Abstract
The fungal disease, charcoal root rot, caused by Macrophomina phaseolina is a foremost yield restraining factor of Sorghum bicolor L. around the world. The expression analysis of genes induced in general defense response can endow with clues to reveal major defense mechanisms against pathogen infection in sorghum plant. The role of chitinase and Stilbene synthase in response to M. phaseolina in sorghum was studied under control growth conditions using a real-time polymerase chain reaction. Here, we report the expression analysis of antifungal genes in two cultivars viz. PJ-1430 (resistant) and SU-1080 (susceptible) at different hours after inoculation with M. phaseolina isolate MTCC 2165. Chitinase and stilbene synthase were induced in PJ-1430 within 0 h, 24 h and in SU-1080 in 48 h, 24 h, respectively, after inoculation. However, the expression levels of chitinase and stilbene synthase in resistant cultivar were significantly higher. The results showed that chitinase and stilbene synthase can be effective to enhance resistance to M. phaseolina.
1. Introduction
Macrophomina phaseolina, a global devastating necrotrophic fungal pathogen, infects more than 500 plant hosts (Das et al. Citation2008; Ma et al. Citation2010; Zveibil et al. Citation2012) including food crops (maize, sorghum), pulse crops (common bean, green gram), fiber crops (jute, cotton), and oil crops (soybean, sunflower, sesame). Charcoal rot of sorghum caused by M. phaseolina, soil and seed-borne disease of sorghum, is endemic to tropical and temperate regions of the world (Wyllie Citation1998). The disease severity may be enhanced by hot and dry environment (Gaige et al. Citation2010) and may cause upto 100% yield losses (Bashir & Malik Citation1988). This disease can cause lodging and grain yield loss in the range of 23–64% under favorable conditions (Mughogho & Pande Citation1984) besides adversely affecting the quality of fodder. Recently, increased incidence of the pathogen on diverse crop species has been reported worldwide, highlighting the importance of this disease to crop production in drought prone regions (Muchero et al. Citation2011).
M. phaseolina infects plants on almost all growth stages and are instigated by seed, soil and plant residues (Reuveni et al. Citation1983). Colonization of pathogen of charcoal rot results in death of seedling because of impediment of xylem vessels. Plant defoliation and wilting occurs due to formation of red to brown lesions on roots and stems with production of dark mycelia and black microsclerotia (Abawi & Pastor-Corrales Citation1990). It is difficult to control M. phaseolina due to its persistence as sclerotia in the soil and plant debris. However, low levels of productivity in sorghum are due to drought and are aggravated by charcoal rot disease which usually appears at grain maturity stage and causes severe lodging. Sorghum charcoal rot is characterized by lodging of plants as they approach maturity.
Plants display an innate pathogen-specific resistance by producing responses such as oxidative burst of cell, change of cell wall composition that prevent infection, and de-novo synthesis of compounds like phytoalexins and pathogenesis-related (PR) proteins (Delaney et al. Citation1994; Xu et al. Citation1994; Wu & Bradford Citation2003). Among all induced responses, production of PR proteins is most important because they can lead to the increased resistance of the whole plant against a pathogenic attack (Ebrahim et al. Citation2011). Chitinase is one of the PR protein considered to be most important because it may lead to the increased resistance of the whole plant against a pathogenic attack. Chitinases, hydrolyze chitin, one of the major cell wall components of many pathogenic fungi (Borad & Sriram Citation2008). The chitinases cleave the cell wall chitin polymers in situ, resulting in a weakened cell wall and rendering fungal cells osmotically sensitive (Huang et al. Citation2005). Many studies on the stress defense mechanisms of plants have been implemented on the basis of gene expression (de Leon & Montesano Citation2013).
Sorghum has innate response mechanisms against pathogens that involve PR proteins. Several chitinases (three in 21–24 kDa range, 28 kDa and 30 kDa) have been reported in sorghum (Darnetty et al. Citation1993). The level of these chitinases was noted to rise during caryopsis development; however, their antifungal activity was not confirmed (Darnetty et al. Citation1993). Sorghum also synthesizes a unique class of flavonoid phytoalexins, the 3-deoxyanthocyanidins, as an essential component of defense mechanism against pathogen infection (Lo et al. Citation2002).
Stilbene synthase enzyme has been reported to produce phytoalexin resveratrol which imparts fungal resistance in plants (Hain et al. Citation1990). Sorghum was the first example of stilbene synthase (SbSTS1) gene in monocots (Yu et al. Citation2006) and is known to contribute to the plant defense against pathogens (Schroder & Schroder Citation1990). SbSTS1 is considered not only to be constitutively expressed but inducible following fungal inoculation (Yu et al. Citation2005).
Our previous work demonstrated increased activity of chitinase in Eruca sativa against Alternaria brassicicola infection (Gupta et al. Citation2012). The present study was undertaken to understand the role of two major PR proteins, viz. chitinase and stilbene synthase in defense response of sorghum on infection with M. phaseolina.
2. Materials and methods
2.1 Fungal strain and its activation
M. phaseolina was obtained from Institute of Microbial Technology, Chandigarh (MTCC 2165). The lyophilized fungal strain was activated by inoculating in the flask containing potato dextrose broth. This was done under proper aseptic conditions in the laminar flow hood. The flask was then incubated in the incubator shaker at a temperature of 25±2°C with shaking at 120 rpm for 5 days. This activated fungal strain was then streaked on the PDA slants. These slants were then incubated for 7 days (25±2°C). The mycelium growth was initiated after 2 days of incubation.
2.2 Preparation of inoculum
Inoculum was prepared under the laminar flow hood under aseptic conditions. The surface of the mycelium mat was scrapped gently 2–3 times with the sterilized inoculating loop and inoculated in the conical flask containing autoclaved distilled water. These flasks were then kept in the incubator shaker (25±20°C, 120 rpm) for 2 h. The mycelia were counted under the microscope using haemocytometer and the concentration was maintained at 105 mycelia/ml.
2.3 Plant growth and inoculation
Two cultivars of Sorghum bicolor viz. PJ-1430 (resistant) and SU-1080 (susceptible) which were of contrasting type were used for this study after screening of eight cultivars on the basis of growth and biochemical parameters, for the artificial infection with M. phaseolina isolate. Seeds were obtained from Agricultural Research Station, Durgapura, Jaipur. Seeds were surface sterilized with 1% HgCl2 for 2 min and then washed several times with distilled water. For sowing seeds were placed in pots of 15 cm diameter containing 1 kg sterilized soil and soilrite (3:1) in growth chamber under 16h/8h day/night photoperiod of 220±20 µE/m2/s at changing day/night temperature of 24°C/20°C (Oldach et al. Citation2001). Fifteen-day-old seedlings were used for inoculation with 10 ml of mycelial suspension of M. phaseolina using thin layer chromatography sprayer. The mock infected plants were sprayed with sterile water after rubbing the leaves with sand paper. The infected and the mock-infected plants were then placed in the growth chamber. Three plants from each treatment were used for RNA extraction at 0, 24, 48, 72, 96, 120, 144, and 168 h post-inoculation (HPI).
2.4 RNA isolation
Hundred milligrams of fresh sample of root and leaf were collected carefully around the site of infection (~10 mm) and homogenized in liquid nitrogen. Total RNA was extracted based on the principle of silica spin column technology to obtain pure and intact RNA from the tissues using RNA isolation kit (Genei, Bangalore).
2.4.1 Quantification of the isolated RNA
Quantification of the RNA content was done by diluting the samples with RNase-free water. Absorbance of the samples was measured at 260 and 280 nm. A260 reading of 1.0 is equivalent to 40 µg/ml of RNA. The purity of the sample was calculated from the A260/A280 ratio. For values higher than 1.6, the RNA purity was considered acceptable.
2.4.2 Electrophoretic separation of RNA
0.8% Agarose was melted in 1× TAE buffer [50×TAE buffer (40 mM Tris acetate, pH: 7.5, and 1 mM ethylenediamine tetraacetic acid) diluted with diethylpyrocarbonate-water]. After cooling down to about 60°C, 0.5 µg/ml ethidium bromide (EtBr) was added. For solidification, the gel medium was poured on horizontal gel trays equipped with combs to form vertical gel loading pockets. The polymerized gels were transferred into a RNase-free gel tank containing 1× TAE buffer. Two microliters of RNA were mixed with 6× loading buffer (0.25% (w/v) bromophenol blue, 0.25% (w/v) xylene glycol, 30% (v/v) glycerol) and loaded in the wells. The RNA was separated on the gels at 100 volts for 1 h. The fluorogram was documented photographically in a gel documentation system (Alpha Imager) as shown in . It represents 100 bp marker in the first lane, leaf (A) and root (B) total RNA in the subsequent lanes 2–6 for both varieties viz. PJ-1430 and SU-1080.
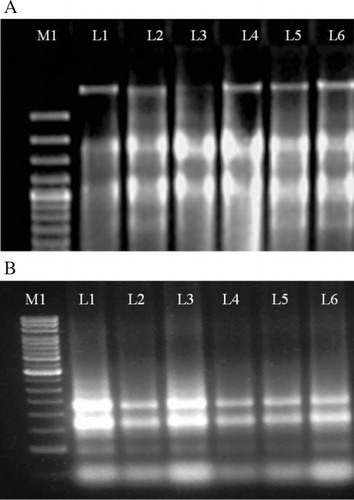
2.5 cDNA synthesis
cDNa was synthesized from total RNA by M-MuLV Reverse Transcriptase kit (Genei, Bangalore). Components of the kit were thawed, mixed and briefly centrifuged, and stored on ice prior to use. Following reagents were added into a sterile, nuclease-free tube on ice in the indicated order: total RNA 5 µg, oligo (dT) primer 1 µl, nuclease-free water 12 µl, gently mixed, centrifuged at 2500 rpm and incubated at 65°C for 5 min. The tube was chilled on ice, spun down, and placed back on ice. 5× reaction buffer 4 µl, RNase inhibitor 1 µl, 10 mM dNTP Mix 2 µl, M-MuLV Reverse Transcriptase 1 µl, total volume 20 µl were mixed gently and centrifuged at 2500 rpm for 5 min. For gene-specific primed cDNA synthesis, the samples were incubated for 60 min at 42°C. Reaction was terminated by heating at 70°C for 5 min.
2.6 RT-PCR analysis
Primer pairs specific to sorghum chitinase (NCBI accession numbers AY047608) and stilbene synthase (NCBI accession number AY069951) cDNA (sense primer, 5′-TAC TGC GGC ACG GAC GGA CGA CTA C-3′ and antisense primer 5′-G CAA GGA CTC GCG GCG GTT-3′; sense primer 5′-GTG GTG GGA ACG TGG GTA GC-3′ and antisense 5′-AA AGA AGC GGG TAC AGT GGG-3′, respectively) were used. Primers for sorghum actin (NCBI accession number X79378) cDNA (sense primer, 5′-AGG CGC AGT CCA AGA GGG GTA-3′; antisense primer, 5′-TGG AAG TTG TGG GGG GCG GTA-3′) were used as an internal control. All real-time polymerase chain reaction (RT-PCR) experiments were conducted in three replicates. Quantitative RT-PCR analysis was done to profile the expression of selected PR genes. Expressions of sorghum innate genes representing the upstream and downstream pathogenesis response system were profiled by qRT-PCR after infection of seedlings with M. phaseolina.
Expression levels of chitinase and stilbene synthase and actin genes were determined at various post-inoculation times by RT-PCR by establishing a gradient concentration that covered 40 nM for primers and 10–100 ng for cDNA. The RT-PCR conditions included initial activation step at 95°C for 5 min, denaturation at 95°C for 30 sec, and combined annealing/extension at 60°C for 30 sec. Melt curve analysis of PCR product was carried out at 72°C for 1 min and ramped from 72 to 98°C by 1°C every 5 sec. Relative gene expression was calculated in terms of fold change (–) using the following formula:
Table 1. Threshold values of root – stilbene synthase gene in PJ-1430 at different time intervals.
Table 2. Threshold values of leaf – stilbene synthase gene in PJ-1430 at different time intervals.
Table 3. Threshold values of root – stilbene synthase gene in SU-1080 at different time intervals.
Table 4. Threshold values of leaf – stilbene synthase gene in SU-1080 at different time intervals.
Table 5. Threshold values of root – chitinase gene in PJ-1430 at different time intervals.
Table 6. Threshold values of leaf – chitinase PJ-1430 at different time intervals.
Table 7. Threshold values of root – chitinase gene in SU-1080 at different time intervals.
Table 8. Threshold values of leaf – chitinase gene in SU-1080 at different time intervals.
2.7 Statistical analysis
Data are mean values of two experiments each containing three replications per treatment (n=6). All results were subjected to one-way analysis of variance using SPSS ver. 16.0. Tukey's test was performed at P<0.01 on each of the significant variables measured.
3. Results
To gain insight into molecular basis of resistance, some defense-related genes were analyzed for their expression levels in response to pathogen attack. The two innate genes selected for analysis were sorghum stilbene synthase (SbSTS1) and sorghum chitinase (SbChit). The in vitro entailed expression study in intact seedlings was carried out over a period of 168 h after infection. Leaf and root samples were taken for analysis at specific intervals. Expression of these genes was studied in the leaves and roots of 15-day-old plants. We monitored the penetration of pathogen during the growth of seedlings. We observed that fungus penetrates the roots in 7-day-old seedlings and is well established in 15-day plants. Hence, we proceeded with this study in 15-day-old plants. It was observed that expression levels for all these genes were significantly higher in resistant variety as compared to the susceptible one. Highest expression level of chitinase and stilbene synthase genes was observed at 72 HPI and 24 HPI in both resistant and susceptible varieties of leaf samples. The expression of SbChit and SbSTS1 was studied in M. phaseolina susceptible SU-1080 and resistant PJ-1430 seedlings of sorghum. Evaluation of the expression of the innate genes after infection with M. phaseolina established that time and genotype had significant effect on the expression of SbChit and SbSTS1. The two genes were also differentially expressed. In the 0–168 HPI analysis window, most of the significant changes in gene expression occurred between 48–96 HPI and 24–96 HPI in chitinase and stilbene synthase, respectively.
The expression of sorghum chitinase gene, SbChit was evaluated in PJ-1430 and SU-1080, SbChit was expressed in both resistant and the susceptible cultivars. The average fold change in the expression of this gene is between 48 and 96 HPI. The highest expression of SbChit was noted in PJ-1430, whereas the least was in SU-1080 (). The fold change in the expression of this gene was 4.79 and 1.66 in PJ-1430 leaf and root sample, whereas the least was 2.97 and 1.07 in SU-1080 leaf and root samples. The expression of chitinase in SU-1080 was induced at 0 and 24 h (0.53 and 0.57 fold, respectively, in leaf and root samples). However, the expression of this gene decreased after 96 h. Thus, upon fungal inoculation, chitinase was induced within 0–72 h in SU-1080, whereas in PJ-1430, the expression of chitinase at 0 and 24 h decreased to 3.14 to 2, respectively, in leaf samples. After that it peaks transiently up to 4.79 at 72 h and return to 2.5 at 96 HPI. There is a down-regulation of chitinase gene in SU-1080 at 168 h because of mRNA degradation or decrease in the expression of mRNA. Thus, although in susceptible cultivars chitinase gene was induced earlier in root samples within 24 HPI, whereas in resistant genotype it was induced only at 48 HPI. The induction level of this gene is higher in resistant genotype in terms of fold change. The results of infection study suggested that chitinase gene was regulated differently in two sorghum cultivars.
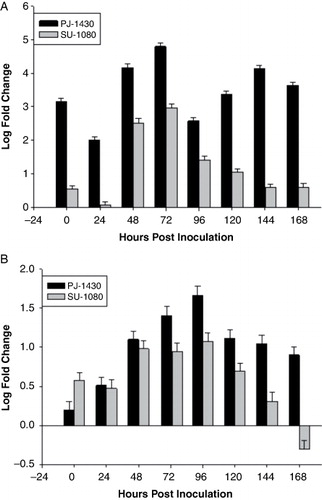
The expression of SbSTS1 was evaluated in PJ-1430 and SU-1080 seedlings of sorghum. The average change in the expression of this gene is between 24 and 48 HPI. The highest expression of this gene was noted in the resistant genotype than the susceptible one (). The fold change in the expression of this gene was 5.64 and 4.01 in resistant genotype PJ-1430, whereas the least in SU-1080 2.62 and 2.29 in SU-1080 in the leaf and root samples, respectively. Expression of this gene was induced at 0 HPI (1.01 and 1) in susceptible genotype and (3.39 and 1.77) in resistant one in leaf and root, respectively. However, the expression of this gene decreased after 48 HPI. Upon fungal inoculation, the expression level of susceptible variety increased upto 2.62 and 2.29 in leaf and root samples after that it decreases transiently and reaches below ground level in leaf sample but in case of resistant genotype fold change increases to 1 to 4 fold in root samples at 48 HPI. The activity of this gene was significantly up-regulated in the charcoal root rot resistant genotype. Previous reports contented that SbSTS1 was only expressed after infection.
4. Discussion
A time-course expression of antifungal genes in 15-day-old plants of sorghum selected for this study showed that their expression levels were significantly higher in resistant cultivars. In past too, defense response studies using biochemical parameters have been carried out in 15-day-old plants of several species, viz. cluster bean, cotton, lady's finger, black gram (Sheikh & Ghaffar Citation1979), cowpea (Ahmed et al. Citation2012), moth bean (Garg & Gupta Citation2010) using mycelial suspension method. The transcripts of chitinase and stilbene synthase exhibited a rapid up-regulation as early as 24 HPI and reached maximum, then down-regulations were noted at later stages. Similar defense response patterns were observed by Caldo et al. (Citation2004) for compatible and incompatible interactions involving Mla1-like genes in barley in response to powdery mildew; transcripts build-up of defense-related genes were same in both resistant and susceptible varieties except that during later stages of infection expression levels get decreased. In our experiment, we used actin gene as an internal control; this gene has been used in Brassica rapa infected with club root disease and its expression has not been affected by fungal expression (Schuller & Muller Citation2006). The infected plants consistently showed higher gene expression level of chitinase in comparison to the control plants (including mock-inoculation controls). Hence, the chitinase activity can certainly be attributed due to induction by pathogen. However, in our study, we obtained higher chitinase gene levels in leaf rather than in roots. Similar results were obtained by Yeoh et al. (Citation2012) in oil palm.
Conversely, maximum expression level of chitinase and stilbene synthase was observed at 72 and 24 HPI, respectively. Moreover, these genes were expressed at higher levels in resistant variety. Expression patterns of both the genes suggest that they play a vital role in S. bicolor and M. phaseolina pathosystem. Plants produce an array of phenolic compounds and PR proteins that exhibit differential defense responses against pathogen (Niderman et al. Citation1995). Highest expression level of stilbene synthase even at 24 HPI suggests that up-regulation of this defense-related gene is among the first defense response affected by pathogen infection in the resistant variety.
Even in susceptible variety, up-regulation of these genes tends to occur at later stages but at lower levels. These delays in up-regulation of defense responses have also been observed in susceptible varieties after pathogen attack (Maleck et al. Citation2000; Martinez de Ilarduya et al. Citation2003). Some studies have demonstrated that infection of fungal pathogen in resistance and susceptible varieties was similar in initial hours after pathogen inoculation. Then, resistance expression resulted in the failure of fungal pathogen to become established in resistant varieties in later days (Ma et al. Citation2009). Therefore, expression of defense-related genes would be expected at variable time period after pathogen attack. The very rapid expression of stilbene synthase gene causes hindrance in the earlier stages of disease cycle.
Expression level of both the genes in resistant variety was 60% more than susceptible one (). This suggests greater induction of chitinase gene after pathogen attack. Expression of stilbene synthase was 50% higher in resistant variety at 24 HPI and 48 HPI in leaf and root samples, respectively, as compared to the susceptible one (). The reports also postulated that higher expression of stilbene synthase is related to disease tolerance (Lo et al. Citation2002). The expression of this gene was first noted in sorghum seedlings infected with Colletotrichum sublineolum (Lo et al. Citation2002). A fast accumulation of stilbene synthase-specific mRNA followed by a decrease was also obtained in transgenic tobacco (Hain et al. Citation1993) and transgenic rice (Stark-Lorenzen et al. Citation1997). Marked increase in barley occurred after 48 h, but was not observed in transgenic tobacco and rice. The repeated increase was only slight and the stilbene synthase mRNA synthesis disappeared completely 72 h after inoculation. Stilbene synthesis was induced in grapevine by inoculation with the pathogens Botrytis cinerea or Plasmopara viticola (Blaich & Bachmann Citation1980). The level of resistance to P. viticola was positively correlated with the capacity of Vitis spp. to synthesize stilbene (Dercks & Creasy Citation1989). The effectiveness of stilbene synthase genes in increasing resistance in transgenic tomatoes was shown by a 65% reduction in disease incidence following inoculation with the pathogen Phytophthora infestans (Thomzik et al. Citation1997). Results from transgenic rice plants indicated that the stilbene synthase gene enhanced plant resistance against the rice blast fungal pathogen Pyricularia oryzae (Stark-Lorenzen et al. Citation1997).
The different trend in the expression of SbSTS1 observed in PJ-1430 and SU-1080 could be clarified by correlating its activity and the state of the leaf and root samples in which it was up-regulated. It was noted that SbSTS1 was up-regulated in all root samples that exhibited disease symptoms. Such symptoms were distinctly detectable in the histochemical analysis of the plant sample which confirmed the presence of fungus in the form of mycelia and sclerotia (not shown). Statistical analysis of SbSTS1 showed that most of the up-regulations occurred between 24 and 48 HPI when the seedlings were showing clear symptoms. In addition, roots of SU-1080 showed signs of infection as early as 48 HPI, which corresponded to a fold change of 2.2. It should therefore be concluded that up-regulation of SbSTS1 in sorghum is associated with symptoms development. Asymptomatic stages of infection are associated with low activity of this gene. A previous report indicated that SbSTS1 was preferentially up-regulated in sorghum lines that showed tolerance to anthracnose (Yu et al. Citation2005). SbSTS1 was expressed in charcoal root rot tolerant PJ-1430, but its activity was also higher in susceptible SU-1080. It is also relevant to point out that SbSTS1 was considered to be expressed exclusively upon C. sublineolum infection (Yu et al. Citation2005). This study, however, established that SbSTS1 was also expressed in non-infected leaves. This critical observation could be interpreted from results obtained from other plant species where it was found that the expression of SbSTS1 gene was induced by a variety of abiotic and biotic stresses, such as elicitor treatment, pathogen inoculation, wounding, UV irradiation, and post-harvest wilting procedures (Preisig-Müller et al. Citation1999; Versari et al. Citation2001). Constitutive expression of CHS8-related stilbene synthase gene was described in young seedlings of grapes (Sparvoli et al. Citation1994). It was concluded that this presumably represents a pre-existing defense mechanism (Sparvoli et al. Citation1994). It was demonstrated in this study that constitutive expression of SbSTS1 occurs in sorghum. These results prove that stilbene synthase can be induced in heterologous plant systems to broadly increase resistance to fungal pathogens. Hence, the signal pathway of these defense-related genes is apparently conserved across a large group of plants. Because phytoalexins are toxic against a variety of pathogenic fungi, it is reasonable to assume that the described system would work in many host–pathogen interactions, such as papaya and its fungal pathogens. Stilbene synthase genes have been used to introduce novel phytoalexins into plants, resulting in enhanced disease resistance (Hain et al. Citation1993; Fischer & Hain Citation1994). However, the level of disease resistance of plants due to fungi toxic substances such as phytoalexins is believed to depend on the ability to synthesize high amounts of the compound within a short period of time after infection (Hain et al. Citation1993; Fischer & Hain Citation1994). Another explanation could be a negative influence of the depletion of malonyl CoA and 4-coumaroyl CoA needed for other plant-owned defense mechanisms such as natural phenolic compound synthesis. So far, our observations have led to the conclusion that, in terms of engineering disease-resistant plants, enhancement of the natural SbSTS1 expression pattern is more favorable than constitutive SbSTS1 expression (Hain et al. Citation1993).
5. Conclusion
Current study on pathogen facilitated induced defense response in sorghum provides us with complete and physiological interpretation of defense mechanism of a host against a pathogen. As in response to M. phaseolina attack, production of antifungal genes was induced in sorghum plant. Based on this information, we should make genetically modified varieties of sorghum capable of constitutive production of these defense-related genes in plants to reduce the risk of damages and losses in plants.
Acknowledgments
The financial support provided by Department of Science and Technology (DST), New Delhi for the project, ‘Banasthali Centre for Education and Research in Basic Sciences’ under the CURIE (Consolidation of University Research for Innovation and Excellence in Women Universities) program is gratefully acknowledged.
References
- Abawi GS, Pastor-Corrales MA. 1990. Root rots of beans in Latin America and Africa: diagnosis, research methodologies, and 129 management strategies. Cali, Colombia: CIAT; p. 114.
- Ahmed O, Balogun OS, Busayo TS. 2012. Screening of cowpea genotypes for resistance to Macrophomina phaseolina infection using two methods of inoculation. Asian J Plant Pathol. 6:13–18.10.3923/ajppaj.2012.13.18
- Bashir MA, Malik BA. 1988. Disease of major pulse crops in Pakistan. Trop Pest Manage. 34:309–314.10.1080/09670878809371262
- Blaich R, Bachmann O. 1980. Synthesis of resveratrol in Vitis: induction and cytological observation. Vitis. 38:65–68.
- Borad V, Sriram S. 2008. Pathogenesis-related proteins for the plant protection. Asian J Exp Sci. 3:189–196.
- Caldo R, Nettleton D, Wise P. 2004. Interaction dependent gene expression in Mla-specified response to barley powdery mildew. Plant Cell. 16:2514–2528.10.1105/tpc.104.023382
- Darnetty, LJF, Muthukrishnan S, Swegle M, Vigers A, Selitrennikoff CP. 1993. Variability in antifungal proteins in the grains of maize, sorghum and wheat. Physiol Plant. 88:339–349.10.1111/j.1399-3054.1993.tb05508.x
- Das IK, Indira S, Annapurna A, Prabhakar, Seetharam N. 2008. Biocontrol of charcoal rot in sorghum by fluorescent Pseudomonads associated with the rhizosphere. Crop Prot. 27:1407–1414.10.1016/j.cropro.2008.07.001
- Delaney TP, Uknes S, Vernooij B, Friedrich L, Weymann K, Negrotto D, Gaffney T, Gut-Rella M, Kessmann H, Ward E, Ryals J. 1994. A central role of salicylic acid in plant disease resistance. Science. 266:1247–1250.10.1126/science.266.5188.1247
- de Leon IP, Montesano M. 2013. Activation of defense mechanisms against pathogens in mosses and flowering plants. Int J Mol Sci. 14:3178–3200.10.3390/ijms14023178
- Dercks W, Creasy LL. 1989. The significance of stilbene phytoalexins in the Plasmopara viticola grapevine interaction. Physiol Mol Plant Pathol. 34:189–202.10.1016/0885-5765(89)90043-X
- Ebrahim S, Usha K, Singh B. 2011. Pathogenesis related (PR) proteins in plant defense mechanism. In: Mendez-Vilas A, editor. Science against microbial pathogens: communicating current research and technological advances, 3rd ed., Vol. 2. Badajoz, Spain: Formatex Research Centre Publisher; p. 1043–1054.
- Fischer R, Hain R. 1994. Plant disease resistance resulting from the expression of foreign phytoalexins. Curr Opin Biotechnol. 5:125–130.10.1016/S0958-1669(05)80024-2
- Gaige AR, Ayella A, Shuai B. 2010. Methyl jasmonate and ethylene induce partial resistance in Medicago truncatula against the charcoal rot pathogen Macrophomina phaseolina. Physiol Mol Plant Pathol. 74:412–418.10.1016/j.pmpp.2010.07.001
- Garg N, Gupta H. 2010. Isolation and purification of fungal pathogen (Macrophomina phaseolina) induced chitinase from moth beans (Phaseolus aconitifolius). J Pharm Bioallied Sci. 2:38–43.10.4103/0975-7406.62708
- Gupta P, Ravi I, Sharma V. 2012. Induction of β-1, 3-glucanase and chitinase activity in the defense response of Eruca sativa plants against the fungal pathogen Alternaria brassicicola. J Plant Interact. 8:155–161.
- Hain R, Bieseler B, Kindl H, Schroder G, Stocker R. 1990. Expression of a stilbene synthase gene in Nicotiana tabacum results in the synthesis of the phytoalexin resveratrol. Plant Mol Biol. 15:325–335.10.1007/BF00036918
- Hain R, Reif HJ, Krause E, Langebartels R, Kindl H, Vorman B, Wiese W, Schmelzer E, Schreier P, Ströcker R, Stenzel K. 1993. Disease resistance results from foreign phytoalexin expression in a novel plant. Nature. 361:153–156.10.1038/361153a0
- Huang CJ, Wang TK, Chung SC, Chen CY. 2005. Identification of an antifungal chitinase from a Trichoderma lignorum for the control of potential biocontrol agent Bacillus cereus. J Biochem Mol Biol. 38:82–88.10.5483/BMBRep.2005.38.1.082
- Lo C, Coolbaugh RC, Nicholson RL. 2002. Molecular characterization and in silico expression analysis of a chalcone synthase gene family in sorghum. Physiol Mol Plant Pathol. 61:179–188.10.1006/pmpp.2002.0428
- Ma J, Hill CB, Hartman GL. 2010. Production of Macrophomina phaseolina conidia by multiple soyabean isolates in culture. Plant Dis. 94:1088–1092.
- Ma X, Hua L, Sivasithamparam K, Barbetti M. 2009. Infection processes and involvement of defense-related genes in the expression of resistance in cultivars of subterranean clover (Trifolium subterraneum) to Phytophthora clandestine. Genet Resist. 100:551–559.
- Maleck L, Levine A, Eulgem T, Morgan A, Schmid J, Lawton K. 2000. The transcriptome of Arabidopsis thaliana during systemic acquired resistance. Nat Genet. 26:403–410.10.1038/82521
- Martinez de Ilarduya O, Xie Q, Kaloshian I. 2003. Aphid induced defense responses in Mi-1 mediated compatible and incompatible tomato interactions. Mol Plant Microbe Interact. 16:699–708.10.1094/MPMI.2003.16.8.699
- Muchero W, Ehlers JD, Close TJ, Roberts PA. 2011. Genic SNP markers and legume synteny reveal candidate genes underlying QTL for Macrophomina phaseolina resistance and maturity in cowpea. BMC Genomics. 12:8.
- Mughogho LK, Pande S. 1984. Charcoal rot of sorghum. In: Mughogho LK, editor. Sorghum root and stalk rots, a critical review: proceedings of the consultative group discussion on research needs and strategies for control of sorghum root and stalk diseases. Patancheru, India: ICRISAT; p. 11–24.
- Niderman T, Genetet I, Bruyere T, Gees R, Stintzi A, Legrand M. 1995. Pathogenesis related PR-1 proteins are antifungal. Isolation and characterization of three 14-kilodalton proteins of tomato and of a basic PR-1 of tobacco with inhibitory activity against Phytophthora infestans. Plant Physiol. 108:17–27.10.1104/pp.108.1.17
- Oldach KH, Morgenstern A, Rother S, Girgi M, O'Kennedy MM, Lorz H. 2001. Efficient in vitro plant regeneration from immature zygotic embryos of pearl millet (Pennisetum glaucum (L.) and Sorghum bicolor (L.) Moench. Plant Cell Rep. 20:416–421.10.1007/s002990100335
- Preisig-Müller R, Schwekendiek A, Brehm I, Reif HJ, Kindl H. 1999. Characterization of a pine multi gene family containing elicitor-responsive stilbene synthase genes. Plant Mol Biol. 39:221–223.10.1023/A:1006163030646
- Reuveni R, Nachmias A, Krikun J. 1983. The role of seed borne inoculum on the development of Macrophomina phaseolina on melon. Plant Dis. 67:280–281.10.1094/PD-67-280
- Schroder J, Schroder G. 1990. Stilbene and chalcone synthase related enzymes with key functions in plant-specific pathways. Z Naturforsch. 45:1–8.
- Schuller A, Muller JL. 2006. A family of auxin conjugate hydrolases from Brassica rapa: characterization and expression during clubroot disease. New Phytologist. 171:145–158.10.1111/j.1469-8137.2006.01727.x
- Sheikh AH, Ghaffar A. 1979. Relation of sclerotial inoculum density and soil moisture to infection of field crops by Macrophomina phaseolina. Pak J Bot. 11:185–189.
- Sparvoli F, Martin C, Scienza A, Gavazzi G, Tonelli C. 1994. Cloning and molecular analysis of structural genes involved in flavonoid and stilbene biosynthesis in in grape. Plant Mol Biol. 24:743–755.10.1007/BF00029856
- Stark-Lorenzen P, Nelke B, Hanler G, Muhlbach HP, Thomzik JE. 1997. Transfer of a stilbene synthase gene to rice (Oryza sativa L.) Plant Cell Rep. 16: 668–673.10.1007/s002990050299
- Thomzik JE, Stenzel K, Stoecker R, Schreier PH, Ham R, Stahl DJ. 1997. Synthesis of grapevine phytoalexin in transgenic tomatoes (Lycopersicon esculentum Mill.) conditions resistance against Phytophthora infestans. Physiol Mol Plant Pathol. 51:265–278.10.1006/pmpp.1997.0123
- Versari A, Parpinello GP, Tornielli GB, Ferrarini R, Giulive C. 2001. Stilbene compounds and stilbene synthase expression during ripening, wilting, UV treatment in grape cv. Corina. J Agric Food Chem. 49:5531–5536.10.1021/jf010672o
- Wu C, Bradford KJ. 2003. Class I chitinase and â-1,3-glucanase are differentially regulated by wounding, methyl jasmonate, ethylene and gibberellin in tomato seeds and leaves. Plant Physiol. 133:263–273.10.1104/pp.103.024687
- Wyllie TD. 1998. Charcoal rot. In: Sinclair JB, Backman PA, editors. Compendium of soybean diseases, 3rd ed. St. Paul, MN: APS Press; p. 114–118.
- Xu Y, Chang PL, Liu D, Narasimhan ML, Raghothama KG, Hasegawa PM, Bressan RA. 1994. Plant defense genes are synergistically induced by ethylene and methyl jasmonate. Plant Cell. 6:1077–1085.
- Yeoh KA, Othman A, Meon S, Abdullah F, Ho CL. 2012. Sequence analysis and gene expression of putative oil palm chitinase and chitinase like proteins in response to Ganoderma boninense or Trichoderma harzianum. Mol Biol Rep. 40:147–158.10.1007/s11033-012-2043-8
- Yu CKY, Nicholson RL, Springob K, Schimdt J, Chu IK, Lo C. 2005. Stilbene synthase gene (SbSTS1) is involved in host and non-host defense responses in sorghum. Plant Physiol. 138:393–401.
- Yu CKY, Lam CNW, Springob K, Schimdt J, Chu IK, Wing KY, Lo C. 2006. Constitutive accumulation of cis-piceid in transgenic arabidopsis overexpression a sorghum stilbene synthase gene. Plant Cell Physiol. 47:1017–1021.
- Zveibil A, Mor N, Gnayem N, Freeman S. 2012. Survival, host-pathogen interaction, and management of Macrophomina phaseolina on strawberry in Israel. Plant Dis. 96:265–272.