Abstract
During ectomycorrhizal (ECM) establishment, biochemical signals lead to the development of complex structures in both the plant and the fungus that ultimately result in the formation of an ectomycorrhiza. The cross-talk between partners begins before physical contact. Our objective was to investigate the chemical nature of the signals during the first stages of in vitro mycorrhization of Pinus pinea with Pisolithus arhizus. For this purpose a double-phase solid–liquid medium was expressly developed for the co-culture in order to simplify the extraction and further molecules analysis. O-coumaric acid ester was identified using HPLC–UV and LC–DAD–MS on the second day of co-culture and its presence was detected for up to 10 days. These results contribute to the characterization of biochemical signals during pre-colonization involving conifer species and an ECM fungus, and demonstrate the suitability of the double-phase medium developed for the growth of both organisms and for the analysis of released chemical mediators.
Introduction
It is well known that root exudates may act as messengers that communicate and initiate biological and physical interactions between roots and soil organisms (Walker et al. Citation2003). The compounds secreted by plant roots can act as chemical attractants or repellants in the narrow zone of soil immediately surrounding the root system (Estabrook & Yoder Citation1998; Bais et al. Citation2001; Walker et al. Citation2003). The successful colonization of roots by mycorrhizal fungi and further development of the ectomycorrhizal (ECM) structures result from a series of events mediated by several root and fungal biochemical signals (Seddas et al. Citation2009). In a recent review by Bonfante and Genre (Citation2010), the identification of several novel nutrient transporters has revealed some cellular processes that underlie symbiosis, but the biochemical signals prior to physical contact and their functions still need to be elucidated, especially for ECM fungi.
Among various compounds, root flavonoids have been demonstrated to play an important role in the root–microbe interaction. Compounds like p-coumaric acid, coumarin, naringenin, and other flavonoids were cited as potential signaling molecules during mycorrhizal formation (Lynn & Chang Citation1990; Mandal et al. Citation2010; Amalesh et al. Citation2011; Hassan & Mathesius Citation2012; Plett & Martin Citation2012). According to Walker et al. (Citation2003), there is evidence that flavonoids are involved in the vesicular-arbuscular mycorrhiza (AM) colonization. It has been shown that flavonoids are also associated with hyphae growth of ECM fungi. For example, Lagrange et al. (Citation2001) isolated rutin from Eucalyptus globulus ssp bicostata roots and demonstrated the promoting effect of rutin on the hyphae growth of two strains of the ECM fungus Pisolithus tinctorius. Therefore, the evidence of the involvement of phenolic compounds in the plant–fungus interaction has been well established and their list continues to expand.
Recently, we demonstrated that in vitro co-culture of Pinus pinea plantlets with Pisolithus arhizus helped to overcome the cessation of adventitious root growth and the development of a better root system compared with the nonmycorrhizal plants (Ragonezi et al. Citation2012). The positive effect was observed prior to the physical contact between pine roots and the fungus which was inoculated on the surface of a double-phase solid–liquid medium. The objective of this study was to elucidate the chemical nature of the mediators and to determine the time during which the signaling responsible for the mycorrhization between P. arhizus and roots of P. pinea began. The liquid medium surrounding the roots was extracted and analyzed by high-performance liquid chromatography with UV detection (HPLC–UV) and with diode array and mass spectrometry (LC–DAD–MS).
Materials and methods
Plant material and micropropagation of P. pinea
Seeds from selected P. pinea adult trees were obtained in March 2009 (Alcácer do Sal region, Portugal) and stored in a cold chamber at 4°C until used. Microshoot regeneration was carried out according to Oliveira et al. (Citation2003). For rooting, elongated microshoots, ±2 cm long, were transferred to a rooting medium based on Woody Plant Medium (WPM) (Lloyd & McCown Citation1981) with half concentration of the macronutrients, 0.65% agar (Difco Bacto-Agar®), and different carbon sources (see below), and adjusted to pH 5.8 before autoclaving. For root induction, the medium was supplemented with 10.7 µM naphthalene acetic acid (NAA) and 0.12 M glucose (WPMRI). The cultures were kept for two weeks in a growth chamber at a constant temperature of 19°C. For the first week the cultures were placed in darkness and for the second week under a 16-h photoperiod. Root expression was obtained after transferring the microshoots to WPM consisting of half concentration of the macronutrients, without plant growth regulators and with 0.058 M glucose (WPMRE) and incubated in a growth chamber at 16-h photoperiod with cool white fluorescent lamps at 90 µmol m−2 s−1 of photosynthetic photon flux density (PPFD) and 25/19°C day/night temperatures. Roots appeared after 3–6 weeks.
Fungi purification and identification
Collection of P. arhizus fruiting bodies and molecular identification of pure isolate were described by Ragonezi et al. (Citation2012). P. arhizus nucleotide sequence has been deposited in the GenBank (NCBI) nucleotide sequence database under the accession number HQ896485.
Co-culture technique
After the root induction and expression phases, the plantlets were transferred to the double-phase medium. All medium components, perlite (Europerl®), WPM with 6 g/l gellan gum (Phytagel™, Sigma-Aldrich®) (WPMS – solid phase), and WPM without a gelling agent nor a carbohydrate (WPML – liquid phase), were first sterilized and prepared in a horizontal laminar flow chamber as follows: (1) 15 mL of molten WPMS was poured into each vessel together with 0.9 g of perlite. After the medium solidified, the perlite remained at the top and together formed the ‘solid phase’ medium layer which was then flipped over inside the vessel with a sterile metal spatula. (2) 30 mL of WPML were poured into the culture vessel forcing the ‘solid phase’ medium to float on its surface due to the perlite. In consequence, the upper part of the ‘solid phase’ medium had no physical contact with the liquid medium (Castro et al. Citation2012, Patent INPI No. 105239, ).
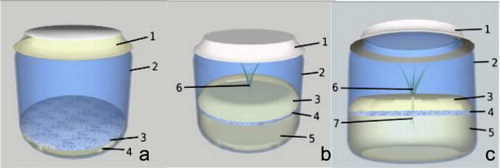
P. pinea plantlets were inoculated with pure cultures of P. arhizus and transferred to growth chamber with the same conditions as root expression phase (a). The cultures were kept under cool white lamps (Philips Master LD36W/840) with a first 90 µmol m−2 s−1 of PPFD. The development of plants and mycelia was monitored daily.
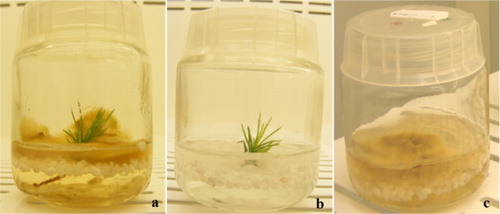
Identical double-phase medium and culture conditions were used for the two types of negative controls: pine plantlets without fungal inoculation (b) and mycelium culture without pine plantlets (c).
Biochemical analysis
Collection of liquid medium samples
Tewnty five milliliters of the liquid phase were collected from each flask containing inoculated plants (from double-phase liquid medium) and from the two negative controls. The samples were frozen separatedly in centrifuge tubes (Falcon™ 50 mL) at −20°C. The sampling was done on days 1, 2, 3, 5, and 10 of the culture. The frozen samples were lyophilized and resuspended in 3 mL of ultra-pure water (resistivity ≥18 M cm) from a Millipore Milli-Q system (Simplicity® UV, Millipore Corp., France): methanol (40:60 v/v VWR® Carnaxide, Portugal) solution. The liquid was filtered through a nylon syringe filter (0.45 µm, VWR® Carnaxide, Portugal), poured into HPLC–UV/LC–DAD–MS vials (VWR® Carnaxide, Portugal), and frozen again at −20°C. For injection into HPLC–UV, the samples were thawed on ice in the dark.
First, tests to detect chemical compounds that are commonly found in the rhizosphere of mycorrhizal plants, called target substances, were carried out. The preparation of standard solutions (SSs) of the target substances such as indole-3-acetic acid (IAA), rutin, and indole-3-butyric acid (IBA) was done as follows: 1 mg of the tested substance was dissolved in 10 mL of methanol corresponding to a final concentration of 100 mg L−1. The solutions were then diluted in a ratio of 1:10 (also in methanol) to provide work solutions (WS) for injections. Sample spiking was performed using the SS of the target substances. The SS was diluted in the liquid phase of the sample: 1 mL of the SS and 9 mL of the sample. The mixture was filtered through a nylon syringe filter (0.45 µm) into HPLC–UV/LC–DAD–MS vial and frozen at −20°C. For injection into HPLC–UV, the samples were thawed on ice in the dark.
Analysis by HPLC–UV and LC–DAD–MS
All samples were analyzed in an HPLC–UV system ELITE LaChrom VWR HITACHI equipped with a VWR HITACHI L-2100 pump, a Rheodyne injector and a VWR® HITACHI L-2400 UV detector. The reversed-phase analytical column was a Supersher 100 Merck® RP-18 (250×4.6 mm, 5 µm) with a pre column LiChrospher 100 RP-18. The data acquisition and automatic processing were performed using an EzChom Elite Software. The separation was achieved in the isocratic mode, with a mobile phase composed of 60% methanol, 1% acetic acid (VWR® Carnaxide, Portugal), and 39% ultra-pure water, at a flow rate of 0.7 mL min−1. All analyses were performed at room temperature, for 45 min, the injection volume was 20 μL, and the chromatographic profile was recorded at 210 nm.
LC–DAD–MS analyses were carried out in a LCQ Advantage Thermo Finnigan mass spectrometer equipped with an electrospray ionization source and using an ion trap mass analyzer. The conditions of analysis were: capillary temperature 300°C; source voltage 4.5 kV, source current 100 µA, capillary voltage −45 V in negative ion mode, and collision energy of 35% in the MS n analysis. The mass spectrometer equipment was coupled to an HPLC system with an autosampler (Surveyor Thermo Finnigan) and a diode array detector (DAD). The analytical column was a reversed-phase Zorbax Eclipse XDB (C18, particle size 3.0 µm, 150 mm×2.1 mm). The chromatographic separation was performed with mobile phase at a flow rate of 0.2 mL min−1, by injecting 20 µL of each sample and the elution program was similar to the one used in the HPLC–UV analysis. The DAD detector was scanned from 200 to 500 nm, and the chromatographic profile was recorded at 210 nm.
Results
HPLC–UV and LC–DAD–MS analysis
The HPLC–UV analysis of the liquid-phase medium from the co-culture of P. arhizus and stone pine plantlets showed that with the exception of the day 1 sample, in all other samples a clear peak was recognized. For example, the chromatograms of the day 2 samples showed a peak at retention time (Rt) 11.50 min, tentatively named unknown compound (UC) (a). However, there was no corresponding peak in the negative control (stone pine culture without the fungus) (b).
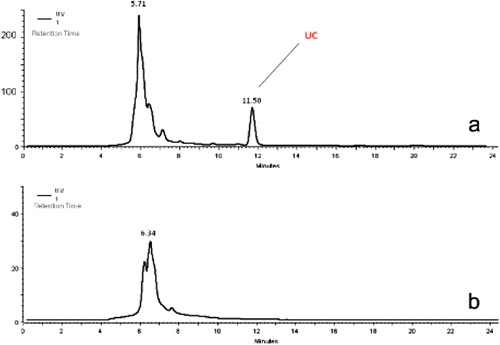
To determine the identity of the UC, the SSs of the target substances such as IAA, rutin, and IBA, were analyzed. When the day 2 sample was spiked with these target compounds, the Rt of UC did not correspond to any Rts of those compounds (a–c).
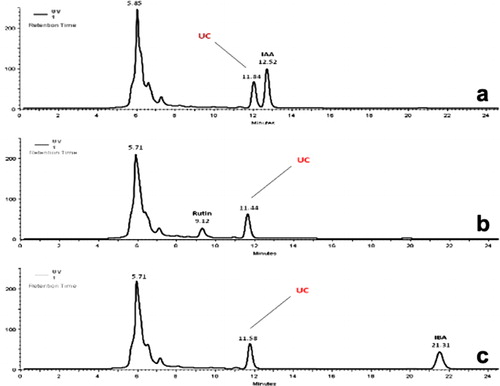
The results of the LC–DAD–MS were consistent with those obtained by HPLC–UV. From day 2 to 10, in all samples the presence of UC was confirmed.
The day 2 sample that was used to identify the UC by LC–DAD–MS showed the DAD total scan (a) and the total ion current chromatograms (b) with Rt of 7.85 and 8.04 min, respectively. The UV spectrum of the UC compound, obtained online by the DAD detector, showed two wavelengths maxima: at 222 and 276 nm (the former being the most intense) (a). The full MS spectra showed an ion signal at m/z 321 with abundance of 100% corresponding to the molecular [M-H]− parent ion, and at m/z 145, one peak that may be assigned to some fragmentation of the m/z 321 ion, corresponding to a benzoyl fragment characteristic of benzoic acid derivatives due to the loss of OH or OR groups (b). The MS2 (b) spectrum of the parent ion at m/z 321 shows four peaks at m/z 321, 163, 145, and 119 that can be attributed to the molecular ion, the benzoyl fragment, the coumaric acid molecular ion, and the loss of CO2 by the coumaric acid ion, respectively. In the MS3 spectrum of the m/z 163 ion (coumaric acid molecular ion), no peaks were identified. The absence of peaks in the MS3 spectrum can be attributed to the initial low concentration of the UC compound, which can make difficult the isolation of the target ion by the ion trap in the MS3 analysis. The UC was identified as an ester of o-coumaric acid. The chemical structure of this compound and its identification were based on the fragmentation pattern and UV spectra that were similar to those reported by Atoui et al. (Citation2005) (b). Additionally, the MS and UV spectra of the coumaric acid fragment are consistent with those reported by Plazonić et al. (Citation2009), for the o-coumaric and p-coumaric acids. In this study, several flavonoids and phenolic acids were identified in Burr Parsley using similar conditions (HPLC–DAD–MS with electrospray ionization and an ion trap analyzer in negative mode). The UV maxima obtained for the two isomers were different, 275 and 310 nm, respectively for the o- and p-coumaric acid. In MS2 spectrum of the p-coumaric acid, the most intense peak was obtained at m/z 119, and a secondary peak with abundance of 20% at m/z 128 was also identified. The o-coumaric acid MS2 spectrum showed only the most abundant peak at m/z 119. The MS3 spectra of both isomers showed no peaks, as reported here for the UC. The identification of the two isomers was also confirmed by those authors using NMR data. Since the UV maxima of the UC was 276 nm and the MS2 spectra presented the most intense peak at m/z 119, due to the coumaric residue fragmentation, and no peak at 128, we believe that the UC is an ester of the ortho isomer of coumaric acid.
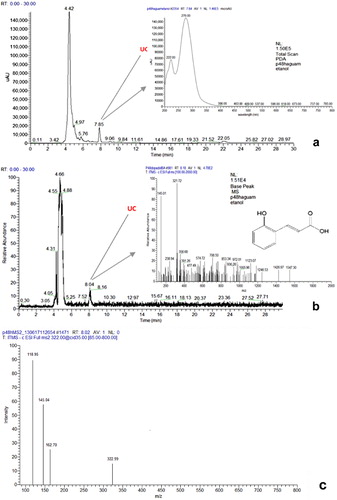
We did not find o-coumaric acid ester in P. arhizus mycelium alone or in the liquid phase culture medium of P. pinea without fungal inoculation.
Discussion
Signaling compounds in the ECM symbiosis are not as well known as those in the arbuscular associations. In AM symbioses, flavonones, flavones, and isoflavones are capable of stimulating spore germination, hyphal elongation, and hyphal branching (Graham Citation1991; Siqueira et al. Citation1991; Tsai and Phillips Citation1991; Harrison & Dixon Citation1993; Scervino et al. Citation2005a, Citation2005b). In the case of legumerhizobia symbiosis, other phenolic acids like hydroxybenzoic and hydroxycinnamic acids that are derived from the general phenylpropanoid pathway act as signaling molecules in the initiation of the symbioses and as agents in the plant defense (Mandal et al. Citation2010). Peters et al. (Citation1986) have suggested the possible involvement of the phenolic exudate luteolin in the control of nodABC expression for the nodule development in Rhizobium meliloti. This result suggested yet another function of the flavonoids in addition to the well-known involvement in plant defense.
In ECM associations, indole auxins, phenolic compounds, and flavonoids were implicated as signaling molecules. It has been reported that Pisolithus spp. secret hypahorine, IAA, and other growth regulators that trigger morphological changes in the root system of host plants (Niemi et al. Citation2000; Martin et al. Citation2001). The involvement of P. arhizus in promoting branching to form second- and third-order lateral roots in both conifer and angiosperm hosts was previously reported by Chambers and Cairney (Citation1999). In earlier study, we demonstrated that the presence of P. arhizus during in vitro co-culture with P. pinea plantlets enhanced the adventitious root growth, before the physical contact occurred, and later, the mycorrhization improved the survival rate of plants during acclimatization (Ragonezi et al. Citation2012).
In the present study, we identified a putative signaling compound in the liquid phase of the double-phase medium in the co-culture of P. pinea plantlets with P. arhizus. LC–DAD–MS analysis identified the substance as an ester of o-coumaric acid, a phenolic acid produced by plants and other organisms as secondary metabolite and a common chemical constituent in the plant kingdom (Abdul-Raman & Habib Citation1989; Vega et al. Citation2008; Ngoc et al. Citation2009; Sellami et al. Citation2009; Canuto et al. Citation2010). Among many effects, phenolic acids exert allelopathy and are implicated in the development of ecological interactions with the adjoining plant and with some rhizospheric organisms (Kefeli et al. Citation2003). For example, o-coumaric acid abundantly produced by Euapatorium adenophorum (a notorious weed worldwide) had a selective allelopathic effect that was toxic for Arabidopsis thaliana seed germination and plant growth but not for its own seed germination (Zheng et al. Citation2012). This result suggests that o-coumaric acid might render E. adenophorum a competitive advantage over neighboring plants during its invasion and establishment. Whether P. pinea o-coumaric acid has an allelopathic effect requires further research.
Our results demonstrated that o-coumaric acid ester was produced in the first two days of the co-culture and that its concentration tended to increase during the first 10 days. No o-coumaric acid ester was identified in the negative controls, suggesting that pine roots might have secreted this compound in response to the presence of fungal mycelium. It is therefore plausible that o-coumaric acid ester played a signaling role in establishing the relationship with ECM associations before the physical contact. These results corroborate recent findings of Plett and Martin (Citation2012) in which poplar root exudates induced the expression of MiSSP7 (symbiosis-up regulated gene indispensable for the establishment of symbiosis) in the ECM fungus Lacaria bicolour. The expression of MiSSP7 protein occurred before the contact with the root. The authors also discovered that rutin and quercetin flavonoids were able to induce the expression of MiSSP7 even in the absence of the tree host. Metabolites such as butanoic acid, cinnamic acid, o- and p-coumaric acid, vanillic acid, and p-hydroxybenzamide occurred in the rhizosphere of Arabidopsis when challenged with the gram-negative bacterial pathogen Pseudomonas syringae pv. tomato (Bais et al. Citation2005). The authors reported that bacteria could effectively modify the antimicrobial plant response, because the strains which were partly resistant to these compounds were able to block the exudation of antimicrobials using a mechanism based on the type III secretory system (Bais et al. Citation2005).
In this study, P. arhizus mycelium grew continuously in the co-cultures and this could be attributed to a similar ability possessed by Pseudomonas strains to modify and/or use o-coumaric acid for its benefit (Ruzzi et al. Citation1997; Barghini et al. Citation1998). Similar results were obtained by Zeng and Mallik (Citation2006) who studied the detoxifying effect of ECM fungi (Laccaria laccata, L. bicolour, and Paxilus involutus) on black spruce (Picea mariana) phenolic compounds produced by the understory plant Kalmia angustifolia. Ferulic acid and o-coumaric acid were degraded within 10 days and the degraded amount depended on the fungus species. The authors concluded that certain ECM fungi not only offered protection to the host plants against phenolic allelochemicals released from neighboring plants, but could also use them as carbon source. This is one of the mechanisms that ECM fungi use to control species interactions in higher plants by changing the rhizosphere chemistry. In our study, on day 10 of co-culture the compound was either not degraded yet or the degradation by the fungus (or utilization for growth) was substituted by the plant secreting new o-coumaric acid ester. In another study by Münzenberger et al. (Citation2003), detoxification of ferulic acid by ECM fungi Laccaria amethystina and Lactarius deterrimus grown in liquid culture showed different detoxification pattern. Both studies confirmed the ability of the symbiont to degrade and detoxify phenolic compounds, and that this ability was not only species-specific, but also specific to different strains of the same fungus species.
On the other hand, several studies have shown a decrease or no change in phenolic compounds in roots inoculated with different ECM fungi. Niemi et al. (Citation2007) found changes in flavonols, catechins, and condensed tannins in inoculated seedlings of Scots pine seedlings with two strains of Suillus variegatus. The authors analyzed their contents in needles, shoots, and roots. Quantitative changes in flavonols and conjugated Polyamines (PAs) were found in the aerial part but not in the roots. The authors concluded that these chemical mediators might be implicated in plant defense reactions rather and do not play a major role in the regulation of the establishment of ECM in Scots pine roots. Also, Schützendübel and Polle (Citation2002) showed that Scots pine short root tips covered by the mycelium of P. involutus (Batsch) Fr. contained less catechin than nonmycorrhizal root tips. Using HPLC analysis, Münzenberger et al. (Citation1995) detected p-hydroxybenzoylglucose, p-hydroxybenzoic acid glucoside, picein, catechin, and epicatechin in nonmycorrhizal fine roots of Larix decidua. Mycorrhizal Larix fine roots contained smaller quantities of these compounds compared with nonmycorrhizal fine roots. In addition, the cell wall-bound ferulic acid was at much lower concentration in mycorrhizal than in nonmycorrhizal fine roots. Pure mycelia of Laccaria amethystea (Bull.) Murr. contained none of the identified phenolics. The authors concluded that L. amethystea induced a decrease in soluble and cell wall-bound phenolics in fine roots of L. decidua Mill., which in turn led to their rapid mycorrhization.
However, it is difficult to compare our results obtained before physical contact between partners, with ones of other authors obtained when symbiosis is already established. In our case we assume that we are dealing with root exudates, on the contrary when the symbiosis is already established different flavonoids are found inside the root tissues. It is also possible that different flavonoids play different roles at different stages of the ECM symbiosis. These aspects are of crucial importance and should be elucidated by continuous analysis during the whole process, inside and outside the root for a given conifer plant and ECM fungus. Biological assays regarding transcriptional analyses should be performed with various pairs of partners to test the molecular target of ECM establishment.
The result of our study is a significant contribution to the list of flavonoids involved in the symbiotic relationships, because it is the first time that o-coumaric acid derivative was identified in the mutualistic interaction between pine species and P. arhizus, possibly through the initial cross talk between the partners. This might explain the positive effect of the in vitro fungal inoculation on the stone pine root growth compared with the noninoculated plantlets (Ragonezi et al. Citation2012).
Acknowledgments
This research was supported by the Portuguese Foundation for Science and Technology (FCT) through the project PTDC/AGR-CFL/71437/2006). K. Klimaszewska was supported by Natural Resources Canada.
References
- Abdul-Raman AA, Habib SA. 1989. Allelopathic effects of alfalfa (Medicago sativa) on bladygrass (Imperata cylindrica). J Chem Ecol. 15:2289–2300. doi:10.1007/BF01012082
- Amalesh S, Gouranga D, Sanjoy KD. 2011. Roles of flavonoids in plants. Int J Pharm Sci Tech. 6:12–35.
- Atoui AK, Mansouri A, Boskou G, Kefalas P. 2005. Tea and herbal infusions: their antioxidant activity and phenolic profile. Food Chem. 89:27–36.
- Bais HP, Loyola Vargas VM, Flores HE, Vivanco JM. 2001. Root specific metabolism: the biology and biochemistry of underground organs. In Vitro Cell Dev Biol-Plant. 37:730–741.
- Bais HP, Prithiviraj B, Jha AK, Ausubel FM, Vivanco JM. 2005. Mediation of pathogen resistance by exudation of antimicrobials from roots. Nature. 434:217–221.
- Barghini P, Montebove F, Ruzzi M, Schisser A. 1998. Optimal conditions for bioconversion of ferulic acid into vanillic acid by Pseudomonas fluorescens BF13 cells. Appl Microbiol Biothechnol. 49:309–314.
- Bonfante P, Genre A. 2010. Mechanisms underlying beneficial plant-fungus interactions in mycorrhizal symbiosis. Nat Commun. 4: 1–11. doi:10.1038/ncomms1046
- Canuto KM, Lima MA, Silveira ER. 2010. Amburosides C-H and 6-o-protocatechuoyl coumarin from Amburana cearensis. J Braz Chem Soc. 21:1746–1753.
- Castro MR, Ragonezi C, Oliveira P, Zavattieri A. 2012. Patent - method for extraction of metabolic root exudates from in vitro plant cultures of Pinus pinea L. National Institute of Industrial Property Portugal (INPI) No. 105239.
- Chambers SM, Cairney JWG. 1999. Pisolithus. In: Cairney JWG, Chambers SM, editors. Ectomycorrhizal fungi. Key genera in profile. Berlin: Springer; p. 1–31.
- Estabrook EM, Yoder JI. 1998. Plant-plant communications: rhizosphere signaling between parasitic angiosperms and their hosts. Plant Physiol. 116:1–7.
- Graham TL. 1991. A rapid, high resolution high performance liquid chromatography profiling procedure for plant and microbial aromatic secondary metabolites. Plant Physiol. 95:584–593.
- Harrison MJ, Dixon RA. 1993. Isoflavonoid accumulation and expression of defense gene transcripts during the establishment of vesicular-arbuscular mycorrhizal associations in roots of Medicago truncatula. Mol Plant Microbe Interact. 6:643–54.
- Hassan S, Mathesius U. 2012. The role of flavonoids in root-rhizosphere signalling opportunities and challenges for improving plant–microbe interactions. J Exp Bot. 63:3429–3444. doi:10.1093/jxb/err430
- Kefeli VI, Kalevitch MV, Borsari B. 2003. Phenolic cycle in plants and environment. J Cell Mol Biol. 2:13–18.
- Lagrange H, Jay-Allgmand C, Lapeyrie F. 2001. Rutin, the phenolglycoside from eucalyptus root exudates, stimulates Pisolithus hyphal growth at picomolar concentrations. New Phytol. 149:349–355.
- Lloyd G, McCown, B. 1981. Commercially feasible micropropagation of mountain laurel, Kalmia latifolia, by the use of shoot tip culture. Proc Plant Prop Soc. 30:421–427.
- Lynn DG, Chang M. 1990. Phenolic signals in combination: implications for plant development. Annu Rev Plant Physiol Plant Mol Biol. 41:497–526.
- Mandal SM, Chakraborty D, Dey S. 2010. Phenolic acids act as signaling molecules in plant–microbe symbioses. Plant Signal Behav. 5:359–368.
- Martin F, Duplessis S, Ditengou F, Lagrange H, Voiblet C, Lapeyrie F. 2001. Developmental cross talking in the ectomycorrhizal symbiosis: signals and communication genes. New Phytol. 151:145–154.
- Münzenberger B, Hammer E, Wray V, Schauer F, Schmidt J, Strack D. 2003. Detoxification of ferulic acid by ectomycorrhizal fungi. Mycorrhiza. 13:117–121.
- Münzenberger B, Kotte I, Oberwinkler F. 1995. Reduction of phenolics in mycorrhizas of Larix decidua Mill. Tree Physiol. 15:191–196.
- Ngoc TM, Lee I, Ha DT, Kim HJ, Min BS, Bae K. 2009. Tyrosinase-inhibitory constituents from the twigs of Cinnamomum cassia. J Nat Prod. 72:1205–1208.
- Niemi K, Julkunen-Tiitto R, Häggman H, Sarjala T. 2007. Suillus variegatus causes significant changes in the content of individual polyamines and flavonoids in Scots pine seedlings during mycorrhiza formation in vitro. J Exp Bot. 58:391–401.
- Niemi K, Salonen M, Ernstsen A, Heinonen-Tanski H, Häggman H. 2000. Application of ectomycorrhizal fungi in rooting of Scots pine fascicular shoots. Can J For Res. 30:1221–1230.
- Oliveira P, Barriga J, Cavaleiro C, Peixe A, Potes AZ. 2003. Sustained in vitro root development obtained in Pinus pinea inoculated with ectomycorrhizal fungi. Forestry. 76:579–587.
- Peters NK, Frost JW, Long SR. 1986. A plant flavones, luteolin, induces expression of Rhizobium meliloti nodulation genes. Science. 233:977–980.
- Plazonić A, Bucar F, Maleš Z, Mornar A, Nigović B, Kujundžić N. 2009. Identification and Quantification of Flavonoids and Phenolic Acids in Burr Parsley (Caucalis platycarpos L.), Using High-Performance Liquid Chromatography with Diode Array Detection and Electrospray Ionization Mass Spectrometry. Molecules. 14:2466–2490.
- Plett JM, Martin F. 2012. Poplar root exudates contain compounds that induce the expression of MiSSP7 in Laccaria bicolor. Plant Signal Behav. 17:12–5.
- Ragonezi C, Caldeira AT, Martins MR, Dias LS, Santos-Silva C, Ganhão E, Miralto O, Pereira I, Louro R, Klimaszewska K, Zavattieri MA. 2012. Pisolithus arhizus (Scop.) Rauschert improves growth of adventitious roots and acclimation on in vitro regenerated plantlets of Pinus pinea. Propag Ornam Plants. 12:139–147.
- Ruzzi M, Barghini P, Montebove F, Schiesser Ponente A. 1997. Effect of the carbon source on the utilization of ferulic, m- and p-coumaric acids by a Pseudomonas fluorescens strain. Annali Di Microbiologia Ed Enzimologia. 47:87–96.
- Scervino J, Ponce M, Erra-Balsells R, Vierheilig H, Ocampo JA, Godeas A. 2005a. Flavonoids exclusively present in mycorrhizal roots of white clover exhibit a different effect on arbuscular mycorrhizal fungi than flavonoids exclusively present in non-mycorrhizal roots of white clover. J Plant Interact. 1:15–22.
- Scervino J, Ponce M, Erra-Balsells R, Vierheilig H, Ocampo JA, Godeas A. 2005b. Flavonoids exhibit fungal species and genus specific effects on the presymbiotic growth of Gigaspora and Glomus. Mycol Res. 109:789–794.
- Schützendübel A, Polle A. 2002. Plant responses to abiotic stress: heavy metal-induced oxidative stress and protection by mycorrhization. J Exp Bot. 53:1351–1365.
- Seddas P, Gianinazzi-Pearson V, Schoefs B, Küster H, Wipf, D, Balu F. 2009. Communication and signaling in the plant–fungi symbiosis: the mycorrhiza. In: Baluška F, editor. Plant-environment interactions. Signaling and communication in plants. Berlin, Heidelberg: Springer; p. 45–71.
- Sellami IH, Maamouri E, Chahed T, Wannes WA, Kchouk ME, Marzouk B. 2009. Effect of growth stage on the content and composition of the essential oil and phenolic fraction of sweet marjoram (Origanum majorana L.). Ind Crop Prod. 30:395.
- Siqueira JO, Safir GR, Nair MG. 1991. Stimulation of vesicular-arbuscular mycorrhizae formation by flavonoid compounds. New Phytol. 118:87–93.
- Tsai SM, Phillips DA. 1991. Flavonoids released naturally from alfalfa promote development of symbiotic Glomus spores in vitro. Appl Environ Microbiol. 57:1485–1488.
- Vega M, De Carvalho M, Vieira I, Braz-filho R. 2008. Chemical constituents from the Paraguayan medicinal plant, Eupatorium macrocephalum Less. J Nat Med. 62:122–123
- Walker TS, Bais HP, Grotewold E, Vivanco JM. 2003. Root exudation and rhizosphere biology. Plant Physiol. 132:55–51.
- Zheng G, Jia Y, Zhao X, Zhang F, Luo S, Li S, Li W. 2012. O-Coumaric acid from invasive Eupatorium adenophorum is a potent phytotoxin. Chemoecology. 22:131–138.
- Zeng R, Mallik A. 2006. Selected ectomycorrhizal fungi of black spruce (Picea mariana) can detoxify phenolic 484 compounds of Kalmia angustifolia. J Chem Ecol. 32:1473–1489.