. 1994. Oxidative damage caused by an excess of copper in oat leaves. Plant Cell Physiol. 35:11–15.
- Maneva S, Bogatzevska N, Miteva E. 2009. Excess copper in soil as a factor affecting bacterial spots caused by Xanthomonas vesicatoria in tomato plants; bio-interaction between two stress factors and their influence on plants. Acta Physiol Plant. 31:125–131. 10.1007/s11738-008-0210-2
- Mazhoudia S, Chaoui A, Ghorbalb MH, El Ferjani E. 1997. Response of antioxidant enzymes to excess copper in tomato (Lycopersicon esculentum, Mill.). Plant Sci. 127:129–137. 10.1016/S0168-9452(97)00116-7
- Mehdy M. 1994. Active oxygen species in plant defense against pathogens. Plant Physiol. 105:467–472.
- Miteva E, Hristova D, Nenova V, Maneva S. 2005. Arsenic as a factor affecting virus infection in tomato plants: changes in plant growth, peroxidase activity and chloroplast pigments. Sci Hort. 105:343–358. 10.1016/j.scienta.2005.01.026
- Mittler R. 2002. Oxidative stress, antioxidants and stress tolerance. Trends Plant Sci. 7:405–410. 10.1016/S1360-1385(02)02312-9
- Mittler R. 2006. Abiotic stress, the field environment and stress combination. Trends Plant Sci. 11:15–19. 10.1016/j.tplants.2005.11.002
- Mittra B, Ghosh P, Henry SL, Mishra J, Das TK, Ghosh S, Babu C, Mohanty P. 2004. Novel model of resistance to Fusarium infection by a mild dose pre-exposure of cadmium in wheat. Plant Physiol Biochem. 42:781–787. 10.1016/j.plaphy.2004.09.005
- Nakano Y, Asada K. 1987. Purification of ascorbate peroxidase in spinach chloroplasts; Its inactivation in ascorbate-depleted medium and reactivation by monodehydroascorbate. Plant Cell Physiol. 28:131–140.
- Poschenrieder C, Cabot C, Tolrà R, Llugany M, Martos S, Gallego B, Barceló J. 2011. Metal defense against biotic stress; is it real? Agrochimica. 55:29–44.
- Satapathy P, Achary VMM, Panda BB. 2012. Aluminum-induced abiotic stress counteracts Fusarium infection in Cajanus cajan(L.) Millsp. J Plant Interact. 7:121–128. 10.1080/17429145.2011.584133
- Spann T, Schumann A. 2010. Mineral nutrition contributes to plant disease and pest resistance (HS 1181). Gainesville: University of Florida Institute of Food and Agricultural Sciences. [Accessed 18 July 2012]. Available from: http://edis.ifas.ufl.edu/hs1181.
- Torres MA, Jones J, Dangl J. 2006. Reactive oxygen species signaling in response to pathogens. Plant Physiol. 141:373–378. 10.1104/pp.106.079467
- Wagacha JM, Muthomi JW. 2007. Fusarium culmorum: infection process, mechanisms of mycotoxin production and their role in pathogenesis in wheat. Crop Protect. 26:877–885. 10.1016/j.cropro.2006.09.003
- Walter S, Nicholson P, Doohan F. 2010. Action and reaction of host and pathogen during Fusarium had blight disease. New Phytol. 185:54–66. 10.1111/j.1469-8137.2009.03041.x
- Yruela I. 2009. Copper in plants: acquisition, transport and interactions. Functional Plant Biol. 36:409–430. 10.1071/FP08288
- Zhao S, Liu Q, Qi Y, Duo L. 2010. Responses of root growth and protective enzymes to copper stress in tufgrass. Acta Biol Cracov Bot. 52:7–11.