Abstract
The role of salicylic acid (SA) in regulating wheat calli growth and peroxidase activity in the co-culture with bunt (Tilletia caries (D.C.) Tul.) and smut (Ustilago tritici Pers.) pathogens was studied in this work. We found that the influence of SA increased the number of globular high-density sites with meristema-like cells and reduced the number of low-structured sites with parenchyma-like cells in wheat calli. SA inhibited fungi spores germination, mycelium growth, and formation of its new spores during the co-cultivation of wheat calli with bunt or smut agent. Interaction of fungi mycelium with wheat calli treated by SA led to formation of zones with hypersensitive reaction in calli high-structured sites with meristema-like cells. The obtained data about the influence of SA on activation of wheat calli growth and the peroxidases with pI ~3.5 and ~9.8, which can bind to the mycelium of T. caries or U. tritici are discussed in this paper.
Introduction
Bunt (Tilletia caries (DC.) Tul.) and smut (Ustilago tritici Pers.) agents are unique wheat fungal pathogens. They develop in wheat plants without any symptoms of disease over a long period of time and destroy the biological yield of wheat. Experimental works based on the investigation of plant–pathogen interaction with the use of new drugs in field conditions created some difficulties because in this case environmental safety should also be monitored (Gisi et al. Citation2010). The use of traditional chemical pests results in environmental contamination. It demands the search for new environmentally friendly drugs and ways of struggle with pathogens (Mishra et al. Citation2012). Primary screening of the immunomodulating activity of these drugs in field conditions is the most difficult part of the work, because in laboratory conditions only fungicide activity can be tested. The opportunity of studying their plant resistance inducing properties in these conditions is limited (Martinez-Espinoza et al. Citation2002).
Earlier, the method of tissue culture was actively used for plant–pathogen interactions investigation (Trillas et al. Citation2000). So, the detection of bunt and smut agent's development on wheat calli cultures is very interesting. This method opens new opportunities for studying molecular mechanisms of plants defense against these pathogens. The interaction in cellular co-culture of host plant and pathogen is similar to their interaction in native conditions (Sethy et al. Citation2003).
The increase of reactive oxygen species (ROS) production as well as the increase of H2O2 production can be referred to the universal plant defense reactions against different stressors (Bairley-Serres & Mittler Citation2006; Kong et al. Citation2013). H2O2 is the signal component of the initiation of the plant defense reaction cascade. Basic enzymes, which are part of ROS formation, are nicotinamide adenine dinucleotide phosphate (NADPH)-oxidase and peroxidase. Lately, researchers' attention has been turned to the possibility of salicylic acid (SA) to participate in various stressful conditions (Catinot et al. Citation2008; Idrees et al. Citation2010). Thus, for example, pathogen influence on plants leads to SA excretion into the apoplast and further transport into the plant (Alvarez, Citation2000). SA influences plant growth and development (Yusuf et al. Citation2013), and stimulates the systemic acquired resistance (SAR) of plants to various pathogens (van Loon Citation2007; Barba-Espin et al. Citation2010; Rivas-San & Plasencia Citation2011).
This work is devoted to studying SA influence on the interaction of wheat calli with bunt (T. caries) and smut (U. tritici) pathogenic agents. Development of the defense reactions of the host immune system connected with the activity of peroxidase and accumulation of H2O2 was discovered.
Materials and methods
Growing of wheat calli and inoculation by pathogen spores
Investigations were carried out with soft wheat calli (Triticum aestivum L.), cv. Zhnitsa. Wheat ripe germs were used as explants for obtaining cell material. Germs were isolated from seed vessels and were planted onto the nutrient Murashige and Skoog medium (MS) (Murashige & Skoog Citation1962) without or with the addition of 0.05 mM SA at 26°C in the dark. Calli were infected by T. caries or U. tritici spores on day 10 after getting the second subculture. Infection load per calli was about 100 fungal spores. Morphological analysis of the calli growth and the fungi mycelium expansion on the calli surface and inside co-cultures with pathogen was carried out for 20 days after the infection.
Microscopic supervision: histological analysis
Growth of T. caries mycelium on wheat calli was studied after having been fixed in ethanol and acetic acid mix (3:1 on volume) at 4°C during 4 h. Fixed samples were transferred to paraffin and cut out on the sledge microtome Reichert (Reichert, Austria). Slides were analyzed on an Imager M1 microscope (Carl Zeiss, Geramany) after having been colored in 1.0% water solution of methylene blue and shipped in the Canadian balm.
Microscopic supervision: hystochemical analysis
For the determination of peroxidase location, calli were stained with 3,3-diaminobenzidine (DAB) in 0.1 M Na-phosphate buffer (PB) in the presence of 0.016% H2O2 as described in Thordal-Christensen et al. (Citation1997) method.
Peroxidase activity and isoenzyme composition assay
In order to isolate the soluble protein fraction, wheat calli were homogenized in 0.01 M PB with pH 6.0 (sample: PB volume =1:3), and the enzyme was being extracted at 4°C for 1 h. The obtained protein extracts were centrifuged for 10 min at 8000 g, using a 5415R microfuge (Eppendorf, USA). The supernatant was collected for the determination of peroxidase activity and isoenzyme spectrum. Peroxidase activity was determined by the micromethod. The sample of 0.075 ml was diluted in PB (1:50) and in 0.025 ml of (o)-phenylenediamine at the concentration of 0.5 mg/ml. The prepared solution was added to the wells of flat-bottomed immunological plates (Nunc, USA). The development of color was terminated with 0.05 ml of 4 N H2SO4 two minutes after the addition of 0.025 ml of 0.016% H2O2. The rate of chromophore response of the samples was determined at 490 nm using a Benchmark Microplate Reader (BioRad, USA).
Isoelectric focusing of protein extracts was conducted using an apparatus manufactured by Hiiu Kalur (Estonia) with 7% polyacrylamide gel (PAAG) and 2.5% BioLytes 3/10 (BioRad, USA) within a working pH interval from 3 to 10 units. Aliquots were dialyzed and the concentration of protein therein was equalized before the application to PAAG. Isoperoxidases were detected with 0.01% solution of DAB in 0.1 M PB and in the presence of 0.016% H2O2 in the gel. After that, PAAG was examined using a scanner (Genius, USA). Isoelectric points (pI) of wheat calli peroxidases were determined using a set of marker proteins with known pI at pH from 3.5 to 10.6 (Serva, USA).
Sorption of wheat isoperoxidases on T. caries and U. tritici mycelium
The sample of spore material (100 mg) was axenically germinated on Czapek's agar for a week at room temperature and subsequently removed from the medium surface with 0.1 M PB. Peroxidase chromatography on the spores of bunt and smut was conducted in bulk. Protein extract, containing peroxidase, was mixed with the suspension of pathogen mycelium in PB and stirred for 30 min. Then, the mycelium was washed out by centrifugation until the removal of peroxidase activity from the supernatant. Bound isoforms of peroxidase were eluted with 1 M NaCl in 10 mM PB. The eluate was concentrated and used for the analysis of peroxidase activity and isoperoxidase pattern.
Measuring of the H2O2 concentration in calli
Plant extract was centrifuged and 50 µl of the supernatant was added to 500 µl of the reagent containing 0.074% Mohr's salt in 5.81% H2SO4 and 0.009% xylenol orange in 1.82% sorbitol (at the ratio of 1:100) and incubated at room temperature for 45 min (Vuletic & Sukalovich Citation2000). The reaction mixture was centrifuged at 8000 g for 10 min, and the optical density of the complex was measured at 560 nm with the BioSpec-Mini spectrophotometer (Shimadzu, Japan). Hydrogen peroxide concentration was determined using a preliminarily built calibration curve (Reachim, Russia).
Statistical analyses
The results were confirmed by two independent experiments. Statistical significance of the differences between mean values was determined by the Student's t -test. The presented data are mean values±SD.
Results
Visual analyses
We used wheat calli co-cultured with biotrophic fungi, the bunt pathogen T. caries and the smut pathogen U. tritici, as a model for studying host–parasite interaction. The control wheat calli on MS medium were friable, coarse globular with little compacted areas. T. caries and U. tritici spores were germinated exclusively on the surface of friable calli on the fourth and fifth day after inoculation. The air fungi mycelium on surface of wheat calli became clearly visible on the seventh day after inoculation. On day 20, the mycelium of T. caries and U. tritici covered approximately 40% and 50% of the calli, respectively. Thus, we have created long-living co-cultures of bunt T. caries and smut U. tritici fungi agents on wheat calli ().
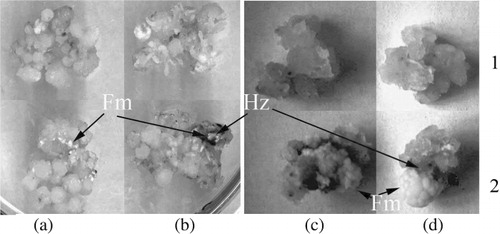
Mycelium of both pathogens colonized five to seven layers of peripheral wheat calli cells and was observed in both intercellular space and inside parenchyma-like cells of calluses, but both pathogens did not colonize zones of meristema-like cells and cells of conducting system of rhizoids. Both similarity and difference can be observed in morphological structure of intra-tissue mycelium of T. caries and U. tritici. The filaments of mycelium were very thin, long, and weak-stained, while the hyphae of T. caries were like sparingly branched filaments with different thickness. Part of them (stick-like) were strongly basophilic, and others (beads-like) were weak-stained. About two months after spore inoculation, we observed the increasing of mycelium and calluses fresh weight ( and ). This fact showed that plant cells and fungi mycelium were viable during the experiment.
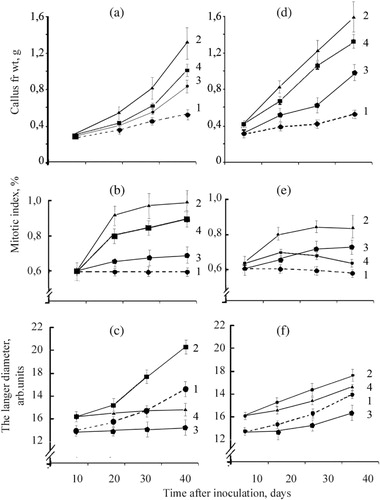
SA reduced the zone of parenchima-like the cells of calli which, consequently, led to the increase of the number of areas of meristema-like cells. Increase of the level of globular sites under SA influence in plant calli was observed by other researchers (Luo et al. Citation2002; Sharma et al. Citation2006). The T. caries spores do not grow on globular sites of wheat calli with meristema-like cells. We observed the necroses in the zone of application of fungi spores on control calli on the fifth and tenth day after inoculation, but SA induced this reaction on the second and third day after inoculation. Such phenomenon probably characterizes the defense reaction of calli cells with pathogen structures which is like the response of resistant plants to the infection by an incompatible form of pathogens. We can suppose that the appearance of necroses is connected with excretion of the proteases from growing spores of T. caries, as described in the co-culture of wheat calli with T. indica (Mania et al. Citation2005). Such a reaction was observed earlier in the plants subjected to the combined action of pathogen and SA.
The bunt and smut agents stimulated the formation of rhizoids on calli. We supposed that this phenomenon is connected with the production of indoleacetic acid (IAA) with bunt and smut pathogens. This assumption is based on the earlier results which showed a substantial increase of the level of IAA in the wheat seedling infected with T. caries (Maksimov et al. Citation2002).
It is shown in – that the co-culture of wheat calli with bunt and smut agents had a higher fresh weight than the control calli without pathogen. Treatment by SA led to the increase of fresh weight of cultured calli. We observed fast accumulation of biomass of the infected calli growing on the media with SA within nine days from the moment of infection. Furthermore, sharp (almost threefold) decrease of the rate of calli biomass accumulation was observed and, by the end of the experiment, this index did not essentially differ from the control level. At the same time, we observed the formation of necrotic lesions in the zones of mycelium localization and an appreciable suppression of the surface growth of pathogen on wheat calli treated by SA.
We did not find any data about the necrosis of calli during the infection process induced by bunt and smut agents. Therefore, co-cultures of wheat calli with T. caries or U. tritici represent a convenient model for a visual assessment of the influence of potential inductors of the resistance on the development of early protective reactions of plant cells to pathogen invasion.
SA led to the increase of the number of globular sites with meristema-like cells in wheat calli ( and ). It explains the emergence of a large number of globular sites in option with SA and SA+ to T. caries or SA+ to U. tritici. In wheat calli, the pathogens caused the increase of parenchyma-like calli cells diameter ( and ). On the contrary, under the influence of SA the diameter of these cells did not increase considerably. Respectively, the obtained data showed that under the influence of SA the cells led to the break of accumulation of parenchyma-like cells in wheat calli and at the same time the increase of both mitotic index and number of globular sites with meristema-like cells.
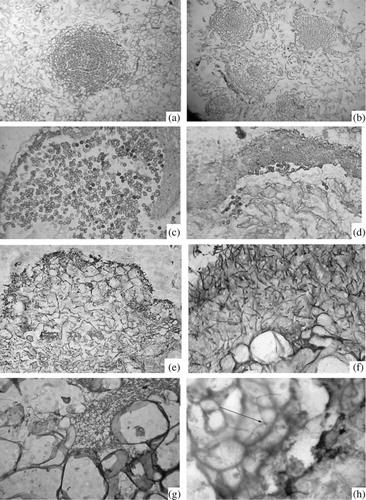
Microscopic observation
The formation of T. caries spores in control calli was observed on day 20 after the start of the experiment ( and ). SA decreased the number of new fungi spores formed in co-culture of both pathogens ( and ). Dense sites of calli represented the zones of meristema-like cells (). Under the influence of SA, the number of globular sites with meristema-like cells increased as compared with the calli growing on the MS medium ().
In the present work, we have noticed that after inoculation, the mycelium of bunt and smut pathogens was detected exclusively in the intercellular space between parenchyma-like cells. Loss of parenchyma-like cells (around 20%) and intercellular spaces with DAB colored material in the fungus penetration zone is observed (). Directly on the hyphae of the fungus, we also observed DAB-colored material (). During cultivation of infected wheat calli on the SA, the number of DAB cells in the fungus growth area increased by 30%, which probably, together with the decrease of the level of friable zones on calli caused deceleration of fungus growth.
Peroxidase activity, isoenzyme composition, and sorption of isoperoxidase on mycelium of T. caries and U. tritici
Peroxidase activity in the cytoplasmic fraction increased on day 10 day after calli inoculation with pathogens as well as after cultivation on the nutrient medium containing SA (). The highest peroxidase activity was observed in the calli which grew on the medium containing SA and were infected by bunt and smut pathogens, which proved the engagement of this enzyme in defense. The analysis of extracellular fraction revealed that under the influence of pathogen peroxidase activity of the calli was higher in comparison with control ones. Possibly, low activity of peroxidase in the extracellular fraction promotes growth of pathogen in intercellular space of the friable parenchima-like cells. Under SA influence, the peroxidase activity in ion-binding fraction increased on 160%.
Table 1. The influence of co-cultivation of wheat calli with the bunt (T. caries) and smut (U. tritici) pathogens on the MS medium with or without salicylic acid on peroxidase activity in calli.
The analysis of isoperoxidase pattern () showed that wheat calli was characterized by the high activity of isoforms with pI ~9.7, 9.3, 7.5, 4.2–4.5, and 3.5. Bunt and smut agents activated these isoforms, and in addition to isoperoxidases with pI~9.7 and 3.5 (). Significantly, the increase of activity of these isoperoxidase was observed in infected calli, cultivated in the presence of SA. Thus, SA was an effective trigger of the synthesis and activation of pathogen-induced isoperoxidases in plants. Interestingly, that infection by T. caries or Fusarium of the resistant forms of wheat-activated isoperoxidases which can sorb on pathogen mycelium or chitin (Khairullin et al. Citation2000; Caruso et al. Citation2001). Furthermore, these isoperoxidases possessed antifungal activity (Caruso et al. Citation2001; Akhunov et al. Citation2008).
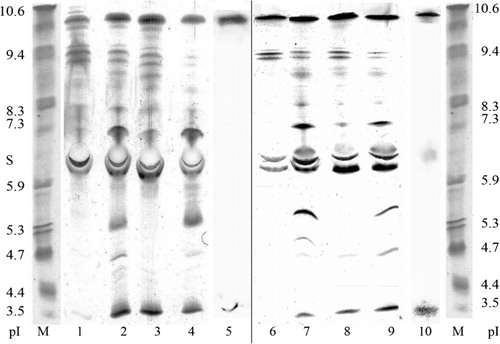
Detection of the sorption of peroxidases on mycelium surface of T. caries and U. tritici by microscopic and biochemical methods (), suggests the important role of these isoperoxidases in the plants defense system.
Changes in hydrogen peroxide level
SA increased the level of H2O2 markedly in infected wheat calli by 30% on the 24 h and fourfold on 48 h after inoculation relative to control (). Organized growth of calli and the increase of their resistance to bunt and smut agents under the influence of SA could be explained by the activation of the enzymes generating H2O2 and by the increase of their endogenous content. The results of our observations confirmed this hypothesis. Apparently, the infection of calli and their cultivation on SA raised the level of endogenous H2O2.
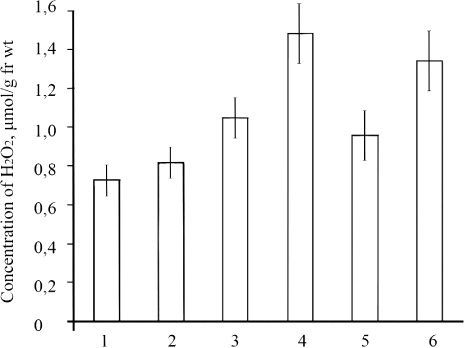
Discussion
It is known that SA is a natural signaling molecule involved in plant defense response against biotrophic pathogen infection (Kawano & Furuichi Citation2007). Co-culture of plant calli with pathogens, especially with smut and bunt pathogens, is a convenient model for the study of interactions between plant hosts and their pathogens (Roy et al. Citation2008).
Earlier we demonstrated that bunt invasion in wheat tissue rapidly increased IAA level in calli (Maksimov et al. Citation2004). Such a change in IAA content can serve as a precondition for hypertrophied growth of plant cells co-cultured with a pathogen. After analyzing the SA effect, the growth of plant cells co-cultured with a pathogen and accumulation of IAA (Maksimov et al. Citation2004), we have come to the conclusion that SA participates in the regulation of this hormone level. It is really known that exogenous SA treatment triggers the stabilization of auxin/indole-3-acetic acid (AUX-IAA) protein and it negatively regulates auxin signaling (Wang et al. Citation2007).
It has been shown that SA stimulates morphogenesis in wheat calli, and inoculation with T. caries results in the formation of areas with hypersensitive response (Maksimov et al. Citation2004). Formation of necroses is induced in the leaves and suspension culture of wheat cells under the influence of SA. This phenomenon depends on the changes in the status of pro-/antioxidant systems in plant cells (Maksimov et al. Citation2012).
SA and auxin signaling pathways interact, for the most part, antagonistically and it is reflected in the calli growth changes (Bari & Jones Citation2009). If auxin signaling correlates with increased susceptibility to biotrophic pathogens then SA leads to the development of SAR to them (Robert-Seilaniantz et al. Citation2011). For example, auxin treatment of plants suppresses the expression of pathogenesis-related protein (PR1) (Park et al. Citation2007). As a result of the repression of auxin signaling by SA plant growth suppression has also been demonstrated. Treatment of Arabidopsis plants with an SA analog, benzothiadiazole S-methyl ester (BTH) results in the repression of a number of auxin responsive genes. These genes include an auxin importer AUX1, an auxin exporter PIN7, auxin receptors TIR1 and AFB1, and the genes belonging to auxin inducible proteins SAUR and AUX/IAA family (Wang et al. Citation2007). A decrease in Arabidopsis biomass following SA treatment is abolished in an axr3–1 mutant background (Canet et al. Citation2010). Similarly, it is found that the majority of the above auxin inducible genes is also repressed in systemic tissues after the induction of SAR. The necrotrophic fungi Plectosphaerella cucumerina and Botrytis cinerea are more virulent for the auxin signaling mutants axr1, axr2, and axr6 which is consistent with the antagonistic relationship between SA and auxin signaling (Robert-Seilaniantz et al. Citation2011). This indicates that the SAR response involves downregulation of auxin responsive genes. However, the level of free auxin did not change after SA treatment. In addition, SA has been shown to inhibit the expression of synthetic auxin-responsive promoter (containing response elements such as TGTCTC) fused to the reporter gene GUS (beta-glucuronidase) DR5::GUS. Wang et al. (Citation2007) argue that SA stabilizes AUX/IAA auxin repressors by limiting auxin receptors which are needed for the downregulation of AUX/IAA proteins. On the other hand, SA plays an important role in functioning of signaling system regulating ROS level in plants. Earlier we have observed that the addition of SA to the medium of co-culturing of wheat calli with the fungus T. caries increases the resistance of plant cells to pathogen (Maksimov et al. Citation2002). This is related to changes in peroxidase activity, H2O2 accumulation and formation of embryogenic structures. As SA accelerates the formation of embryogenic cell complexes, it can be assumed that the defense role of wheat chitin-specific anionic peroxidase with pI ~3.5 which are accumulating in soluble and extracellular fractions is related with strengthening of cell walls of the attacked calli (Vlot et al. Citation2009). Its accumulation in the cell wall area under the effect of SA contributes to the quick saturation of the zone of inoculation with the products of enzymatic reaction, which are involved in the lignification of cell walls of the host plant and in the formation of secondary low-molecular compounds with high defense activity.
It can be assumed that the transfer of some isoperoxidases to the extracellular ionic binding fraction will improve defense responses to pathogens (Lehtonen et al. Citation2009). For instance, it has been shown that two apoplastic isoperoxidases of poplar participate in lignification of cell walls (Lu et al. Citation2012). An important prerequisite for switching on lignin synthase activity of peroxidases is their ability to interact with cell wall polysaccharides (Maksimov et al. Citation2012). These results are interesting because isoperoxidases activated by SA and inoculation are capable to bind to chitin and are detect in the culture medium.
Peroxidases are not only constitutive but also inducible enzymes, which are synthesized under the influence of various physical, chemical, and biotic agents (Haluskova et al. Citation2009). Their molecular and functional heterogeneity makes it possible to change the ratio between isoforms in favor of these more adapted to adverse environmental conditions, and this increase in peroxidase activity may be considered as defense function of the plant organism (Morkunas & Gmerek Citation2007). When plant cells are exposed to pathogens and SA, wheat anionic peroxidases act as relatively mobile isoenzymes activated both in soluble and ionic bound fractions of protein.
The fact that wheat cationic isoperoxidase with pI ~9.8 and anionic isoperoxidase with pI ~3.5 are detected in extracellular protein fraction of calli growing on the medium containing SA suggests that SA induces their excretion into apoplast. Probably, it is connected with physiological and biochemical features of investigated fungi. So, the principal opportunity of co-culturing of wheat calli with bunt and smut agents is shown. This culture can be used for studying of mechanisms of plant cells resistance to fungal pathogen. As plant cells are susceptible to bunt and smut agents in co-culture, it can be used for the study of plants resistance and screening of various modulators of phytoimmunity.
Acknowledgment
The study was supported by the grant of the Russian Federation Ministry of Education and Science P-339.
References
- Akhunov AA, Golubenko Z, Khashimova NR, Mustakimova ECh, Vshivkov SO. 2008. Role of chitin-specific peroxidase in wilt-resistant cotton. Chem Nat Comp. 44:493–496.10.1007/s10600-008-9104-1
- Alvarez ME. 2000. Salicylic acid in the machinery of hypersensitive cell death and disease resistance. Plant Mol Biol. 44:429–442.10.1023/A:1026561029533
- Bailey-Serres J, Mittler R. 2006. The roles of reactive oxygen species in plant cells. Plant Physiol. 141:311–316.10.1104/pp.104.900191
- Barba-Espin G, Diaz-Vivancos P, Clemente-Moreno M, Albacete A, Faize L, Faize M, Perez-Alfocea F, Hernandez JA. 2010. Interaction between hydrogen peroxide and plant hormones during germination and the early growth of pea seedlings. Plant Cell Environ. 33:981–984.10.1111/j.1365-3040.2010.02120.x
- Bari R, Jones JDG. 2009. Role of plant hormones in plant defence responses. Plant Mol Biol. 69:473–488.
- Canet JV, Dobon A, Ibanez F, Perales L, Tornero P. 2010. Resistance and biomass in Arabidopsis: a new model for salicylic acid perception. Plant Biotechnol J. 8:126–141.
- Caruso C, Chilosi G, Leonardi L. 2001. A basic peroxidase from wheat kernel with antifungal activity. Phytochemistry. 58:743–750.10.1016/S0031-9422(01)00226-6
- Catinot J, Buchala A, Abou-Mapsour E, Metraux JP. 2008. Salicylic acid production in response to biotic and abiotic stress depends on isochorismate in Nicotiana benthamiana. FEBS Lett. 582:473–478.10.1016/j.febslet.2007.12.039
- Gisi U, Chet I, Gullino ML, editors. 2010. Recent development of plant diseases. Berlin, Heidelberg: Springer.
- Haluskova I, Valentovicova K, Huttova J, Mistrik J, Tamas L. 2009. Effect of abiotic stresses on glutathione peroxidase and glutathione S-transferase activity in barley root tips. Plant Physiol Biochem. 47:1069–1074.10.1016/j.plaphy.2009.08.003
- Idrees M, Khan MMA, Aftab T, Naeem M, Hashimi N. 2010. Salicylic acid-induced physiological and biochemical changes in lemongrass varieties under water stress. J Plant Interact. 5:293–303.10.1080/17429145.2010.508566
- Kawano T, Furuichi T. 2007. Salicylic acid as a defence-related plant hormone: roles of oxidative and calcium signaling paths in salicylic acid biology. In: Hayat S, Ahmad A, editors. Salicylic acid: a plant hormone. Berlin, Heidelberg: Springer; p. 277–322.
- Khairullin RM, Yusupova ZR, Troshina NB. 2000. Protective responses of wheat treated with fungal pathogens: interaction of wheat anionic peroxidases with chitin, chitosan, and thelyospores of Tilletia caries. Russian J Plant Physiol. 47:97–102.
- Kong L, Wang F, Si J, Feng B, Zhang B, Li S, Wang Z. 2013. Increasing in ROS levels and callose deposition in peduncle vascular bundless of wheat (Triticum aestivum L.) grown under nitrogen deficiency. J Plant Interact. 8:109–116.10.1080/17429145.2012.712723
- Lehtonen MT, Akita M, Kalkkinen N, Ahola-Iivarinen E, Ronnholm G, Somervuo P, Thelander M, Valkonen JPT. 2009. Quickly-released peroxidase of moss in defense against fungal invaders. New Phytologist. 183:432–443.10.1111/j.1469-8137.2009.02864.x
- Lu G, Kejian P, Yahua C, Guiping W, Zhenguo S. 2012. Roles of apoplastic peroxidases, laccases and lignification in the manganese tolerance of hyperaccumulator Phytolacca americana. Acta Physiol Plantarum. 34:151–159.10.1007/s11738-012-0995-x
- Luo J-P, Jiang S-T, Pan L-J. 2002. Enhanced somatic embryogenesis by salicylic acid of Astragalus adsurgens Pall.: relationship with H2O2 production and H2O2-metabolizing enzyme activities. Plant Sci. 161:125–132.10.1016/S0168-9452(01)00401-0
- Maksimov IV, Cherepanova EA, Sorokan AV. 2012. Polysaccharide-specific isoperoxidases as an important component of the plant defence system. In: Verbeek CJR, editor. Products and application of biopolymers, chapter 10. Croatia: Copyright InTech; p. 201–220.
- Maksimov IV, Surina OB, Sakhabutdinova AR, Troshina NB, Shakirova FM. 2004. Changes in the phytohormone levels in wheat calli as affected by salicylic acid and infection with Tilletia caries, a bunt pathogenic agent. Russ J Plant Physiol. 51:228–233.
- Maksimov IV, Troshina NB, Khairullin RM, Surina OB, Ganiev RM. 2002. The effect of the common bunt on the growth of wheat seedling and calluses. Russ J Plant Physiol. 49:685–689.10.1023/A:1020253305191
- Mania S, Purwar S, Singh BR, Gang GK, Kumar A. 2005. Effect of jasmonic acid on the growth, differentiation and senescence of calli from wheat showing differential immunity to Tilletia indica. Plant Cell Biotechnol Mol Biol. 7:97–102.
- Martinez-Espinoza AD, Garsia-Pedrajas MD, Gold SE. 2002. The Ustilaginales as plant pests and model systems. Fungal Genetics Biol. 35:1–20.10.1006/fgbi.2001.1301
- Mishra AK, Sharma K, Misra RS. 2012. Elicitor recognition, signal transduction and induced resistance in plants. J Plant Interact. 7:95–120.10.1080/17429145.2011.597517
- Morkunas I, Gmerek J. 2007. The possible involvement of peroxidase in defense of yellow lupine embryo axes against Fusarium oxysporum. J Plant Physiol. 164:185–194.10.1016/j.jplph.2005.11.005
- Murashige T, Skoog E. 1962. A revised medium for rapid growth and bioassays with tobacco tissue cultures. Plant Physiol. 15:473–497.10.1111/j.1399-3054.1962.tb08052.x
- Park J-E, Park J-Y, Kim Y-S, Staswick PE, Jeon J, Yun J, Kim S-Y, Kim J, Lee Y-H, Park, C-M. 2007. GH3-mediated auxin homeostasis links growth regulation with stress adaptation response in Arabidopsis. J Biol Chem. 282:10036–10046.10.1074/jbc.M610524200
- Rivas-San VM, Plasencia J. 2011. Salicylic acid beyond defence: its role in plant growth and development. J Exp Bot62:3321–3338.10.1093/jxb/err031
- Robert-Seilaniantz A, Grant M, Jones JDG. 2011. Hormone crosstalk in plant disease and defense: more than just jasmonate/salicylate antagonism. Annu Rev Phytopathol. 49:317–343.
- Roy A, Ghosh ST, Chandhuri M, Saha PK. 2008. Effect of different plant hormones on callus induction in Gymnema sylvestris R.B. African J Biotechnol. 7:2209–2211.
- Sethy NK, Pandey D, Singh US, Kumar A. 2003. Caspase-3 like protein in wheat – Tilletia indica dual culture system as potential biomarker for host resistance to Karnal bunt. Indian J Biotechnol. 2:596–602.
- Sharma A, Mathur PM, Rajamani G, Joshi A. 2006. Salicylic acid induced plantlet regeneration in peper (Capsicum fruitesceus) calli. Asian J Bio Sci. 1:35–36.
- Thordal-Christensen H, Zhang Z, Collinge DD. 1997. Subcellular localization of H2O2 accumulation in papillae and hypersensitive response during the barley-powdery mildew interaction. Plant J. 11:1187–1194.10.1046/j.1365-313X.1997.11061187.x
- Trillas MI, Cotxarrera L, Casanova E, Cortadellas N. 2000. Ultrastructural changes and localization of chitin and callose in compatible and incompatible interactions between carnation callus and Fusarium oxysporum., Physiol and Mol Plant Pathol. 56:107–116.10.1006/pmpp.1999.0254
- Van Loon LC. 2007. Plant responses to plant growth-promoting rhizobacteria. Eur J Plant Pathol119:243–254.
- Vlot AC, Dempsey DA, Klessig DF. 2009. Salicylic acid, a multifaceted hormone to combat disease. Annu Rev Phytopathol. 47:177–206.
- Vuletic M, Sukalovich VH. 2000. Characterization of cell wall oxalate oxidase from maize roots. Plant Sci. 157:257–263.
- Wang D, Pajerowska-Mukhtar K, Culler AH, Dong X. 2007. Salicylic acid inhibits pathogen growth in plants through repression of the auxin signaling pathway. Curr Biol. 17:1784–1790.
- Yusuf M, Hayat S, Alyemeni MN, Fariduddin Q, Ahmad A. 2013. Chapter 12. Salicylic acid: physiological roles in plants. In: Hayat S, Ahmad A, Alyemini MN, editors. Salicylic acid: plant growth and development. Dordrecht, Heidelberg, New York and London: Springer; p. 277–297.